Bioenergetics 1 PDF 2024
Document Details
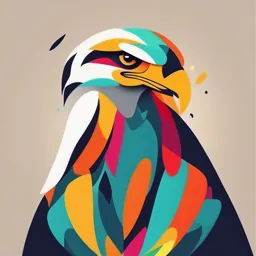
Uploaded by DecisiveMorningGlory
Curtin University
2024
Ricardo L. Mancera
Tags
Summary
These are lecture notes for a biochemistry course at Curtin University, covering the topic of bioenergetics. The notes detail the concepts of chemical thermodynamics, such as enthalpy and entropy, and their relations to biochemical reactions. The document also lists characteristics of life and how organisms use matter and energy.
Full Transcript
BCCB2000 Foundations of Biochemistry Bioenergetics 1 Ricardo L. Mancera WARNING This material has been reproduced and communicated to you by or on behalf of Curtin University in accordance with section 113P of the Copyright Act 1968 (the Act) The material in this communication may be subject to copy...
BCCB2000 Foundations of Biochemistry Bioenergetics 1 Ricardo L. Mancera WARNING This material has been reproduced and communicated to you by or on behalf of Curtin University in accordance with section 113P of the Copyright Act 1968 (the Act) The material in this communication may be subject to copyright under the Act. Any further reproduction or communication of this material by you may be the subject of copyright protection under the Act. Do not remove this notice. 1 Learning objectives When you complete this lecture you will be able to Recapitulate some key principles of chemical thermodynamics Understand the need of organisms for sources of energy and carbon Describe the concepts of energy, enthalpy, entropy, Gibbs free energy, and activation energy Use the biochemical knowledge learned to answer questions and solve problems Characteristics of life Complicated structure and highly organised Ability to extract, manage and use matter Ability to extract, transform, manage and use energy Ability to synthesise new material: Replacement synthesis Replacing damaged or used components of cells Growth: Self-replication synthesis Cell replication and DNA synthesis Greater number of cells Growth: Expanding synthesis Larger cells Ability to self-organise and self-assemble 2 Life needs matter and energy Matter – carbon (C) and other elements (H, O, N, P, S) Organic compounds and other molecules that contain carbon and other atoms Energy – on-going supply of chemical energy For example: calcium (Ca), sodium (Na), potassium (K), magnesium (Mg) and iron (Fe) Needed for all the changes that occur in life The flow of energy and matter in a living system is controlled by enzymes The flow of energy and matter (carbon) 3 The flow of matter Matter used by living organisms is converted to other types of matter: The atoms (such as carbon) that comprise the matter remain the same but are rearranged into other molecules This matter is used for self-replication, self-assembly, synthesis etc. in all life forms Matter can be followed in the chemical reactions of life (metabolism) Radioactively labelled atoms are used such as 14C, 15N, 3H (tritium) Matter is not transformed to energy Life does not and cannot use nuclear fusion or fision! What is energy? Energy is what needs to be transferred between objects to cause specific changes (i.e. do work) or change temperature (i.e. exchange heat) Energy has many forms: Stored forms: chemical, nuclear, elastic and gravitational Moving forms: thermal, mechanical, electrical, electromagnetic (radiant) and sound Energy is transferred and converted from one form to another, but it is not created or destroyed Energy remains constant in the Universe (first law of thermodynamics) All natural processes proceed in a direction towards a minimum potential energy Atoms and molecules respond to external forces applied on them 4 Energy values in perspective 1 J = energy used when raising a 100 g apple 1 m against the force of gravity 49 J = dropping a 5 kg bag of potatoes from about a metre height 98 kJ = energy used to lift a tonne of bricks (1000 kg) by 10 m 222 kJ = energy used to lift a 70 kg person to the top of the Eiffel tower (324 m) Approximate calculated values using the following definitions and constants: Force (Newtons, N) = mass (kg) x acceleration (m·s-2) On Earth, acceleration due to gravity = 9.81 m·s-2 Energy (Joules, J) = Force x distance = mass (kg) x acceleration (m·s-2) x distance (m) 1.0 J = Force of 1.0 N applied over a distance of 1.0 m (units = kg·m2·s-2) Energy values in perspective 1 calorie = 4.184 J 1 Calorie = 1000 calories = 1 kcal = 4.184 kJ The large ‘C’ calorie is often used in nutrition as a measure of the energy content of food 5 How is energy used by organisms? Change of location or orientation (mechanical work) Change of concentration (osmotic and electrical work) Muscle contraction, opening and closing of a flower, movement of bacteria Movement of nutrients, drugs, minerals, etc. across a membrane against their concentration and/or electrostatic potential Making and breaking chemical bonds Synthesis and degradation of compounds Continual maintenance and repair Flow of energy: thermodynamics The flow, transfer, interconversion, transformation or change brought about by energy is described by thermodynamics Transfers of energy can be tracked by defining a system and its surroundings A system describes a part of the world of interest and consists of matter capable of undergoing a change. A complete definition includes: The matter that it contains, the pressure, volume and temperature For example, the system can be a glucose molecule, a cell, or any defined space with a real or imaginary boundary The surroundings are anything in contact with the system that can influence its state Universe = system + surroundings 6 Flow of energy: thermodynamics Systems can interact with their surroundings via a flow of heat (q) or work (w) Heat is the transfer of energy that changes the motion of particles in the surroundings in a chaotic manner Work is the transfer of energy that changes the motion of particles in the surroundings in a uniform matter Universe Universe = system + surroundings Surroundings System Energy* in Total internal stored energy U Energy* out *Transfers of energy occur through heat (q) or work (w). The direction of energy transfer is defined as: Transfer from system to surroundings = –q or –w Transfer from surroundings to system = +q or +w Describing systems Three of the most important variables used to describe a system are Pressure Volume Temperature In biological systems these variables are essentially constant The change in these variables is slow in comparison with the rapid change in reactions Volume changes can occur in living organisms but most reactions occur in solution so constant volume can be assumed for most reactions 7 Internal, potential and kinetic/motional energy Internal (total) energy (U) Comprised of potential (E) and kinetic energy (K): U = E + K Described by the enthalpy (plus the product of pressure and volume): H = U + PV Potential energy (E) Energy due to location and position The potential energy of a molecule reflects its conformation and its location with respect to external forces (e.g. other molecules) Electronic energy levels in ionic, covalent and other bonds in molecules and ions Kinetic/motional energy (K) Energy involved in translation (movement), rotation and vibration of molecules Determined by the mass and velocity of the molecules Directly related to the temperature Organisms and energy An organism Is never at equilibrium with its surroundings Constantly transfers matter and energy with its surroundings Is highly ordered Always obey the first and second laws of thermodynamics 8 Chemical reactions and energy Thousands of different types of chemical reactions occur in organisms Need to know if a reaction can occur spontaneously or not (thermodynamics) A spontaneous chemical reaction is one that does not require any additional input of energy Need to also know how fast (or slow) these reactions occur (kinetics) Speed of reactions is important: if reactions occur too slowly an organism will not survive Chemical reactions and energy A reactive molecule is one with relatively more energy than another A reactive molecule is relatively unstable A higher energy molecule is relatively more reactive and unstable than a lower energy molecule A stable molecule is one that can last long enough to be used in another reaction In a chemical reaction: Bonds are broken and formed Potential energy in bonds used to change kinetic energy of electron rearrangement Making bonds releases energy Breaking bonds requires energy Electrons tend to redistribute to an atom or molecule that has a lower energy arrangement Energy to make or break bonds is referred to as the ‘bond energy’ (E) 9 Enthalpy and its changes (ΔH) The total energy of a system is equivalent to its enthalpy (H). Enthalpy essentially describes the changes (ΔH) involved in various physico-chemical processes: Formation and breaking of covalent bonds Formation and breaking of non-covalent bonds Hydrogen bonds, ionic interactions and van der Waals interactions Changes in the motion of atoms due to changes in temperature Changes in volume due to changes in pressure or density (usually small) Enthalpy is equivalent to the energy transferred by heat in chemical and biological systems If pressure and temperature are constant Enthalpy and its changes Release energy from the system to the surroundings (–ΔH) Energy required to break bonds is lower than energy released from forming bonds Enthalpy products < enthalpy reactants Absorb energy from the surroundings into the system (+ΔH) Energy required to break bonds is greater than energy released from forming bonds Enthalpy products > enthalpy reactants 10 Entropy and its changes (ΔS) Entropy describes the tendency for Motional energy to disperse or spread out Matter to disperse or spread out Energy and matter go from being localised to more spread out, at a given temperature It is convenient to describe entropy separately as matter or energy dispersal but they are different forms of the same thing Dispersal of matter and energy is often described as an increase in disorder Entropy and its changes Entropy in action: expansion of gas molecules across two chambers and dissolution and diffusion of a solid in solution The gas/solute molecules become more spread out: dispersal of matter The motional energy (including kinetic energy) of the molecules becomes more spread out: dispersal of energy 11 Entropy and its changes Mixing of two gases also increases entropy Entropy should be ultimately thought of as a direct measure of the number of ways in which molecules and their energy can be distributed Entropy and its changes Entropy gas > entropy of liquid > entropy of solid Entropy of solution (a mixture) > entropy of pure solvent or solute Entropy increases (ΔS) in a system if a chemical reaction results in an increase in the number of molecules Similarly, entropy decreases if a chemical reaction results in a decrease in the number of molecules That is, ‘motional energy’ is more constrained in reactions where there are fewer products compared to reactants 12 Entropy and its changes Entropy can be used to describe the motional energy in molecules and ions (vibrational, translational, rotational and electronic) Closed systems at absolute zero temperature (0 K) have an entropy of zero (third law of thermodynamics) Restricted motional energy leads to decreased entropy Increased motional energy leads to increased entropy Entropy does not prevent ordered, complex structures from being formed The entropy of the Universe always increases (second law of thermodynamics) Enthalpy and entropy Most chemical reactions result in Lower energy (enthalpy) A more stable state Greatest increase in dispersal of energy/matter (disorder) (entropy) Chemical reactions and molecules result from the effects of both enthalpy and entropy ΔH and ΔS only consider initial and final states of a molecule or chemical reaction They are so-called state functions The path taken going from the initial to the final state is not relevant For example, the energy released during the oxidation of a molecule, e.g. glucose, is the same inside a living cell or in a laboratory experiment 13 Entropy in biological systems Entropy of living systems is low Complex molecules Cellular and organism organisation The low entropy of living systems is thermodynamically allowed: Dispersal of matter Breakdown of large biological molecules Rearrangement of atoms Dispersal of energy Mainly from ‘loss’ of heat energy from biochemical reactions to the surroundings Organisms and energy revisited The constant exchange of matter and energy with the surroundings of an organism has two consequences: Increases entropy of surroundings and hence the ‘Universe’ Allows the organism to create order within themselves (low entropy of the ‘system’) Surroundings Energy and matter in Organism (the ‘system’) Energy and matter out 14 Entropy and spontaneous chemical reactions A reaction is spontaneous when it can proceed from reactants to products without any additional input of energy The entropy of the Universe needs to always increase for a spontaneous reaction to occur, i.e. ΔSuniverse > 0 (positive +ΔS): ΔSuniverse = ΔSsurroundings + ΔSsystem Gibbs free energy (ΔG) Gibbs free energy (‘free energy’) or ΔG is a useful way to determine if a reaction will be spontaneous ΔG predicts the spontaneity of a chemical reaction only from the properties of the system itself: Accounts for both enthalpy and entropy changes in the system and surroundings ΔG describes how far a reaction is from equilibrium ΔG depends upon the concentration of reactants and products Often biochemical reactions involve concentrations of reactants that make the reaction spontaneous! 15 Gibbs free energy (ΔG) Temperature (K) ΔG = ΔH –TΔS Change in free energy Change in enthalpy Change in entropy Energy available to do work and make changes in the system* Heat released/absorbed by the system* Energy still residing in the system* Energy contained in bonds of the molecules in the system Energy contained in the motional energy of components (e.g. products) in the system * ‘System’ is the chemical reaction or process that is being considered Gibbs free energy (ΔG) Negative free energy change (–ΔG) means that the reaction is spontaneous (exergonic) Positive free energy change (+ΔG) means that the reaction is not spontaneous (endergonic) Exergonic reactions can be used to ‘drive’ endergonic reactions if coupled 16 Predicting spontaneous reactions ΔG = ΔH –TΔS Understanding Gibbs free energies If ATP is hydrolysed at 25°C under standard conditions where ΔG’º = -30.5 kJ mol-1, and it is not coupled to any other reaction. Would this mean that the entire free energy change manifests as heat? The enthalpy (ΔH) part would be released as heat but not the entropy (TΔS) part ΔG = ΔH –TΔS About +14.6 kJ mol-1 remains in the ‘system’ and is unavailable for work, contributing to increased motional energy of the products Released as heat 17 Standard Gibbs free energy ΔGº is the energy available to perform work (i.e. make changes) at standard conditions of 1 M concentration for each reactant, 1 atmosphere pressure and a temperature of 25°C ΔG’º is the energy available to perform work (i.e. make changes) at biochemical standard conditions of 1 M concentration for each reactant, 1 atmosphere pressure, a temperature of 25°C and a pH of 7.0 A standard value is needed for thermodynamic calculations: To compare the free energies of different chemical reactions To allow free energies from different chemical reactions to be added Standard and actual Gibbs free energy The standard free energy (ΔG’º) value is a constant It is a characteristic of a particular chemical reaction The actual free energy (ΔG) value is the free energy change resulting from the actual concentrations of reactants in the cell (or in vitro) at a particular moment The actual free energy (ΔG) varies with reaction progress as the concentration of reactant(s) and product(s) change 18 Gibbs free energy and equilibrium ΔG describes how far a chemical reaction is from equilibrium It is another way of describing equilibrium For a reaction under standard conditions: aA + bB = cC + dD has an equilibrium constant Keq = [C]c[D]d / [A]a[B]b ΔGº = - RT ln Keq at 25°C, 1 atmosphere, and [1 M] for all reactants ΔG'º = - RT ln K’eq at 25°C, 1 atmosphere, [1 M] for all reactants except H+, which is at pH 7. R is the gas constant (8.315 JK-1mol-1), T is temperature (K), ln = natural logarithm (base e) Gibbs free energy – non-standard conditions Need to use actual concentrations of products and reactants, not standard concentrations For a reaction under non-standard conditions: aA + bB = cC + dD c d ΔG = ΔG'º + RT ln [C] a[D] b [A] [B] R is the gas constant (8.315 JK-1mol-1), T is temperature (K), ln = natural logarithm (base e) 19 Equilibrium, free energy and entropy Equilibrium depends upon the concentration of products and reactants Equilibrium does not necessarily mean that the reactants and products are in equal concentration At equilibrium, rate of forward reaction = rate of reverse reaction Reversible reactions tend towards equilibrium Equilibrium is favoured by entropy Entropy of ‘mixing’ Free energy reaches zero at equilibrium ΔG’º and the equilibrium constant K’eq Relationship between K’eq, ΔG’º and the direction of chemical reactions ΔG'º = - RT ln K’eq K’eq G’º > 1.0 -ve 1.0 0 < 1.0 +ve Reaction Proceeds in the direction of products Is at equilibrium Proceeds in the direction of reactants ΔG’º measures the tendency to move towards equilibrium 20 Activation energy Barrier in free energy that any chemical reaction needs to overcome before it can proceed Spontaneous (exergonic) reactions nearly always have an activation energy too! Activation energy Where does the energy come from to surmount the activation energy barrier? Energy of the atoms/molecules themselves There is a distribution of energy at any given temperature, so some molecules have higher (and others lower) energies Energy from surroundings Heat transfer 21 Activation energy Not the same as the enthalpy or entropy for a reaction But can have enthalpy and entropy components Keeps molecules relatively stable Minutes, days or even years! A ‘check’ on the spontaneity of a chemical reaction Depends upon the path taken from initial to final states The reaction pathway can be very complex but this is ‘disguised’ by exhibiting only one (the highest) activation energy Decreased by enzymes and other catalysts A different reaction pathway is enabled to take place that has a lower activation energy Can we now define life? System that evades the decay to thermodynamical equilibrium by homeostatically maintaining “negative entropy” in an open system (Erwin Schrödinger) Life creates “order from disorder” The entropy of the Universe (the ultimate closed system) always increases Second law of thermodynamics is maintained! “Self-sustaining chemical system capable of undergoing Darwinian evolution” (Gerald Joyce) 22 Further reading Chemical Thermodynamics, in Chemistry: The Central Science (Brown, LeMay, Busten, Murphy & Woodward): https://chem.libretexts.org/Bookshelves/General_Chemistry/Map%3A_Chemistry__The_Central_Science_(Brown_et_al.)/19%3A_Chemical_Thermodynamics Thermodynamics for chemists (Khan Academy): https://www.khanacademy.org/science/chemistry/thermodynamics-chemistry https://www.khanacademy.org/science/chemistry/thermodynamics-chemistry/gibbsfree-energy/v/gibbs-free-energy-and-spontaneity Prof Dave’s explanation of the Laws of thermodynamics, entropy and Gibbs free energy: https://www.youtube.com/watch?v=8N1BxHgsoOw What is entropy? By Jeff Phillips. A more detailed explanation of entropy: https://www.youtube.com/watch?v=YM-uykVfq_E 23