Antibacterial Drugs - Foundations and Frameworks PDF
Document Details
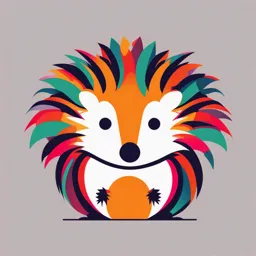
Uploaded by ReverentHorse
Tags
Summary
This document provides an overview of antibacterial drugs, covering their mechanisms of action, uses, and the difference between antibiotics and antibacterials. It discusses the history and impact of antibacterial drugs on public health. The document includes a detailed discussion of cell wall, DNA/RNA, and protein synthesis inhibitors.
Full Transcript
exchange.scholarrx.com /brick/antibacterial-drugs-foundations-and-frameworks Antibacterial Drugs: Foundations and Frameworks 13-17 minutes It’s us versus them, and we are profoundly outnumbered. The earth has an estimated 5 nonillion (that’s 30 zeros, folks) bacteria, and well before humans took the...
exchange.scholarrx.com /brick/antibacterial-drugs-foundations-and-frameworks Antibacterial Drugs: Foundations and Frameworks 13-17 minutes It’s us versus them, and we are profoundly outnumbered. The earth has an estimated 5 nonillion (that’s 30 zeros, folks) bacteria, and well before humans took their first steps, a battle has raged on between bacteria and eukaryotic life. While many bacteria took their place as our closest allies, helping many organisms digest food, acquire nutrients, and protect them from their environments, others found their niche in causing harm. Good thing we humans have some weapons—antibacterial drugs—in our arsenal! Humans, in particular, have spent millennia combating these microscopic pests, developing many methods of preservation for our foods and salves for our wounds. But it was not until the early 1900s, with the discovery of penicillin, that we learned how to use specific compounds to combat bacteria more directly. Antibacterial drugs, starting with the penicillins, are a group of medications that fight bacterial infections. They are commonly confused with antibiotics, which are a larger group of medications, better termed as antimicrobials. While antibiotics include those medications that target fungi, archaea, parasites, and bacteria, antibacterials target bacteria specifically. What is the difference between antibiotics and antibacterials? Antibiotics, better known as antimicrobials, include those medications that target fungi, archaea, parasites, and bacteria, whereas antibacterials target bacteria specifically. The discovery and subsequent use of antibacterial drugs are responsible for one of the largest jumps in life expectancy in human history. With their discovery, the most common cause of death in postindustrial countries quickly changed from communicable diseases to noncommunicable diseases, such as heart disease and cancer. Life expectancy at birth rose rapidly after their use began, and the overall proportion of elderly populations more than doubled in developed countries. Alongside other advancements in care, the introduction of antibacterial drugs in the 20th century truly transformed the face of modern medicine. Due to antibacterial resistance, use of many of the early antibacterial agents has been discontinued in modern times. Nalidixic acid (an old urinary tract infection drug), for example, is now completely replaced by ciprofloxacin and other fluoroquinolones. Since the discovery of penicillin in 1928, dozens of antibacterials have been put into (and back out of) practice in the world. Alongside their increased use, resistance to antibacterials by pathogens became more and more common, prompting the development of new, stronger agents and novel antibacterial classes. While we cannot cover every class of agent, we can break them down by three general mechanisms: Inhibiting bacterial cell wall or membrane synthesis Inhibiting DNA and/or RNA synthesis Inhibiting protein synthesis What are the three general mechanisms used by antibacterial agents? Antibacterials generally act by inhibiting cell wall or membrane synthesis, inhibiting DNA and/or RNA synthesis, or inhibiting protein synthesis. Some classes important in everyday practice are shown with their general mechanisms in Figure 1. Figure 1 Credit: ©ScholarRx This group of antibacterial agents includes a number of classes, which we can separate into cell wall inhibitors and membrane inhibitors. Cell wall inhibitors are more commonly used and include penicillins, cephalosporins, monobactams, carbapenems, and vancomycin. With the exception of vancomycin, a glycopeptide that binds to D-alanyl-D-alanine in bacterial cell walls, the rest contain β-lactam rings, which disrupt bacterial cell wall formation. These β-lactam antibacterials can have variable susceptibility to β-lactamases, enzymes in bacteria that destroy these rings. Furthermore, penicillins can be broken down into newer derivatives, including: Aminopenicillins: improved uptake versus older penicillins β-Lactamase–resistant penicillins: penicillin derivatives resistant to β-lactamase (which normally inhibits penicillin action) Ureidopenicillins: novel group of penicillin derivatives with wider spectrum Daptomycin (a lipopeptide antibacterial) and polymyxins act via disruption of cell membranes, not cell walls. Instead of targeting bacterial cell structure, this group of classes acts by inhibiting the synthesis of DNA and/or RNA. It includes sulfonamides and trimethoprim, which inhibit tetrahydrofolate formation via separate mechanisms to inhibit both DNA and RNA production, and quinolones/fluoroquinolones, which bind to transcription enzymes to destroy DNA during transcription or replication. Unlike agents that block DNA/RNA synthesis pathways, this class breaks down an existing DNA molecule. Metronidazole is a member of this class. It enters the bacterial cell via passive diffusion then forms toxic freeradical metabolites, which disrupt DNA integrity (Figure 2). It is worth noting that metronidazole has an antiprotozoal activity as well. What a beast! Figure 2 Credit: ©ScholarRx This last group of antibacterial agents acts by inhibiting protein synthesis. Usually, this is via binding to ribosomal subunits—the mechanism used by aminoglycosides, tetracyclines, glycylcyclines, and amphenicols (Figure 3). Macrolides employ a number of mechanisms but also bind to the bacterial ribosome. Lastly, linezolid, an oxazolidinone, disrupts protein production by inhibiting formation of the complex required to translate mRNA into protein. Figure 3 Credit: ©ScholarRx Although this may seem like an overwhelming amount of information, we present it here just as a general review. Remember that not all antibacterials are made the same. Some are significantly “stronger” than others but may be limited due to cost, side effects, or concern for resistance, especially when treating methicillinresistant Staphylococcus aureus (MRSA). In general, we want to use the most appropriate antibacterial for the job—a task we will discuss in more detail later. See Table 1 for a summary of these antibiotics. Table 1 Class Examples of Agents Examples of Bacterial Targets Cell Wall and Membrane Inhibitors Penicillins β-lactamaseresistant Aminopenicillins Ureidopenicillins Penicillin G, K, N, O, and V Methicillin, nafcillin, oxacillin Ampicillin, amoxicillin; used with βlactamase inhibitors Piperacillin; used with or without βlactamase inhibitors Mostly gram-positive organisms. Key in syphilis, group B streptococci Staphylococcus species Listeria, Streptococcus pneumoniae, Staphylococcus species, Enterococcus faecalis and E faecium Escherichia coli, Bacteroides species, Haemophilus influenzae, Staphylococcus aureus, Pseudomonas aeruginosa Very wide range: mostly gram-positive cocci and Cephalosporins Five generations: ceftriaxone (third), gram-negative rods; gram-negative coverage cefepime (fourth), and ceftaroline (fifth) increases with generations (fifth generation covers MRSA) Monobactams Aztreonam P aeruginosa, E coli, Klebsiella Class Examples of Agents Examples of Bacterial Targets Carbapenems Imipenem, meropenem P aeruginosa, MRSA, enterococci Glycopeptide Vancomycin MRSA, Clostridium difficile, enterococci Lipopeptide Daptomycin MRSA, enterococci Polymyxins Polymyxin B, polymyxin E Resistant P aeruginosa, gram- negative skin flora DNA and RNA Synthesis Inhibitors Sulfamethoxazole (SMX), Wide spectrum: aerobes, gram-positive and sulfasalazine, sulfadiazine gram-negative bacteria Trimethoprim Trimethoprim (usually with SMX) E coli, Proteus mirabilis, Klebsiella pneumoniae Quinolones/ fluoroquinolones Ciprofloxacin, levofloxacin, moxifloxacin E coli, Legionella, Salmonella typhi, Bacillus (all fluoroquinolones; nalidixic acid was anthracis parenteral quinolone drug) Sulfonamides Protein Synthesis Inhibitors Aminoglycosides Streptomycin, gentamicin, tobramycin, Gram-negative aerobes amikacin Tetracyclines Tetracycline, doxycycline Chlamydia species, Rickettsia species, Lyme disease Glycylcycline Tigecycline only Wide spectrum: E coli, K pneumoniae, MRSA Amphenicols Chloramphenicol, thiamphenicol Macrolides Oxazolidinone Vibrio cholerae, S aureus, S pneumoniae, Rickettsia species, E coli Erythromycin, clarithromycin, Streptococci, Staphylococci, Legionella, azithromycin Chlamydia Linezolid MRSA, vancomycin-resistant enterococci (VRE) While Table 1 may seem exhaustive, it contains only a fraction of the important information needed to know for antibacterials. It is important to consider the guidelines of therapy, escalation of therapy, cost, side effect profiles, interactions, and resistance risks as well. Our purpose here is to provide a general foundational framework for the many antibacterial agents used in clinical practice. As no antibacterial can cover every bacterium, antibacterials are often combined with other agents to cover all or most bacteria infecting a particular patient. They may also be paired with certain agents that help in their action—such as the pairing of β-lactamase inhibitors with β-lactam agents to disrupt β-lactamases. This is a difficult question to answer and requires some context. It is crucial to first understand that not all antibacterials are the same even if they have the same mechanism of action. For example, a bacterium that has strong β-lactamase expression will be very resistant to an antibacterial that uses β-lactam rings. So, we have to consider what the bacteria we’re treating may actually be susceptible to before we treat. In clinical and hospital practice, a measurement called the minimum inhibitory concentration (MIC) determines antibiotic susceptibility. MIC, as the name suggests, is the minimal antibiotic concentration required to inhibit the growth of the bacterium in question. This is different from the minimum bactericidal concentration (MBC), which is the minimum concentration of antibacterial that would result in bacterial cell death (defined as inability to reculture the bacteria). MBC is rarely used in practice, but it is used to determine if an antibacterial is more bactericidal than bacteriostatic. So, what does this all mean? Well, bacteriostatic antibacterials are agents that halt the growth of bacteria. Many of the antibacterials listed in Table 1 are bacteriostatic. When bacterial growth and replication are halted, the immunocompetent host is able to more easily clear the organism—making these agents as effective as bactericidal agents. As the MIC approaches the MBC, the agent is considered more bactericidal. Bactericidal antibacterials are those that actually kill bacteria as their primary action. MICs can often be easily calculated through assays that are commercially available, many of which can return results in about 24 hours. MBC, however, is much more difficult to obtain and has little clinical value given the efficacy of bacteriostatic action. So it is rare for MBC data to be acquired for patients outside of research purposes. Probably the most common MIC testing is methicillin with S aureus to determine if an infection is due to MRSA or methicillin-sensitive S aureus (MSSA). In the hospital or clinic, a swab of infected material can be sent for analysis. Once determined to be S aureus, it can be grown in increasing concentrations of methicillin-filled culture broth (Figure 4). If the bacteria have a significantly elevated MIC, treatment will require agents active against MRSA instead of the agents more commonly used against MSSA. Figure 4 Credit: ©ScholarRx Why is it important to check the methicillin MIC for hospitalized patients with S aureus infections? It is important to confirm the infection is not MRSA, as this often requires very different agents to treat. While we wait for cultures to identify the cause of an infection, we may not have the time to wait on starting treatment. After all, in some cases, minutes really do matter (eg, in acute bacterial meningitis), and some cultures can take days to return with species identification or susceptibility results. In these cases, empiric (“best guess”) treatment is started to cover the likely (and most dangerous) culprits. Why? We can always deescalate treatments, but if we do not properly cover patients, not only do we not adequately treat the infection, but we are also exposing them to an antibacterial that may have its own side effects without actually affecting the infection. Making the right choice in empiric treatments is thus often difficult, requiring understanding of the patient’s disease process and also local resistance rates. What is the terminology used to describe the antibacterial therapy that may be initiated before knowing the culprit organism? Empirical treatment describes the antibacterial therapy that may be initiated before knowing the culprit organism. To adequately consider empiric treatments, local surveillance (antibiotic susceptibility monitoring) data and knowledge of disease epidemiology and occurrence of sentinel pathogen(s) in inpatients are crucial. Different regions have variations in bacterial resistance rates and infection rates. Variation in infection rates may influence our initial diagnosis (eg, certain populations have significantly higher rates of syphilis infections than others), and once we know the diagnosis, we can tailor treatment based on known resistance rates. In other cases, factors specific to the patient may change our approach. Many hospitals and health departments keep track of the development of resistant strains given their massive public health impact. The development of new multidrug-resistant organisms, for example, is often a nationally or even globally newsworthy event, such as the confirmation of highly resistant Neisseria gonorrhoeae strains. For instance, imagine a patient coming to the clinic with a large thigh abscess. Although this would probably be a simple infection in some patients, this patient is a known intravenous drug user who shares needles and has had multiple emergency department visits in the past month. This significantly raises our concern for a resistant organism, most likely MRSA, but also for a complex infection. This patient is in pain and will need treatment immediately—we cannot wait for cultures. As such, this patient would likely be started on vancomycin (to cover MRSA) and a second agent to cover gram-negatives or anaerobes (such as piperacillin). If cultures return the next day showing that the infection is only MSSA, we would de-escalate treatment accordingly.