AeE 418 Compilation (1) (1) PDF - Human Factors in Aviation
Document Details
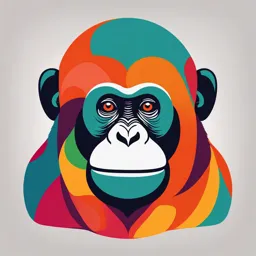
Uploaded by FineKineticArt
Tags
Summary
This document provides an introduction to human factors, tracing its historical development from early anthropometric studies to the incorporation of human limitations in aviation and other industries. It also highlights the role of regulations, standards, and the influence of human factors in aviation accidents. The document contains information on aircraft design and increasing automation in aviation.
Full Transcript
Main Topic 1: Introduction to Human Factors Human Factors It is a term that covers the science of understanding the properties of human capability, the application of this understanding to the design, development, and deployment of systems and services, and the art of ensuring successful applicatio...
Main Topic 1: Introduction to Human Factors Human Factors It is a term that covers the science of understanding the properties of human capability, the application of this understanding to the design, development, and deployment of systems and services, and the art of ensuring successful application of human factor principles into the maintenance working environment. a. Historical Development 1487 Leonardo Da Vinci conducted research in anthropometrics; his Vitruvian Man became one of the earliest guides for anthropometry. He also sketched a human-powered flying machine. Early 1900s Frank and Lillian Gilbreth worked on reducing human error in medicine, developing the challenge-response system (still used in aviation today). The Wright brothers pioneered human factors in aviation, developing unstable aircraft with controls modeled on bird flight. Their innovations included human interactive controls, engine power control, and rudder boost/trim control. World War I (1914–1918) Aviation psychology shifted focus from pilots to aircraft design, controls, and environmental effects. Aeromedical research and human testing methods were developed. Two aeronautical labs were established at Brooks Air Force Base, Texas, and Wright Field, Ohio. Post World War I The Hawthorne Effect was identified in civilian industry, showing motivational factors influenced worker productivity. World War II (1939–1945) The need to design equipment that accounted for human limitations became apparent. In 1947, Fitts and Jones studied control knob configurations in aircraft flight decks. Post-World War II (1950s-1960s) Researchers like Alphonse Chapanis, Paul Fitts, and Arnold Small led human factors studies. The Cold War spurred Department of Defense-supported research, with expanded labs and military-sponsored research. Research transitioned from small equipment to entire workstations and systems, with greater involvement from civilian engineers in equipment design. b. Regulations and Standards Human Factors in aviation and other industries is governed by a set of regulations and standards designed to enhance safety and performance. Some key regulations include: ICAO (International Civil Aviation Organization) ICAO sets global standards for aviation safety, which include guidelines for Human Factors in aircraft design, training, and operations. FAA (Federal Aviation Administration) The FAA has established Human Factors standards, particularly in areas like cockpit design, pilot training, and crew resource management (CRM). EASA (European Union Aviation Safety Agency) EASA regulates Human Factors in European aviation, focusing on ergonomics, fatigue management, and safety systems. ISO 9241 This is an international standard for ergonomics in human-computer interaction, widely applied in aviation and other industries. OSHA (Occupational Safety and Health Administration) OSHA enforces regulations that address Human Factors in workplace safety, focusing on ergonomics and reducing human errors in high-risk environments. Influence of Human Factors in Aviation Trends in Aviation Aircraft Design Process What Is Aircraft Design? Includes the conceptualization, development, evaluation, and refinement of aircraft systems and components. The Aircraft Design Process: Step By Step ○ Mission Definition A mission definition is required before the design process may commence. It is used to define the aircraft’s state and flight characteristics. The characteristics identified then serve as necessary design requirements. ○ Conceptual Design Once configurations are established, rough sketches are drawn. In this conceptual design process, designers seek to achieve all the requirements outlined by the mission definition. They must also consider features such as wing location, engine size and more. ○ Preliminary Design During the preliminary design phase, the conceptual design is optimized to fit into the necessary parameters. At this stage, approved sketches are used to make models, which are used to conduct further testing, such as wing location tests. ○ Detailed Design At this stage of the process, designers will finalize the aircraft design. All the design elements are determined and specified, as well as the quantity of elements such as ribs, spars, sections and more. Increasing Automation Cockpit Evolution CASES INVOLVING HUMAN FACTORS Case Studies (Human Factors in Aircraft Accidents) Tenerife Airport Disaster Date: March 27, 1977 What Happened: Two Boeing 747s (KLM Flight 4805 and Pan Am Flight 1736) collided on the runway at Tenerife Airport, resulting in 583 fatalities. This remains the deadliest aviation accident in history. Human Factor: Miscommunication and cockpit decision-making. The KLM crew misunderstood ATC instructions and began takeoff without clearance, exacerbated by fatigue and urgency. There was also poor communication between ATC and flight crews, partly due to language barriers and radio interference. United Airlines Flight 173 Date: December 28, 1978 What Happened: A DC-8 ran out of fuel while circling near Portland International Airport, leading to a crash that killed 10 passengers. The crew became distracted by a landing gear issue and failed to monitor fuel levels. Human Factor: Poor situational awareness and crew resource management (CRM). The captain did not delegate tasks or listen to the crew’s concerns about the fuel situation, showing a breakdown in communication and leadership within the cockpit. Air Florida Flight 90 Date: January 13, 1982 What Happened: A Boeing 737 crashed into the Potomac River shortly after takeoff from Washington National Airport, killing 78 people. The aircraft was not properly de-iced, causing an engine stall and loss of control. Human Factor: Poor decision-making and failure to follow procedures. The crew ignored evidence that ice was forming on the wings and did not de-ice the plane adequately, despite being aware of dangerous weather conditions. Complacency and lack of adherence to operational safety protocols played a role. Colgan Air Flight 3407 Date: February 12, 2009 What Happened: A Bombardier Dash 8 Q400 stalled and crashed during approach to Buffalo Niagara International Airport, killing all 49 onboard and one person on the ground. The crash was attributed to improper handling of the aircraft in response to an aerodynamic stall. Human Factor: Pilot fatigue and poor training. The captain and first officer were fatigued, having slept in crew lounges. In addition, the pilot’s inappropriate reaction to the stall warning—pulling back on the controls instead of pushing forward—revealed inadequate training on how to handle such situations. Asiana Airlines Flight 214 Date: July 6, 2013 What Happened: A Boeing 777 crashed on final approach to San Francisco International Airport. The aircraft struck the seawall just short of the runway, leading to 3 fatalities and 187 injuries. Human Factor: Mismanagement of automation and lack of situational awareness. The pilots over-relied on automated systems and failed to monitor their airspeed, causing the plane to descend too quickly. CRM issues and insufficient training on manual landing procedures also contributed. Summary Future Directions MAIN TOPIC 1: INTRODUCTION TO HUMAN FACTORS a. Historical Development The roots of Human Factors can be traced back to early industrial engineering efforts, but it gained momentum in the 20th century: Pre-20th Century Foundations: Human factors ideas existed in early manual labor studies, including ideas about optimizing worker efficiency and safety. World War I & II: These wars played a critical role in the formal development of Human Factors. Rapid advancements in technology led to increased demand for the safe and effective use of complex machinery, such as aircraft and radar systems. Researchers began to systematically study how humans interact with technology, focusing on minimizing human error. Post-War Growth (1950s–1970s): After World War II, Human Factors research expanded into civilian industries like aviation, automotive, healthcare, and nuclear power. Organizations such as the Human Factors and Ergonomics Society (HFES) were established to promote research and application. Computer Revolution (1980s–1990s): With the advent of personal computers and information systems, Human Factors evolved to address issues related to user interfaces, human-computer interaction (HCI), and cognitive ergonomics. The goal shifted from just physical aspects to also include cognitive aspects like decision-making and information processing. Modern Era (2000s–Present): Today, Human Factors has a broader scope, including automation, AI systems, and even the design of virtual and augmented reality environments. It plays a critical role in industries like healthcare, aviation, automotive, and defense. b. Regulations and Standards Regulations and standards in Human Factors ensure that systems, products, and environments are designed to meet the physical and cognitive needs of users. These regulations are often created by government bodies, industry groups, and international organizations to enhance safety and usability: ISO (International Organization for Standardization): ISO 9241: This standard focuses on ergonomics and Human-Computer Interaction (HCI). It provides guidance for designing systems and products that are easy to use, efficient, and safe. ISO 6385: Principles for designing work systems, focusing on the interaction between people and work environments. MAIN TOPIC 1: INTRODUCTION TO HUMAN FACTORS OSHA (Occupational Safety and Health Administration): OSHA enforces regulations in the workplace to ensure worker safety, including ergonomic guidelines to minimize injuries from repetitive tasks and physical strain. FDA (Food and Drug Administration): In healthcare, the FDA provides guidelines for human factors in the design of medical devices to ensure they are safe and effective for use by healthcare professionals and patients. AAMI (Association for the Advancement of Medical Instrumentation): This group publishes standards that govern the design and usability testing of medical devices, including Human Factors engineering requirements. FAA (Federal Aviation Administration): The FAA mandates Human Factors guidelines in the design of aircraft cockpits, control systems, and training procedures to enhance safety and reduce pilot error. Human Factors and Ergonomics Society (HFES): HFES also provides a variety of best practice guides, technical standards, and regulations focused on ergonomics and Human Factors research, design, and application. The Civil Aviation Authority of the Philippines (CAAP) is responsible for regulating and overseeing civil aviation in the Philippines. Its primary functions include: Air Safety: CAAP ensures that aviation safety standards are met, including the safety of aircraft, airports, and air traffic control. Licensing: It issues licenses to pilots, air traffic controllers, and other aviation personnel, as well as certifications for aircraft and aviation operations. Air Traffic Management: CAAP manages and controls the country’s airspace, coordinating flights and ensuring efficient air traffic flow. Airport Operations: It supervises and operates various airports across the Philippines, particularly those not managed by other entities like the Manila International Airport Authority (MIAA). Aviation Regulation and Enforcement: CAAP enforces aviation laws, regulations, and international standards set by organizations like the International Civil Aviation Organization (ICAO). Incident Investigation: It investigates aviation accidents and incidents to improve safety and prevent future occurrences. MAIN TOPIC 1: INTRODUCTION TO HUMAN FACTORS Overall, CAAP ensures the safe, orderly, and efficient development of the Philippine civil aviation sector. The International Civil Aviation Organization (ICAO) is a specialized agency of the United Nations that works to promote the safe and orderly development of international civil aviation. Its key functions include: Establishing Global Standards: ICAO develops international standards and regulations, known as SARPs (Standards and Recommended Practices), to ensure safety, security, efficiency, and environmental protection in international aviation. Air Navigation and Traffic Management: ICAO coordinates air traffic management (ATM) systems and air navigation to support safe and efficient international air travel. It sets guidelines for airspace, navigation, and communication systems. Security and Safety: ICAO sets standards for aviation security, airport operations, and the certification of aircraft and aviation personnel. It works to prevent unlawful interference in civil aviation and ensure accident investigation procedures. Environmental Protection: ICAO addresses the environmental impact of aviation, including issues like aircraft noise, emissions, and sustainable aviation fuel development, by creating policies to minimize the industry's environmental footprint. Capacity Building: ICAO helps member states develop their aviation infrastructure, offering training and technical assistance to improve their ability to meet international aviation standards. Cooperation among States: ICAO facilitates international cooperation among member states to ensure the harmonization of regulations and procedures in global aviation, promoting seamless travel across borders. ICAO works closely with its 193 member states to ensure that international aviation standards are uniformly applied, making air travel safer, more secure, and sustainable. Pilot's Test 1. Medical Exam: Checks physical and mental health, including vision and hearing. 2. Written Knowledge Exam: Tests knowledge of aviation rules, navigation, weather, and aircraft systems. MAIN TOPIC 1: INTRODUCTION TO HUMAN FACTORS 3. Flight Simulator Test: Assesses handling of flight situations and emergencies using a simulator. 4. Checkride (Practical Flight Test): Involves flying with an examiner to show flying skills and decision-making. 5. Instrument Rating Test: Evaluates proficiency in flying using instruments in low visibility. 6. Flight Proficiency Test: Tests specific flying maneuvers and emergency procedures. 7. Crew Resource Management Evaluation: Focuses on teamwork and communication in the cockpit. 8. Color Vision Test: Checks the ability to distinguish colors, important for reading instruments and signals. Certifications 1. Pilot Certificates: Private Pilot License: Allows flying for personal use. Commercial Pilot License: Allows flying for pay or commercial purposes. Airline Transport Pilot License: Required to be a captain for airlines. Instrument Rating: Enables flying using instruments in low visibility. Multi-Engine Rating: Allows flying aircraft with more than one engine. 2. Aircraft Type Certificates: Type Certificate: Certifies that an aircraft design meets safety standards. Supplemental Type Certificate: Approves modifications to an existing aircraft. 3. Airworthiness Certificates: MAIN TOPIC 1: INTRODUCTION TO HUMAN FACTORS Standard Airworthiness Certificate: Confirms the aircraft is safe to fly. Special Airworthiness Certificate: For specific uses, like experimental aircraft. 4. Maintenance Certificates: Airframe and Powerplant Certificate: Certifies mechanics to maintain and repair aircraft. Repairman Certificate: Allows individuals to perform specific maintenance tasks. 5. Operator Certificates: Air Carrier Certificate: Needed for airlines to operate flights. Commercial Operator Certificate: Allows non-airline operators to conduct commercial flights. 6. Part 145 Repair Station Certificates: Certifies maintenance facilities to perform repairs and inspections on aircraft. College of Engineering – Department of Electronics Engineering Main Topic #1 Introduction to Human Factors Group #1 Boongaling, Eryck John M. Hernandez, Miguel Angelo A. College of Engineering – Department of Electronics Engineering HUMAN FACTORS Human Factors and Ergonomics is a discipline concerned with the study of human interaction with systems and society’s attempt to understand how to build better systems. Prior to the development of terminology around human factors, the study of humans at work was called ergonomics. “Ergonomics” was a term first coined by the Polish scientist Jastrzeboswki. The word “Ergonomics” comes from the Greek “Ergon” meaning work and “Nomos” meaning law. This field integrates knowledge from psychology, engineering and ergonomics as it examines human potential, frailty, and interactions within environment. The above concepts of human factors enable the designers and developers to develop products, processes and environment, which do not pose a challenge to the users and hardly allow them to make errors. Human factors are important in many fields, aviation, healthcare, technology, manufacturing and many others are among the most obvious. Introducing human factors aspects in design and development brings about positive changes that eliminate conditions of mishaps, boosts productivity and thereby delivering better utility to the user. Applying lessons from anthropology, design can respect the capabilities of the users and provide solutions in terms of functionality, capability and fun to give better user experience. A. HISTORICAL DEVELOPMENT The history of human factors is an interesting one and has come a long way in terms of the way in which it looks at people and their relations to, or with systems. This journey can be divided into several stages, at which there are significant advancement and shifts because of many factors, including changing technology, switching social values, or other surprising and stunning events. Early Origins: Anthropometry and the Dawn of Human-Centered Design (1400s-Early 1900s) The emergence of the field can be best attributed to the Renaissance period that saw Leonardo da Vinci undertake anthropometry sometime in 1487. ” Vitruvian Man” is a relevant example of the Early Renaissance efforts to explore the human measurements and their application to the creations. In the earlier part of the twenty-first century, the Gilbreths built on this early work of College of Engineering – Department of Electronics Engineering concentrating more on human aspects to design challenge-response systems to minimize medical mistakes. World War II: The Catalyst for Human Factors and Ergonomics (1939-1945) This however did not gain human factors its footing as the future as the world went to the second world war. The requirements during the war such as quick mobilization and the growing complexity of technology pointed to the fact that the design of systems was largely done without much consideration for the executive capacity of man. These years witnessed the creation of aviation psychology and aeromedical research along with other systems or designs of human error. The Cold War Era: Expansion and Institutionalization (1947-1991) The Cold War era witnessed the expansion and institutionalization of human factors research, with both military and private sector involvement. Universities established human factors laboratories, and professional organizations like the Human Factors Society emerged in 1957, solidifying the field's professional status. This period saw a significant expansion of human factors research into various domains, driven by the increasing complexity of technology and the need for user-friendly interfaces. Human Performance: A Behavioral Perspective (1960s-Present) The Cold War saw the emergence of human factors as a vast field for research and many military and private collaboration. Facilities like those of industrial psychology were set up in universities, and formal groups such as the Human Factors Society soon formed in 1957 with the responsibility of professional activities. During this time, there was a noticeable growth in efforts to develop the direction of human factors research to different fields, due to the growth of technology and the demand for easy-to-use interfaces. Systems Thinking: Recognizing the Interplay of Human, Task, and System (1970s-Present) Systems thinking became the dominant perspective in HF for the same reason as in the previous paradigm, that is, it insisted on tackling faults inherent in the systems that can lead to errors. College of Engineering – Department of Electronics Engineering The Legacy of Tragedy: Human Factors in Aviation (1970s-Present) A review of aviation accidents including the Eastern Airlines crash in 1972 and the collision in Tenerife airport in 1977 showed that human factors were responsible for many of the accidents, and hence there was broader use of human factors training and education as well as the enhancement of non-technical skills. The Total System Era: A Holistic Approach to Safety (1980s-Present) Despite this, in the last few years, human factors have adopted a total system approach and acknowledges that errors can occur at any stage of a process and so, the approach implemented needs to be able to handle such errors. Human factors are an active and fluid discipline that adjusts to new technology and issues while rooted with the fundamental concepts of human oriented design and error elimination, and systems approach. An historical look at the field which has evolved from a trial-and-error type of approach to systems thinking strengthens the realization of the part it plays in developing a safer, more efficient environment.” B. REGULATIONS AND STANDARDS Human Systems Engineering (HSE) is a multidisciplinary field that requires adherence to various regulations and standards to ensure the safety, usability, and effectiveness of designed systems. Regulations and standards in this area aim to ensure that systems are designed and operated in a way that is compatible with human capabilities and limitations. These regulations and standards are often mandated by government agencies, industry bodies, or international organizations. International Organization for Standardization (ISO) A non-governmental organization that develops standards for quality, safety, and efficiency. The ISO provides common standards that help ensure products and services are safe, reliable, and of good quality. ISO develops standards across a wide range of industries, including manufacturing, technology, healthcare, and environmental management. College of Engineering – Department of Electronics Engineering International Electrotechnical Commission (IEC) A global organization that develops standards for electrical, electronic, and related technologies. The Organization ensures the safety, reliability, and quality of electrical and electronic products and systems. International Civil Aviation Organization (ICAO) A specialized agency of the United Nations that sets standards for international civil aviation. Its primary goals are to ensure the safe, orderly, and efficient development of international civil aviation throughout the world. ISO 45001 An international standard that specifies requirements for an occupational health and safety (OH&S) management system. It provides a framework for organizations to manage risks and improve OH&S performance. ISO 45001 provides an internationally-recognized framework for managing occupational health and safety risks. It enables organizations to systematically assess hazards and implement risk control measures, leading to reduced workplace injuries, illnesses and incidents. ISO 9241 A series of international standards that provide guidelines for the design of ergonomic work environments, specifically focusing on human-computer interaction (HCI). These standards aim to make technology more usable, efficient, and comfortable for people. IEC 61508 An international standard that provides a framework for functional safety in electrical, electronic, and programmable electronic safety-related systems. It is widely used in industries such as process automation, transportation, and aerospace. College of Engineering – Department of Electronics Engineering ICAO Annex 6 An international standard that sets forth the requirements for the operation of aircraft. This standard addresses human factors considerations in aviation, such as cockpit design, crew resource management (CRM), and fatigue management. It aims to ensure that aircraft are operated safely and efficiently, while minimizing the risk of accidents. ANSI/HFS 100 A standard developed by the Human Factors and Ergonomics Society (HFES) and approved by the American National Standards Institute (ANSI). It provides a comprehensive overview of the principles and practices of human factors engineering. The standard is widely used in various industries to improve the design and usability of products, systems, and environments. College of Engineering – Department of Electronics Engineering Department of Electronics Engineering Batangas State University - The National Engineering University Alangilan Campus Main II Batangas City Cognitive Systems in Aerospace In Partial Fulfillment of the Requirements of the Course AeE 418 - Human Systems Engineering for the Degree in Bachelor of Science in Aerospace Engineering Prepared by: Group 6 Chavez, Cesar Francis Reiley A. Cueto, Frances Czareena C. Gozo, Marifyl F. BSAEROENG - 4101 Engr. Alvin Rex L. Arellano Instructor September 26, 2024 College of Engineering – Department of Electronics Engineering COGNITIVE SYSTEMS IN AEROSPACE Attention and Perception DISTRACTIONS Distractions can be mental or physical, and they can disrupt a technician's concentration on a job, leading to missed details and errors. ATTENTION Attention is a fundamental cognitive process that underpins nearly every human activity. It allows us to selectively focus on relevant information, filtering out distractions and prioritizing important stimuli. Attention allows us to be vigilant and prepared for potential dangers. TYPES OF ATTENTION Sustained - focusing on a person, task or activity for a certain time or until the relevant conversation, task or activity is complete. Selective - being able to focus on a specific task while simultaneously being able to block out any background noise or stimuli. Alternating - ability to change the focus of your attention and switch between different tasks. Divided - trying to focus on many different conversations, tasks and activities at the same time, also known as multitasking. College of Engineering – Department of Electronics Engineering IMPORTANCE OF ATTENTION IN AVIATION Safety - Aviation is a high-risk environment. With this, maintaining consistent attention is needed to avoid mistakes that could lead to accidents. Safety Regulations - Aerospace engineers are known to work within strict safety regulations. Paying attention to these regulations is important to ensure aircraft meet its safety standards. Situational Awareness - In the aviation field, attention is one of the foundations of situational awareness. This allows every personnel to make informed decisions and avoid possible accidents. ATTENTION VS. PERCEPTION Attention - the concentration of awareness Perception - the way sensory information is organized, interpreted, and consciously experienced. IMPORTANCE OF PERCEPTION IN AVIATION Situational Awareness - Constantly understands the surroundings, especially the Pilots, which are built upon accurate perception of visual cues, instrument readings, and auditory signals. Decision Making - Perception influences an engineer’s ability to make quick and accurate decisions in critical situations. Design - Engineers design cockpits with human perception in mind. Ensuring the visibility, ergonomic controls, clear instrument displays, and minimizing distractions. Decision-making and Situational Awareness Decision-making in aerospace cognitive systems refers to the process by which both human operators (pilots, air traffic controllers) and automated systems (AI, autopilots) make informed choices under various conditions. The complexity of aerospace operations requires rapid, accurate decision-making, often involving multiple factors like environmental conditions, system status, and mission objectives. 1. Human-Machine Interaction Collaborative Decision-Making: In modern aviation, decisions are increasingly made through a collaboration between pilots and automated systems. Cognitive systems are designed to assist human decision-making by providing real-time data analysis, alerts, and recommendations. Automation Bias: There’s a tendency for humans to overly rely on automated systems, potentially leading to errors in judgment. Well-designed cognitive systems College of Engineering – Department of Electronics Engineering mitigate this by ensuring human operators remain engaged in the decision-making process. 2. Adaptive Automation Dynamic Task Allocation: Cognitive systems in aerospace can shift between levels of automation depending on the complexity of the task or environmental changes. This ensures that human operators are only required to make critical decisions when necessary, reducing cognitive overload. Real-time Data Processing: Decision-making systems use sensors and advanced algorithms to process vast amounts of data (weather conditions, flight parameters, etc.) in real time, aiding the pilot’s decision-making. 3. Artificial Intelligence and Machine Learning Predictive Decision Support: AI-driven systems can predict potential issues, such as engine failures or changes in weather conditions, and suggest corrective actions before a human operator can react. Autonomous Systems: Fully autonomous systems (e.g., drones) use advanced decision-making algorithms to navigate and execute missions without human intervention. Examples of Cognitive Systems in Aerospace Decision-Making: Autonomous Flight Systems: Advanced autopilot systems can handle many aspects of flight, including takeoff, landing, and navigation, while human pilots can intervene as needed for complex or critical decisions. Mission Planning Tools: Cognitive systems can analyze various factors, such as weather conditions, terrain, and threat assessments, to optimize mission planning and routing. In-Flight Decision Support Systems: These systems can provide pilots with real-time information and recommendations, such as potential hazards, weather warnings, and emergency procedures. Predictive Maintenance: Cognitive systems can analyze sensor data to predict equipment failures and schedule maintenance proactively, reducing downtime and improving operational efficiency. Situational Awareness in aerospace refers to the pilot's or operator's ability to perceive and understand what is happening in their environment, predict future states, and make decisions accordingly. It plays a crucial role in maintaining safety and efficient operations, particularly in complex and dynamic environments like air traffic control, piloting, and UAV (unmanned aerial vehicle) management. College of Engineering – Department of Electronics Engineering Key Elements: 1. Perception of the Environment: Pilots or operators must first accurately perceive elements in the environment, including other aircraft, weather conditions, air traffic control instructions, and the status of their own aircraft. 2. Understanding the Current Situation: This involves interpreting the perceived data to form a clear picture of what is happening. For instance, a pilot recognizing that changing weather conditions require immediate corrective action. 3. Projection of Future States: Based on the current understanding, the operator should be able to predict what will happen next, allowing proactive decision-making. For example, anticipating a conflict with another aircraft based on trajectory. Importance in Aerospace: Safety: SA is essential for maintaining safety in the air, where errors due to lack of awareness can lead to accidents or near-misses. Efficiency: Operators with good situational awareness can respond more quickly and accurately to changing conditions, reducing delays or inefficiencies. Automation and Human-Machine Interaction: With the increasing use of automation in cockpits, maintaining situational awareness is challenging. While automation helps in routine tasks, pilots must remain vigilant to avoid losing touch with critical aspects of flight operation. Technological Support for Situational Awareness: 1. Head-Up Displays (HUDs): HUDs project critical flight information on a transparent display directly in the pilot’s line of sight, enhancing awareness without diverting attention from the outside environment. 2. Enhanced Vision Systems (EVS): These systems use infrared or other sensors to provide a clearer view of the environment, especially in poor visibility conditions. 3. Synthetic Vision Systems (SVS): SVS generate a 3D view of the terrain and surrounding airspace, allowing pilots to visualize the environment even in poor weather or darkness. 4. Traffic Collision Avoidance System (TCAS): This system alerts pilots to nearby aircraft that may pose a collision risk, improving situational awareness regarding surrounding traffic. Challenges: Over-reliance on Automation: When pilots become too dependent on automated systems, they may lose situational awareness, particularly in emergencies or when the system fails. College of Engineering – Department of Electronics Engineering Information Overload: Modern cockpits present a lot of data, and processing all of it can sometimes lead to reduced situational awareness if the operator is overwhelmed. Fatigue and Stress: Mental fatigue or high levels of stress can also reduce situational awareness, making it harder to stay vigilant over long flights or during critical phases of flight. Examples of cognitive systems in aerospace: Head-up displays (HUDs): HUDs can overlay information, such as flight path, altitude, and target data, directly onto the pilot's field of view, improving situational awareness and reducing pilot workload. Traffic collision avoidance systems (TCAS): TCAS uses radar and other sensors to detect potential collisions and provide avoidance instructions to pilots. Autonomous flight systems: Autonomous flight systems can use AI and machine learning to navigate aircraft and make decisions in complex environments, reducing the workload on pilots and improving safety. Memory and Information Processing Professionals Involved in Memory and Information Processing in Aerospace ATC Pilot Airside Safety Personnel Aerospace engineer Astronaut Space Mission Controllers Senses Used in Memory and Information Processing in Aerospace Aural Visual Mental Kinesthetic INFORMATION PROCESSING The ability to effectively process and respond to information within a required timeframe varies depending on individual factors such as age, health, stress, experience, environment, and workplace culture, as well as task-specific demands and inherent limitations. INFORMATION PROCESSING CAPABILITIES: College of Engineering – Department of Electronics Engineering - important in designing and delegating tasks to ensure that the information processing requirements fall within the capabilities of employees and colleagues (i.e. within their memory, attention and decision-making capabilities) Failure to see information Misunderstanding information Handling the information incorrectly Forgetting the information Reacting inappropriately BUILDING BLOCKS OF HUMAN INFORMATION PROCESSING 1. Sensing 2. Perceiving 3. Decision-making 4. Motor action or performing SITUATIONAL AWARENESS AND INFORMATION PROCESSING It is by relying on interpreting data from our senses, instruments, and communications. In simple situations, awareness is accurate, but in complex or stressful ones, processing large amounts of information becomes difficult, reducing awareness. Effective information processing is essential to maintaining awareness, especially under pressure. Cue Processing and Situational Awareness Cue Processing refers to detecting, interpreting, and responding to environmental stimuli. In aviation, it is essential for situational awareness, decision-making, and safety. Both human operators and automated systems rely on cues from instruments, sensors, and the environment to manage aircraft operations. Situational Awareness - Pilots process cues from instruments, weather, and air traffic to maintain an accurate mental model of flight conditions. Cockpit displays and HUDs provide critical data to support real-time adjustments. Cue Diagnosing - Automated systems like Health and Usage Monitoring Systems (HUMS) assess aircraft performance by processing cues from sensors, diagnosing issues before failures occur. Automation and Decision-Making - Systems like autopilot and collision avoidance systems interpret sensor data to adjust flight paths. Human pilots monitor these systems and intervene when necessary, especially in complex situations. College of Engineering – Department of Electronics Engineering Human-Machine Interaction - Technologies like augmented reality (AR) provide visual cues to technicians for aircraft maintenance, enhancing accuracy and reducing errors. Information Processing in Early Aviation Manual Flight Operations Basic Instruments Weather Cues Navigation Communication Challenges in Early Aviation Lack of real-time data and unreliable instruments increased error rates. Heavy cognitive demands, especially in poor weather or complex scenarios, led to frequent accidents. Early Advances Gyroscopic Instruments and early autopilots reduced cognitive load by providing real-time orientation data and automating basic flight functions. Radio navigation and communication improvements enhanced information flow between pilots and ground stations. MEMORY Memory retention in aerospace is critical for both pilots and aerospace professionals, as they must recall complex procedures, flight protocols, emergency responses, and technical knowledge. This involves both short-term memory (handling real-time tasks and dynamic situations) and long-term memory (storing and retrieving information learned through training and experience). Effective memory retention ensures safety, efficiency, and reliability in various operations. Types of Memory 1. Sensory memory 2. Working and short-term memory 3. Long-term memory 4. Motor memory Memory Retention in Aerospace College of Engineering – Department of Electronics Engineering Training and Repetition - Pilots and crew undergo rigorous training to develop strong memory retention of emergency procedures, aircraft systems, and standard operating protocols. Continuous repetition reinforces long-term memory, allowing quick recall under pressure. Checklists and Procedures - While memory plays a major role, aviation uses checklists as backups, ensuring key steps are not missed, especially during critical phases like takeoff, landing, or emergencies. Simulations and Drills - Regular simulation exercises help pilots and engineers practice real-life scenarios, reinforcing procedural memory and improving retention of emergency responses. Challenges in Memory Retention: Cognitive Overload - Handling large amounts of information under stress can overwhelm memory, leading to errors in recall. Skill Decay - Without regular practice or exposure, memory retention of rarely-used procedures can decline over time, making periodic retraining essential. References: Attention and Perception https://global.sacap.edu.za/blog/applied-psychology/types-of-attention/ https://www.mentalup.co/blog/types-of-attention Decision-making https://ntrs.nasa.gov/ https://ieeexplore.ieee.org/Xplore/home.jsp https://www.sciencedirect.com/ https://www.mitre.org/ https://www.asma.org/journal Situational Awareness https://journals.sagepub.com/doi/10.1518/001872095779049543 https://psycnet.apa.org/record/2004-11388-001 https://journals.sagepub.com/doi/10.1518/001872008X288420 https://psycnet.apa.org/record/2019-06879-000 College of Engineering – Department of Electronics Engineering https://www.researchgate.net/publication/267624130_Human_Factors_Metho ds_A_Practical_Guide_for_Engineering_and_Design_second_edition https://www.pacdeff.com/pdfs/AviationSA-Endsley%201999.pdf https://journals.sagepub.com/doi/full/10.1177/1555343419872050 Memory and Information Processing https://skybrary.aero/articles/memory-oghfa-bn https://www.sciencedirect.com/science/article/abs/pii/S0925753505000664 https://escholarship.org/content/qt7657f2k8/qt7657f2k8_noSplash_38757f1bc 779a9f910ba389d7af6a51a.pdf?t=op2lnd https://skybrary.aero/articles/information-processing https://corescholar.libraries.wright.edu/psychology/289/ COGNITIVE SYSTEM IN AEROSPACE TOPIC 2 What Is A cognitive system refers to the mental processes that allow us to think, learn, remember, and solve problems. COGNITIVE It includes things like perception (how we see SYSTEM? and understand the world), attention (what we focus on), memory (how we store and recall information), and reasoning (how we make decisions). Essentially, it’s how our brains process information and help us interact with our environment. cognitive system in aerospace Cognitive systems in aerospace engineering focus on understanding how human mental processes, such as attention, perception, decision-making, and memory, influence performance in complex environments like aviation. As aerospace engineering relies on high precision and safety standards, integrating cognitive systems into aerospace technology ensures that both human and machine function optimally together. Pilot attention & perception & spatial cogniton 01 Pilot attention & perception & spatial cognition Visual attention A vast amount of the pilot’s primary flight tasks of aviating and navigating are served by visual channels of information. The number of displays and alerts on the modern flight deck can be overwhelming, and while the view out the cockpit (OTC) is generally less complex, it can be correspondingly more important and often degraded. supervisory control discrete event detection visual search information integration supervisory control The generic task of supervisory control (Sheridan, 2002) describes the monitoring of a number of sources of dynamic information in the service of two generic subtasks: assuring that all variables are within their normal range, and controlling some of these variables to restore their normal range or to follow commands. This latter task defines tracking Four key functional variables that characterize the pilot’s gaze as it moves around and outside the cockpit to seek information. 01 Percentage dwell time (PDT) 02 Dwell duration 03 Scan pattern 04 Period of Neglect Percentage dwell time (PDT) - the percentage, or proportion of time that the gaze is on any one Four key functional instrument or area of interest (AOI). variables that characterize the Dwell duration - measures the amount of time the pilot’s gaze as it moves eye remains fixated on an AOI before leaving it, around and outside the independent of how frequently it is scanned. cockpit to seek information. Scan pattern - describes the sequence of AOIs that are fixated. Period of neglect - during which a critical AOI is not scanned, and the pilot may thus be vulnerable if a critical event occurs there while attention is elsewhere. Salience (S): The gaze is attracted to more salient AOIs, for example those that are large SEEV or colored. model Effort (E): The gaze is inhibited to visit AOI’s that are a farther distance away, because of the added effort of eye movements, and particularly head rotations to get there. Expectancy (E): The eye visits more frequently those AOIs that have a high bandwidth. Value (V): The eye visits AOIs more frequently that serve more valuable tasks. Discrete event detection NSEEV model of noticing Visual Eccentricity Salience Mental Workload Expectancy Signal detection theory Visual search Three fundamental elements of visual search Target Non - Target Distractors clutter Fixes: Decluttering Discrimination Highlighting Intelligent Target cueing Information integration The Proximity Compatibility Principle Spatial Proximity Information sources that need to be compared, or integrated in a task (close mental proximity) should be in close physical proximity on the display Information requiring only focused attention on one source, need not, and often should not be close to other sources. Nonspatial Proximity Proximity in information space Color proximity Objectness Objectness and 3D perspective displays From Attention to Perception: VISUAL AND VESTIBULAR ILLUSIONS Introduction to Perception in Aviation Visual and vestibular information is crucial for spatial orientation. Illusions can distort a pilot's perception, leading to errors. 02 Top-Down vs. Bottom-Up Processing Top-Down Processing: Driven by expectations and prior experiences. Bottom-Up Processing: Based on sensory input from the environment. 03 Visual Illusions in Aviation Slanted Runway Effect: Impact: Can result in hard landings or stalls. Pilots may misjudge the angle of descent due to runway slope. 04 Vestibular illusions and spatial disorientation What are Vestibular Illusions? Occur when the vestibular system (responsible for balance and spatial orientation) provides inaccurate information to the brain, often conflicting with visual cues. These illusions commonly happen in situations with poor visual references (e.g., flying in clouds or at night). SPATIAL COGNITION IN AVIATION introduction to spatial cognition Definition: Spatial cognition involves understanding the position and movement of objects in space relative to oneself. Importance in Aviation: Crucial for pilots to maintain situational awareness, navigate, and avoid hazards. 01 Spatial Cognition Components Mental Representation: Pilots form mental maps of aircraft's spatial properties, such as bank and airspeed. Geographical Awareness: Awareness of aircraft position relative to destinations (e.g., waypoints) and hazards (e.g., terrain, weather, traffic). 02 Frames of Reference in Spatial Cognition Egocentric: Information is referenced to the pilot’s body coordinates (e.g., left- right, up-down). Exocentric: External frame of reference showing airspace relative to the aircraft. 03 Maps in Spatial Navigation Support for Pilot's Navigation: Maps, whether paper or electronic, provide critical navigation support. Frame-of-Reference Considerations: 2D vs. 3D maps, rotating maps, and clutter management in electronic displays. 04 Spatial Awareness and Flight Displays Fully Egocentric Displays: Ideal for maintaining accurate flight paths as they replicate control-display compatibility. Exocentric Displays: Provide broader awareness of airspace, especially for terrain, traffic, and weather monitoring. DECISION MAKING IN AEROSPACE 02 DECISiON-MAKING Decision-making in aerospace involves selecting appropriate actions in real-time, often under high pressure. Decisions must be informed by a mix of experience, training, and available data. Rule-based decisions Skill-based decisions Knowledge-based decisions Situational awareness IN AEROSPACE 01 SITUATIONAL AWARENESS In Endley’s definition, situational awareness is the perception of elements in the environment, comprehension of their meaning, and projection of their status in the near future. Components of Situational Awareness: Spatial Awareness System Awareness Task Awareness 02 Methods to measure sa SAGAT (Situational Awareness Global Assessment Technique): Freezing a task and querying operators about the state of the task. SPAM (Situation Present Assessment Method): Real-time queries during operations to assess situational awareness 03 Factors affecting sa Workload Interactions: Higher workload can reduce SA by overwhelming cognitive resources. Self-Regulation: Adjusting task focus to regain SA under high demands 04 technological supports Head-Up Displays (HUDs) Enhanced Vision Systems (EVS) Synthetic Vision Systems (SVS) Traffic Collision Avoidance System (TCAS) MEMORY & Information PROCESSING 01 Memory & Information Processing Memory is critical in aerospace operations for recalling procedures, protocols, and previous experiences. Working Memory Long-term Memory Information processing involves the way the brain receives, stores, and retrieves information, which influences decision-making and performance in real-time. Human-Information Processing (HIP) Model Cognitive Load Theory THANK YOU! College of Engineering – Department of Electronics Engineering Main Topic 2: Cognitive Systems in Aerospace Group 2 Members: Dalangin, Verlo Junreneil A. Ramirez, Queen Layka. College of Engineering – Department of Electronics Engineering COGNITIVE SYSTEMS IN AEROSPACE Cognitive systems refers to the human mental processes involved in perception, decision-making, memory and awareness. In aerospace, these systems are critical to both manual operations (pilots, air traffic controllers) and automated systems (cockpit automations, AI-based assistance). 1. Importance in Aerospace Safe and efficient aerospace operations rely on the cognitive abilities of human operators, enhanced or complemented by advanced technology. Misjudgments, distractions, and cognitive overload can lead to errors, making the understanding of these systems key for safety and performance improvements. 2. Challenges in Aerospace Dynamic environments, high stress, and multitasking are common in aerospace operations, requiring well-developed cognitive systems and technological support. Mitigating human errors through improved cognitive design is crucial for flight safety, automation development, and training. A. ATTENTION AND PERCEPTION ATTENTION Attention refers to the cognitive process of selectivity concentrating on a particular piece of information or task while ignoring others. In aerospace, attention is critical due to the high cognitive demands of monitoring multiple systems and maintaining control in complex environments. Types of Attention in Aerospace Selective Attention - Focusing on specific stimuli while ignoring irrelevant information. ○ Application: A pilot may need to concentrate on vital flight instruments or incoming radio communications during critical phases of flight (takeoff, landing) while ignoring irrelevant data. Divided Attention - The ability to focus on and process multiple sources of information or tasks simultaneously. ○ Application: Pilots often engage in multitasking, such as flying the plane, adjusting navigation settings, and communicating with air traffic control, all while monitoring the systems. College of Engineering – Department of Electronics Engineering Sustained Attention (Vigilance) - The ability to maintain prolonged focus on tasks over extended periods, which is particularly important in aerospace, where long-haul flights and continuous monitoring of aircraft systems are common. ○ Application: Monitoring flight parameters during cruising altitudes where activity is low but critical systems still need oversight. Challenges to Maintaining Attention Cognitive Overload: Pilots and controllers are often required to process massive amounts of information. Cognitive overload can impair attention, leading to errors in judgment or missed information. Stress and Fatigue: Aerospace professionals, particularly pilots on long flights or air traffic controllers working in high-stress environments, are susceptible to attention lapses due to fatigue, which reduces the ability to maintain sustained attention. Automation-Induced Complacency: Modern cockpits rely heavily on automation, which can reduce the need for constant manual monitoring, leading to decreased attentiveness. When the operator is required to intervene, they may not be fully prepared due to inattentiveness. PERCEPTION Perception is the cognitive process that allows individuals to interpret sensory input—such as visuals, auditory, and spatial information—into meaningful representations of the environment. In aerospace, accurate perception is essential for effective decision-making and situational awareness. Types of Perception in Aerospace Visual Perception - The ability to process visual information from the external environment and from flight instruments. ○ Application: Pilots rely heavily on visual perception to monitor instrument panels, identify other aircraft, assess weather conditions, and navigate visually during takeoff and landing. ○ Challenges: Situations such as low visibility (fog, clouds, night) can impair visual perception. This can lead to disorientation, often referred to as spatial College of Engineering – Department of Electronics Engineering disorientation, a condition in which a pilot loses their sense of position relative to the earth's surface. Auditory Perception - The ability to process and interpret sounds, such as radio communications, alarms, and engine noise. ○ Application: Pilots and air traffic controllers depend on clear and accurate auditory perception for communication and responding to alarms or alerts. For example, engine sounds can sometimes indicate mechanical issues, while alarms warn of system malfunctions. ○ Challenges: Noisy environments in the cockpit can impair auditory perception, making it harder for pilots to hear critical communications or alarms. Spatial Perception - The ability to perceive the position, movement, and orientation of oneself or an object relative to other objects. ○ Application: Pilots use spatial perception to judge altitude, speed, direction, and proximity to other aircraft or terrain. Air traffic controllers rely on spatial perception to manage the positioning of multiple aircraft in a three-dimensional space. ○ Challenges: Spatial disorientation can occur due to poor visibility or complex flight dynamics (e.g., sharp turns or climbs), causing pilots to misjudge the aircraft's orientation. Challenges to Perception in Aerospace Visual Illusions: Environmental factors, such as weather, lighting, or atmospheric conditions, can create illusions that mislead pilots. For instance, a bright runway can appear closer than it actually is, leading to improper landings. Perceptual Overload: In high-pressure scenarios, such as emergency situations, the pilot or controller may receive more sensory input than can be processed accurately, leading to poor decision-making. Equipment Dependence: Pilots often rely heavily on instruments (e.g., radar, altimeter), and sensor failures or malfunctions can mislead their perception of the aircraft's status and environment. College of Engineering – Department of Electronics Engineering B. DECISION-MAKING IN AEROSPACE Decision-making is the process of selecting a course of action from several options. In aerospace, decisions must often be made quickly and accurately under conditions of uncertainty or stress. Two main types of decision-making occur in aerospace: Naturalistic (Intuitive) Decision-Making - Decisions are made based on experience, intuition, and pattern recognition, often without extensive analysis. This process is faster but relies on prior knowledge and expertise. ○ Application: Pilots often rely on naturalistic decision-making in rapidly changing situations, such as responding to sudden weather changes or mechanical failures. Analytical Decision-Making - A more deliberate and calculated approach, involving the systematic evaluation of data and potential outcomes. This type of decision-making is slower and often used in pre-flight planning or during less time-sensitive operations. ○ Application: Flight planning, such as choosing the most fuel-efficient route, or troubleshooting a non-critical system issue during cruise. Factors Influencing Decision-Making Time Pressure: In aerospace, decisions often need to be made within seconds or minutes, such as in emergency situations (e.g., engine failure, sudden weather changes). Pilots and controllers must rely on their training and experience to make quick decisions with limited time for reflection. Risk and Uncertainty: Aerospace operations involve inherent risks, and decision-makers often have to choose between options that carry various levels of uncertainty. For example, a pilot may need to decide whether to land in uncertain weather conditions or divert to an alternate airport. Stress and Fatigue: High-stress environments, such as managing an in-flight emergency, and fatigue from long-haul flights can impair cognitive functioning and decision-making abilities. Experience and Expertise: Pilots with more flight hours and experience tend to make faster, more accurate decisions due to their greater exposure to diverse situations. Experienced pilots are better at recognizing patterns and responding intuitively to complex scenarios. College of Engineering – Department of Electronics Engineering Technological Support for Decision-Making Flight Management Systems (FMS): These systems automate much of the decision-making involved in route planning, navigation, and fuel management. They provide real-time information to help pilots make informed decisions about flight paths, speeds, and altitude adjustments. Decision Support Systems (DSS): DSSs integrate data from multiple sources (weather, traffic, system status) and use algorithms to provide pilots and controllers with optimal decision-making solutions in real-time. They assist by suggesting alternate routes or maneuvers based on changing conditions. Artificial Intelligence (AI) in Decision-Making: AI-driven systems can predict potential risks (e.g., collision risks, system failures) and suggest corrective actions before an incident occurs, improving decision-making efficiency and reducing human error. Training and Simulations: Modern training programs for pilots and controllers use high-fidelity simulators that replicate real-world scenarios. These simulations help improve decision-making under pressure by exposing trainees to emergency and high-stress situations in a controlled environment. C. Situational Awareness Situational awareness (SA) in aerospace refers to the ability of pilots, air traffic controllers, and other aviation personnel to perceive, comprehend, and predict events and conditions in their environment. The cognitive system behind situational awareness involves several key components: Cognitive Processes in Situational Awareness Attention: The ability to focus on relevant information while filtering out distractions. Memory: Storing and recalling relevant knowledge, such as procedures and past experiences. Decision-Making: Evaluating options and making informed choices based on the perceived situation. College of Engineering – Department of Electronics Engineering Enhancing Situational Awareness 1. Training: Simulation and scenario-based training help develop and refine situational awareness skills. 2. Technology: Advanced cockpit displays, augmented reality, and automated systems can enhance the pilot's ability to gather and process information. 3. Communication: Clear communication among crew members and with air traffic control is vital for maintaining situational awareness. D. Memory and Information Processing In aerospace, memory and information processing are crucial for effective decision-making and situational awareness. The cognitive system involved includes several key elements: Key Components of Memory and Information Processing 1. Types of MemWorking Memory: The ability to hold and manipulate information temporarily. For pilots, this is crucial for managing tasks ory: like navigating and communicating simultaneously. ○ Long-Term Memory: Stores knowledge and experiences over time. This includes procedures, checklists, and past flight experiences ○ Short-term Memory: STM receives, holds and processes information from sensory memory. Processing in STM is necessary before transferring to LTM. Retrieves information from LTM. ○ Sensory Memory :Each sensory system has a corresponding sensory memory. Stores and transforms the stimuli into a form that can be processed. 2. Information Processing: ○ Perception: Gathering sensory information from the environment, including visual, auditory, and instrument data. ○ Attention: Focusing on relevant information while filtering out distractions. Selective attention is vital for prioritizing critical data during flight. College of Engineering – Department of Electronics Engineering ○ Encoding: The process of converting perceived information into a form that can be stored in memory. ○ Retrieval: Accessing stored information when needed, which is essential during critical phases of flight. Cognitive Load Cognitive load refers to the amount of mental effort being used in the working memory. Managing cognitive load is crucial in aerospace, as high loads can impair decision-making and situational awareness. Enhancing Memory and Information Processing 1. Training: Repetitive simulation training helps improve memory retention for procedures and enhances information processing skills. 2. Checklists: Using structured checklists reduces cognitive load and aids memory recall during critical tasks. 3. Automation: Advanced cockpit technologies can assist with information processing, allowing pilots to focus on higher-level decision-making. College of Engineering – Department of Electronics Engineering References: Memory (OGHFA BN). (2022, June 19). SKYbrary Aviation Safety. https://skybrary.aero/articles/memory-oghfa-bn Information Processing. (2022, June 19). SKYbrary Aviation Safety. https://skybrary.aero/articles/information-processing Lintern, G. (2011). The Airspace as a Cognitive System. International Journal of Aviation Psychology, 21(1), 3–15. https://doi.org/10.1080/10508414.2011.537556 Woods, D. D. (1993, November 1). Cognitive engineering in aerospace applications. NASA Technical Reports Server (NTRS). https://ntrs.nasa.gov/citations/19940017346 ` College of Engineering – Department of Electronics Engineering Main Topic 3: Human Processing Under Stress Group 1 Members: Ambay, Francis Cedrick M. Bragas, Kailin E. Garcia, Alexander Dave B. Manalo, Czyrus Jamiel S. ` College of Engineering – Department of Electronics Engineering What is Human Information Processing? Human information processing theory deals with how people receive, store, integrate, retrieve, and use information. Human Information Processing (HIP) refers to the cognitive science metaphor that views the mind and computer as symmetrically coupled information processors, focusing on how information is sensed, accessed, and transformed in the human mind and its implications for computer information processing. Human information processing is an approach to the study of human thought and behavior developed beginning in the 1950s as an alternative to the behavioral approaches that were popular at that time. It is a cognitive approach that is often equated with contemporary cognitive psychology. The central tenet of the information-processing approach is that the human can be characterized as an information-processing system, which encodes input, operates on that information, stores and retrieves it from memory, and produces output in terms of actions. Principles of the information processing approach According to Huitt (2003), there are a few basic principles that most cognitive psychologists agree with: The mental system has limited capacities, i.e. bottlenecks in the flow and processing of information, occur at very specific points A control mechanism is required to oversee the encoding, transformation, processing, storage, retrieval and utilization of information. This control mechanism requires processing power and that varies in function of the difficulty of the task. There is a two-way flow of information. Sensory input is combined with information stored in memory in order to construct meaning. The human organism has been genetically prepared to process and organize information in specific ways. Basic Human Information Processing Building Blocks 1. Sensing - This refers to the initial reception of external stimuli, like sound or light, which form brief sensory memories. For example, visual memory lasts less than a second, while auditory memory (echoic) lasts up to 8 seconds. 2. Perceiving- Perception is how we make sense of what we see or hear by creating an internal image of the world around us. 3. Deciding- Deciding is the process of selecting the most appropriate action for a given situation. 4. Acting- Acting involves executing the chosen action, whether it’s adjusting the plane’s trajectory, communicating with air traffic control, or making system changes. ` College of Engineering – Department of Electronics Engineering Factors affecting Human Information Processing STRESS Stress is the subconscious response to the demands placed on a person. It is a physiological and psychological response to external or internal stimuli that disrupts an individual's normal equilibrium. It encompasses physical, mental, and emotional strain, often arising from various factors such as workload, environmental conditions, and interpersonal dynamics within the cockpit or crew environment. Everyone handles stress differently and particular situations can bring about different degrees of difficulty for different people. For example, working under a strict timeline can be a stressor for one person and normal for another. The causes of stress are referred to as stressors. They are categorized as physical, psychological, and physiological stressors. Physical stressors originate from the external environment or physical conditions that exert strain on the body. They add to the personnel’s workload and make it uncomfortable for him or her in their work environment. Examples: Temperature—high temperatures in the hanger increases perspiration and heart rate causing the body to overheat. Low temperatures can cause the body to feel cold, weak, and drowsy. Noise—hangers that have high noise levels (due to aircraft taking off and landing close by) can make it difficult for maintenance personnel to focus and concentrate. ` College of Engineering – Department of Electronics Engineering Lighting—poor lighting within a work space makes it difficult to read technical data and manuals. Likewise, working inside an aircraft with poor lighting increases the propensity to miss something or to repair something incorrectly. Confined spaces—small work spaces make it very difficult to perform tasks as technicians are often contorted into unusual positions for a long period of time. Psychological stressors are events or situations that challenge an individual's mental or emotional state. Examples: Work-related stressors—over anxiousness can hinder performance and speed while conducting maintenance if there is any apprehension about how to do a repair or concerns about getting it done on time. Financial problems—impending bankruptcy, recession, loans, and mortgages are a few examples of financial problems that can create stressors. Marital problems—divorce and strained relationships can interfere with one’s ability to perform their job correctly. Interpersonal problems—problems with superiors and colleagues due to miscommunication or perceived competition and backstabbing can cause a hostile work environment. Physiological stressors include fatigue, poor physical condition, hunger, and disease. Poor physical condition—trying to work when ill or not feeling well can force the body to use more energy fighting the illness and less energy to perform vital tasks. Improper meals—not eating enough, or foods lacking the proper nutrition, can result in low energy and induce symptoms like headaches and shaking. Lack of sleep—fatigued, the maintainer is unable to perform to standard for long periods of time and can become sloppy with repairs and miss important mistakes. Conflicting shift schedules—the effect of changing sleep patterns on the body’s circadian cycle can lead to a degradation of performance. FATIGUE Fatigue is defined as a state of physical and/or mental weariness that extends beyond normal tiredness, significantly impairing an individual's ability to perform safely. It can be categorized into physical fatigue, which affects muscle performance, and mental fatigue, which impacts attention and cognitive function. The International Civil Aviation Organization (ICAO) describes fatigue as a "physiological state of reduced mental or physical performance capability resulting from sleep loss, extended wakefulness, circadian phase, and/or workload" Fatigue is a major human factor that has contributed to many maintenance errors resulting in accidents. Fatigue can be mental or physical in nature. Emotional fatigue also exists and affects mental and physical performance. A person is said to be fatigued when a reduction or impairment in any of the following occurs: cognitive ability, decision-making, reaction time, coordination, speed, strength, and balance. Fatigue reduces alertness and often reduces a person’s ability to focus and hold attention on the task being performed. ` College of Engineering – Department of Electronics Engineering Symptoms of fatigue may also include short-term memory problems, channeled concentration on unimportant issues while neglecting other factors that may be more important, and failure to maintain a situational overview. A fatigued person may be easily distracted or may be nearly impossible to distract. He or she may experience abnormal mood swings. Fatigue results in an increase in mistakes, poor judgment, and poor decisions or perhaps no decisions at all. A fatigued person may also lower his or her standards. Tiredness is a symptom of fatigue. However, sometimes a fatigued person may feel wide awake and engaged in a task. The primary cause of fatigue is a lack of sleep. Good restful sleep, free from drugs or alcohol is a human necessity to prevent fatigue. Fatigue can also be caused by stress and overworking. A person’s mental and physical state also naturally cycles through various levels of performance each day. Variables such as body temperature, blood pressure, heart rate, blood chemistry, alertness, and attention rise and fall in a pattern daily. This is known as one’s circadian rhythm. A person’s ability to work (and rest) rises and falls during this cycle. Performance counter to circadian rhythm can be difficult. Until it becomes extreme, a person may be unaware that he or she is fatigued. It is easier recognized by another person or in the results of tasks being performed. This is particularly dangerous in aviation maintenance since the lives of people depend on maintenance procedures performed at a high level of proficiency. Working alone when fatigued is particularly dangerous. Types of Fatigue Transient Fatigue - This is acute fatigue that occurs due to extreme sleep restriction or prolonged wakefulness within a short timeframe, typically 1 to 2 days. Often results from a single night of inadequate sleep or long working hours without rest. Cumulative Fatigue - This type of fatigue builds up over time due to repeated mild sleep restriction or extended hours awake across several days. Common in individuals who consistently get less sleep than needed, leading to a "sleep debt" that accumulates and requires several days of recovery to fully resolve. Circadian Fatigue - This refers to reduced performance during nighttime hours, particularly during an individual's "window of circadian low" (WOCL), which typically occurs between 2:00 a.m. and 5:59 a.m. Often experienced by shift workers or those traveling across time zones, affecting alertness and cognitive function during their natural sleep periods. Physiological Fatigue - Resulting from physical exertion, lack of sleep, or poor dietary choices. Chronic Fatigue - Lasting longer than six months and not improving with rest; often associated with underlying health conditions. STANDARD DUTY HOURS AND REST PERIODS Under the PCAR Part 8 - Operations: Part 8.11.1.3 - Duty And Rest Periods: All Crew Members (a) With respect to duty periods: ` College of Engineering – Department of Electronics Engineering (1) Persons are considered to be on duty if they are performing any scheduled or unscheduled tasks on behalf of the Operator. (2) No Operator may schedule: (i) A flight crew member for more than 14 hours of duty, except as prescribed by the Authority. (ii) A cabin crew member for more than 14 consecutive hours of duty, except as prescribed by the Authority. (iii) A flight operations officer/aircraft dispatcher for more than 10 consecutive hours of duty, except as prescribed by the Authority. (b) With respect to rest periods: (1) The minimum rest period for flight crew members and flight operations officer/dispatcher is considered to be 8 consecutive hours, unless otherwise prescribed by the Authority. (2) The minimum rest period for cabin crew members shall be 9 consecutive hours, unless otherwise prescribed by the Authority. (3) The operator shall relieve the flight crew member, cabin crew or flight operations officer/flight dispatcher from all duties for 24 consecutive hours during any 7 consecutive day period. (4) Time spent in deadhead transportation, that an operator requires of a flight crew member and provides to transport the crew member to an airport/heliport at which he/she is to serve on a flight as a crew member, or from an airport/heliport at which he/she was relieved from duty to return to his/her home station, is not considered part of a rest period. (5) No operator may assign, nor may any person: (i) Perform duties in commercial air transportation unless that person has had at least the minimum rest period applicable to those duties as prescribed by the Authority, or (ii) Accept an assignment to any duty with the operator during any required rest period. Part 8.11.1.8 - Flight Time, Duty and Rest Periods: Flight Crew Members (a) Except as provided in paragraph (b) of this section, no operator shall assign any flight crew member to a duty period exceeding: (1) 14 hours in any 24 consecutive hours for aircraft certificated for two flight crew members. (2) 16 hours in any 24 consecutive hours for aircraft certificated for three flight crew members. … ` College of Engineering – Department of Electronics Engineering Under the Federal Aviation Administration (FAA) 14 Code of Federal Regulations (CFR) Part 135 - Operating Requirements: Commuter and on Demand Operations and Rules Governing Persons on Board Such Aircraft: Subpart F - Crewmember Flight Time and Duty Period Limitations and Rest Requirements Maximum Duty Hours Per Day: Under 14 CFR Part 135, the maximum duty day for flight crew members is generally 14 hours, which includes all time from report to the end of the last flight of the day. This is designed to ensure that crew members do not exceed safe operational limits due to fatigue. Resting Period Requirements: The regulations stipulate a minimum rest period of 10 hours between duty periods. This ensures that crew members have adequate time to recover before their next duty cycle. Weekly and Monthly Limits: As per the regulations, crew members cannot exceed 60 duty hours in any 7 consecutive days and are limited to a maximum of 190 duty hours in any 28 consecutive days. Annual Limits: The total annual flight time is capped at 900 hours, ensuring that crew members do not accumulate excessive flying hours over the year. WORKLOAD MANAGEMENT What is Workload management? - balances tasks evenly across employees according to availability, skill sets, and overall business goals. Why is it important? - it helps individuals and teams prioritize tasks, improve efficiency, and reduce stress. https://productive.io/blog/workload-management/ Here are several key topics under workload management in the aviation industry: Crew Scheduling: Strategies for optimizing pilot and crew schedules to minimize fatigue and ensure compliance with regulations. Task Prioritization: Techniques for prioritizing tasks in high-pressure environments, including decision-making processes during flight operations. - Identifying safety-critical tasks first. - Addressing time-sensitive tasks promptly. - Efficiently allocating resources. -Focusing on critical tasks for overall progress. - Staying adaptable to unexpected changes. -Maintaining open communication and collaboration within teams ` College of Engineering – Department of Electronics Engineering Human Factors and Ergonomics: Understanding how human capabilities and limitations affect workload, including cockpit design and user interface. Training and Simulation: The importance of training programs that prepare crew for high-workload scenarios, using simulators to practice decision-making under pressure. Regulatory Compliance: Adhering to aviation regulations regarding crew duty times and rest requirements to ensure safety. Organizational Culture: The impact of workplace culture on workload management, including communication and teamwork dynamics. - Organizational performance is subject to cultural influences at every level and the organizational culture consists of shared beliefs, practices and attitudes. VIGILANCE Vigilance refers to the state of being watchful, alert, and attentive, especially to potential danger or difficulties. It involves continuously monitoring one's surroundings or a situation in order to detect and respond to changes, threats, or other important events. Vigilance is often associated with situations requiring careful observation and quick reaction, such as in security, safety, or when performing tasks that demand sustained attention. (Matthews G, 2008). Trivia: The term “vigilance” was coined by Sir Henry Head (1923) who used it to refer to a state of maximum physiological and psychological readiness to react. “Clock test” was developed by Norman Mackworth to study the decrement of vigilance overtime, the results showed that the quality of sustained attention is fragile, waning quickly over time. He found that signal detections declined from 85 per cent to 75 per cent within the first 30 minutes on task and continued to drop more gradually for the remainder of the 2-hour vigil. This drop in performance over time is known as the vigilance decrement or the decrement function. Why is it important in the aviation industry? Vigilance is crucial in aviation because it ensures the safety of passengers and smooth flight operations. Pilots, air traffic controllers, and ground crews must stay alert to quickly identify and respond to potential risks, as even small lapses can have serious consequences. Their focus and attention keep flights safe, especially in high-pressure situations.. ` College of Engineering – Department of Electronics Engineering FACTORS AFFECTING VIGILANCE 1. Cognitive Load Description: Cognitive load refers to the mental effort required to process information and perform tasks. It encompasses intrinsic load (difficulty of the material), extraneous load (unrelated distractions), and germane load (effort to understand). Impact: High cognitive load impairs the ability to sustain attention and leads to a decline in vigilance over time. Increased cognitive demands can overwhelm working memory, making it difficult to focus (Sweller, 1988). Citation: Parasuraman, R., Warm, J. S., & See, J. E. (1998). Brain systems of vigilance. In R. Parasuraman (Ed.), The attentive brain (pp. 221–256). Cambridge, MA: MIT Press. 2. Task Complexity Description: Task complexity refers to the number of components and the difficulty involved in performing a task. Complex tasks often involve multitasking or high cognitive demands. Impact: More complex tasks require greater cognitive resources and attention, leading to quicker declines in vigilance, especially under time pressure or stress (Lindholm et al., 2005). Citation: Lindholm, H., Karrberg, P., & Eklund, G. (2005). Task complexity and performance: A multi-dimensional analysis. International Journal of Human-Computer Studies, 63(4-5), 470-487. 3. Fatigue and Sleep Deprivation Description: Fatigue arises from prolonged mental exertion or lack of sleep, affecting cognitive performance. Impact: Sleep deprivation and fatigue significantly reduce reaction times and attention spans, leading to impaired vigilance. Studies have shown that sleep-deprived individuals can exhibit performance deficits comparable to those under alcohol intoxication (Dawson & Reid, 1997). Citation: Dawson, D., & Reid, K. (1997). Fatigue, alcohol, and performance impairment. Nature, 388(6639), 235. 4. Stress and Anxiety Description: Stress and anxiety stem from external pressures or internal worries and can have varying effects on cognitive performance. ` College of Engineering – Department of Electronics Engineering Impact: Moderate levels of stress may enhance vigilance and focus, but chronic or high stress can lead to cognitive overload and impaired attention (Yerkes & Dodson, 1908). Citation: Yerkes, R. M., & Dodson, J. D. (1908). The relation of strength of stimulus to rapidity of habit formation. Journal of Comparative Neurology and Psychology, 18(5), 459-482. 5. Physiological States Description: Physiological states include factors such as hunger, hydration, fatigue, and overall health that can influence cognitive functioning. Impact: Physical discomfort from hunger or dehydration can significantly impair attention and cognitive performance. For instance, studies have shown that even mild dehydration can affect concentration and vigilance (Grandjean & Christensen, 2005). Citation: Grandjean, A., & Christensen, L. (2005). Effects of hydration on cognitive performance. Journal of Nutrition, 135(6), 1532S-1535S. 6. Motivation and Interest Description: Motivation refers to the internal or external drives that influence engagement with a task. Interest is linked to how stimulating or relevant a task is to the individual. Impact: Higher levels of motivation and interest generally correlate with improved vigilance and sustained attention. Conversely, low motivation can lead to disengagement and increased attention lapses (Deci & Ryan, 2000). Citation: Deci, E. L., & Ryan, R. M. (2000). The "what" and "why" of goal pursuits: Human needs and the self-determination of behavior. Psychological Inquiry, 11(4), 227-268. 7. Environmental Factors Description: Environmental factors include external conditions such as noise, lighting, temperature, and overall workspace design that can affect cognitive performance. Impact: Poor environmental conditions, such as excessive noise or inadequate lighting, can significantly hinder the ability to maintain vigilance. Studies indicate that distracting noise can lead to a notable decline in attention (Sundstrom et al., 1994). Citation: Sundstrom, E., Town, J. P., & Rice, R. W. (1994). Office noise, satisfaction, and performance. Environment and Behavior, 26(2), 195-222. 8. Monotony and Repetition ` College of Engineering – Department of Electronics Engineering Description: Monotonous tasks involve repetitive actions with little variation, which can lead to cognitive fatigue and disengagement. Impact: Vigilance tends to degrade more rapidly in monotonous environments due to a lack of mental stimulation. Research shows that tasks with little variation can lead to increased lapses in attention (Mackworth, 1948). Citation: Mackworth, N. H. (1948). The breakdown of vigilance during prolonged visual search. Quarterly Journal of Experimental Psychology, 1(1), 6-21. 9. Duration of Vigilance Task Description: The duration of a vigilance task refers to the length of time an individual must remain attentive to a stimulus or task. Impact: Vigilance typically decreases over time, often exhibiting significant decline after about 30 minutes, known as vigilance decrement. Extended periods of required attention can lead to mental fatigue and decreased performance (Warm et al., 2008). Citation: Warm, J. S., Parasuraman, R., & Matthews, G. (2008). Vigilance requires hard mental work and is cognitively demanding. Human Factors, 50(3), 303-311. CIRCADIAN RHYTHMS Circadian rhythms are 24-hour cycles that are part of the body’s internal clock, running in the background to carry out essential functions and processes. Circadian rhythms help living things respond to changes in their environment, in ways that conserve energy, help them find food, and allow them to grow and heal. Circadian rhythms affect many bodily processes, your mental state, and your behavior. Perhaps the most well-known circadian rhythm is the sleep-wake cycle, which determines how sleepy or alert you feel throughout the day and night. Circadian rhythms influence important functions in the human body, such as: Sleep patterns Hormone release Appetite and digestion Temperature Cognitive function The body’s reaction to stress ` College of Engineering – Department of Electronics Engineering Circadian rhythms are the physical, mental, and behavioral changes an organism experiences over a 24-hour cycle. Light and dark have the biggest influence on circadian rhythms, but food intake, stress, physical activity, social environment, and temperature also affect them. The system that regulates an organism’s innate sense of time and controls circadian rhythms is called a biological clock. It’s composed of proteins encoded by thousands of genes that switch on and off in a specific order. A master clock coordinates all the biological clocks in an organism. The human master clock is a large group of nerve cells that form a structure called the suprachiasmatic nucleus (SCN). Among other functions, the SCN controls production of the hormone melatonin based on the amount of light the eyes receive. In the evening, a person’s master clock tells their brain to make more melatonin, causing sleepiness. The SCN also synchronizes the circadian rhythms in different organs and tissues across the body. For this reason, it times circadian rhythms according to environmental cues known as “zeitgebers,” German for “timekeepers.” Light and darkness are the most important and powerful zeitgebers. Other zeitgebers involved in circadian timing include : Meals Exercise Social interactions Daily routines Stress These zeitgebers trigger the release of hormones in the brain and the delivery of chemical signals to body tissues. Thus, the master clock is able to effectively time vital body functions, such as the conversion of food into energy, fluctuations in body temperature, and when a person feels like sleeping or waking up. According to Dr. Lulu Guo, “An adult’s natural internal clock is on average 24.2 hours. We use external stimuli to help entrain this rhythm daily to 24 hours. Changing the amount and times of sunlight exposure, or changing our routines, can send signals to our ‘master clock’ and shift our natural circadian rhythms.” Health Effects of Disrupted Circadian Rhythms If you don’t sleep when your body tells you that it’s time to—or if you sleep for long periods during the day—your circadian rhythms might become misaligned with the 24-hour cycle of day and night There are many reasons why this might happen, including shift work, travel across time zones, social or study habits that lead to irregular bedtimes, illness, stress, frequent sleep disruptions, or a noisy and bright sleep environment. ` College of Engineering – Department of Electronics Engineering Misalignment between your circadian rhythms and your environment, especially over the long term, can have serious consequences for your health and wellbeing. Sleep problems: Misaligned circadian rhythms can make it harder to fall asleep at bedtime, lead to more wake-ups during the night, and result in less sleep overall. Misalignment can first be confused as insomnia but frequent misalignment can in fact contribute to the development of insomnia. Performance issues: If your circadian rhythms are off, you are likely to experience a range of issues that affect your ability to function, such as excessive sleepiness, difficulty focusing, memory problems, and difficulty performing high-precision tasks. Emotional and social difficulties: Sleep deprivation makes it harder to manage stress and to regulate emotions, so if your circadian rhythms are out of sync, you’re more likely to experience mental health struggles like depression and conflict in your relationships. Accidents and errors: Misaligned circadian rhythms increase your risk of having a car accident, making a serious mistake at work, or getting hurt on the job. Health problems: A range of health problems are associated with out-of-sync circadian rhythms, including obesity, diabetes, heart attacks, high blood pressure, and cancer. Fatigue: Chronic misalignment of circadian rhythms or a lack of a routine to help entrain the internal clock can cause symptoms of low energy and grogginess. “Sleeping at the right times is just as important as the number of hours of sleep.” - Dr. Guo Circadian rhythms can fall out of sync with the outside world due to factors in the human body or environment. For example: Variants of certain genes can affect the proteins that control biological clocks. Neurological diseases, such as Alzheimer’s disease, can disrupt circadian rhythms, causing poor sleep quality and changes in symptoms from day to night. Travel between time zones (jet lag) and shift work alters the normal sleep-wake cycle. Light from electronic devices at night can confuse biological clocks. Drowsiness, poor coordination, and difficulty with learning and focus may occur when circadian rhythms fall out of sync short term. Long-term sleep loss and continually shifting circadian rhythms can increase the risks of obesity, diabetes, mood disorders, heart and blood pressure problems, and cancer, and can also worsen existing health issues. Circadian Rhythm Sleep Disorders There are six sleep disorders that can cause abnormalities in a person’s ability to sleep and wake in expected 24-hour patterns. Some of these disorders are environmental in nature or “extrinsic,” meaning that they happen when a person’s activities or circumstances cause their circadian ` College of Engineering – Department of Electronics Engineering rhythms to desynchronize from their environment. Other disorders are “intrinsic”—that is, they happen when a person’s circadian timekeeping system doesn’t function properly. Shift work disorder: This disorder occurs when shift work prevents a person from sleeping according to their natural circadian rhythms. People with this disorder often struggle to stay awake during night shifts and to sleep at home during the day, which results in sleep deprivation. There is further disruption as many shift workers change sleep schedules throughout the week depending on if they are working or not working. Jet lag disorder: When people travel across multiple time zones, their circadian rhythms won’t initially align with the light-dark cycle in their new environment. This can result in jet lag, which causes daytime sleepiness, digestive problems, and sleep difficulties. Advanced sleep-wake phase disorder: Individuals with this disorder feel sleepy before their desired bedtime and wake up very early in the morning. Sleep debt occurs when people with this disorder force themselves to stay up late, as they are unable to sleep in. Delayed sleep-wake phase disorder: People with this disorder don’t feel ready for bed until very late at night, and they struggle to get up at conventional wake times. They would sometimes feel a “second wind” in the evening and this pattern is frequently labeled as being a “night owl”. This can result in sleep loss for people with work, school, or family obligations in the morning. Non-24-hour sleep-wake rhythm disorder: In people with this disorder, the master clock driving circadian rhythms fails to make the daily corrections that align it with the 24-hour day. Their internal clock may shift by a few minutes or hours every day, making it difficult to maintain a routine and sleep at night or stay awake during the daytime. Irregular sleep-wake rhythm disorder: For indiv