Adaptations To Resistance Training PDF
Document Details
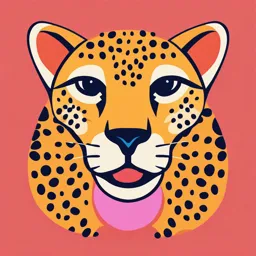
Uploaded by SelfDeterminationTriumph
Tags
Summary
This document provides an overview of adaptations to resistance training, explaining how muscles adapt to exercise. It discusses the neural and physical changes that occur during and after training, including mechanisms such as muscle hypertrophy and neural adaptations. The document is aimed at understanding how resistance training influences muscular strength and fitness.
Full Transcript
05.03.2024 ADAPTATIONS TO RESISTANCE TRAINING D I A N N E L. S A LVA L E O N A D A P TAT I O N S T O R E S I S TA N C E T R A I N I N G HOW DO WE GET STRONGER, INCREASE MUSCLE IMPROVE POWER, &OUR MUSCULAR FITNESS? Muscular fitness is important to general health and athletic performance. Moreso, resi...
05.03.2024 ADAPTATIONS TO RESISTANCE TRAINING D I A N N E L. S A LVA L E O N A D A P TAT I O N S T O R E S I S TA N C E T R A I N I N G HOW DO WE GET STRONGER, INCREASE MUSCLE IMPROVE POWER, &OUR MUSCULAR FITNESS? Muscular fitness is important to general health and athletic performance. Moreso, resistance traiing programs are necessary to increase strength, power, and endurance. In this chapter we will discuss the changes that occur as a result of resistance training- more specifically, improvements in strength. We will explain the mechanisms involved in musclar gains. A D A P TAT I O N S T O R E S I S TA N C E T R A I N I N G ADAPTATIONS changes in structure and function which occur after repeated exposures to bouts of exercise, or training sessions. casuses changes in structure and functions to the cells With chronic exercise, many adaptation occur in the neuromuscular system. A D A P TAT I O N S T O R E S I S TA N C E T R A I N I N G ADAPTATIONS Aerobic Training little or no gains in muscular strength and power Resistance Training major neuromusular adaptations occur Strength and muscular training in the over -all training programs of athletes have gained traction to the sporting community since the 1960s because research has proven that there are performance benefits when athletes undergo resistance training. The extent of these adaptations depend on the type of training program followed. A D A P TAT I O N S T O R E S I S TA N C E T R A I N I N G MECHANISMS OF GAINS IN MUSCULAR STRENGTH For many years, strength gains were assumed to result directly from increases in muscle size. This assumption was logical becuase most who strength trained regularly often developed large, bulky muscles. Also muscles associated with a limp immobilized in a cast for weeks or months start to decrease in size and lose strength immediately. Gains in muscle size are generally paralleled by gains in strength and losses in muscle size correlate highly with losses in stregth. Thus it is tempting to conclude that a direct cause and effect between muscle size and muscle stregnth. While there is an association between size and strength, muscle strength involves far more than that of mere muscle size.. in addition to increased size of the muscle, what explains strength gains with training? There is increasing evidence that the neural control of the trained muscle is also altered, allowing a greater force production from the muscle A D A P TAT I O N S T O R E S I S TA N C E T R A I N I N G MECHANISMS OF GAINS IN MUSCULAR Neural Control of Strength Gains STRENGTH SYNCHRONIZATION AND RECRUITMENT OF ADDITIONAL MOTOR UNITS synchronization improve the rate of force development and capability to exert steady forces INCREASED RATE CODING OF MOTOR UNITS AUTOGENIC INHIBITION as frequency of stimulation of a give motor unit is increased, the muscle eventually reaches a state of tetanus, producing the absolute peak force or tension. may be attenuated with resistance training, allowing a greater force production from trained muscles independent of increases in muscle mass OTHER NEURAL FACTORS *Coactivation of agonist and antagonist muscles *Changes in the morphology of neuromuscular junction might be directly related to the muscle force-producing capacity NEURAL CONTROL of STRENGTH GAINS Enoka, has made a convincing argument that strength gains can be achieved without structural changes in muscle but not without neural adaptations Rather strength gains is a property of the motor system. Motor unit recruitment, stimulation frequency, and other neural factors are also important to strength gains. They may well explain most strength gains that occur in the absence of hypertrophy, as well as episodic superhuman feats of strength SYNCHRONIZATION AND RECRUITMENT OF ADDITIONAL MOTOR UNITS Motor units are generally recruited asynchrounously; they are not all called on at the same instant. They are controlled by a number of different excitatory and inhibitoary impulses. Whether the muscle fibers contract or stay relaxed depends on the summation of the many impulses received by the given motor unit at any one time. The motor unit is activated and its muscle fibers contract only when the incoming excitatory impulses exceed the inhibitory impulses and the threshold is met or exceeded.. Strength gains may result from changes in the connections between motor neurons located in the spinal cord, allowing motor units to act more synchronously, facilitating contraction, and increasing the muscle's ability to generate force. There is good evidence to support increased motor unit synchronouzation with resistance training. An alternate possibility is simply that more motor units are recruited to perform the given task , independent of whether these motor unis act in unision. Such improvement in recruitment patterns could result from an increase in neural drive to the alpha motor neurons during maximal contraction. INCREASED RATE OF CODING OF MOTOR UNITS under the assumption that recruitment there is an increase in neural drive, there is also an increase of rate of coding rate of coding is the rate of action potential discharge to a muscle and could accumulate to produce peak force or tension. as the frequency of stimulation of a given motor unit is increased, the muscle eventually reaches a state of tetanus, producing the absolute peak force or tension. AUTOGENIC INHIBITION Inhibitory mechanisms in the neuromuscular system, such as the Golgi tendon organs by be necessary to prevent the msucles from exerting more force than the bones and connective tissues can tolerate. This is reffered to as autogenic inihibition. However in extreme situations when larger forces are sometime produced, significant damage can occur to these structures, suggesting that the protective inhibitory mechanisms can be overridden. Training can gradually reduce or counteract these inhibitory impulses, allowing the msucles to reach greater levels of stregnth. Thus, strength gains may be achieved by reduced neural inhibition. OTHE NEURAL FACTORS in addtion to increasin motor unit recruitment or decreasing neirological inhibition, other neural factor can contribute to strength gains with resistance training. One of these is referred to as coactivation of agonist and antagonist muscles. to maximize the force generated by an agonist, it is necessary to minimize the coaction. Reduction in coactivation could explain a portion of strength gains attributed in neural factors but its contribution would likely be smalle A D A P TAT I O N S T O R E S I S TA N C E T R A I N I N G MECHANISMS OF GAINS IN MUSCULAR increase in muscle size STRENGTH Muscle Hypertrophy TRANSIENT HYPERTROPHY increased muscle size that develops during and immidiately a single bout of exercise. Size increase due to fluid accumulation in the interstitial and intercellular space CHRONIC HYPERTROPHY increase in muscle size that occurs with long-term resistance training FIBER HYPERTROPHY FIBER HYPERPLASIA Increase in the size of existing individual muscle fibers increase in the number of muscle fibers A D A P TAT I O N S T O R E S I S TA N C E T R A I N I N G F I B E R HYPERTROPHY Muscular cross sectional area changes. A and C are before training, B and D are after training (and doping in this study). SinhaHikim et al., 2002. increase in the size of existing muscle fibers early research suggested that the number of muscle fibers in each of a person’s muscles is fixed. then muscles hypertrophy could only result from indv. muscle hypertrophy ⚬ more myofibrils, ⚬ more actin and myosin filaments, ⚬ more sarcoplasm, ⚬ more connective tissue, or any combination of these. In resistance training, indv. muscle hypertrophy appears to result from a net increase of protein synthesis. testosterone is partly responsible for changes ⚬ primary function: muscle growth ⚬ e.g. males (teenager) experience a 10 fold increase in muscle growth starting puberty The figure shows cross-sections of muscle biopsies obtained before and after 20 weeks of treatment in one man treated with GnRH agonist and 600 mg testosterone enanthate weekly. The left panels represent baseline sections, and the right panels sections obtained after 20 weeks of treatment. The magnification is 200-fold in panels A and B, and 1000-fold in panels C and D. (Reproduced with permission from Sinha-Hikim et al. 2002.) Early research suggested that the number of muscle fibers in each of a person’s muscles was established by birth or shortly thereafter and that this number remained fixed throughout life. If this were true, then whole-muscle hypertrophy could result only from individual muscle fiber hypertrophy. This could be explained by more myofibrils, more actin and myosin filaments, more sarcoplasm, more connective tissue, or any combination of these. *** muscle fibers are composed of myofibrils Hypertrophy is probably caused by increased numbers of myofibrils and actin and myosin filaments, which would provide more cross-bridges for force production during maximal contraction. The size of existing myofibrils does not appear to change. The increase in muscle cross-sectional area results from adding new sarcomeres in parallel to each other. increase in muscle protein synthesis. The muscle’s protein content is in a continual state of flux. Protein is always being synthesized and degraded. But the rates of these processes vary with the demands placed on the body. During exercise, protein synthesis decreases, while protein degradation increases. After exercise, although protein degradation continues, protein synthesis increases three- to fivefold more, leading to a net synthesis of myofibrillar (myosin and actin) protein. A single bout of resistance exercise can elevate net protein synthesis for up to 24h. A D A P TAT I O N S T O R E S I S TA N C E T R A I N I N G F I B E R increase in the number of muscle fibers HYPERPLASIA muscle fiber hyperplasia can occur in animals and possibly in humans. ⚬ studies on muscle hyperplasia in humans show that muscle hyperplasia is possible on certain individuals or under certain training conditions. ■ usually intense resistance training The cats were trained with a pure form of resistance training: high resistance and low repetitions. The other animals were trained with more endurance-type activity: low resistance and high repetitions. A D A P TAT I O N S T O R E S I S TA N C E T R A I N I N G INTEGRATION OF NEURAL ACTIVATION AND FIBER HYPERTROPHY Early increases in voluntary strength or maximal force production are associated primarily with neural adaptations (first 8-10 weeks of training) ⚬ protein build up takes time through protein degradation v.v. protein synthesis ⚬ strength improvement due to changes in the pattern by which nerved activate the muscle fibers Long-term increases in strength generally result from hypertrophy of the trained muscle ⚬ hypertrophy contributes progressively and becomes a major contributor after 10 weeks of training Research on resistance training adaptations indicates that early increases in voluntary strength, or maximal force production, are associated primarily with neural adaptations resulting in increased voluntary activation of muscle. This was clearly demonstrated in a study of both men and women who participated in an 8-week, high-intensity resistance training program, training twice per week. Muscle biopsies were obtained at the beginning of the study and every 2 weeks during the training period. Strength, measured according to the 1RM, increased substantially over the 8 weeks of training, with the greatest gains coming after the second week. Muscle biopsies, however, revealed only a small, insignificant increase in muscle fiber cross-sectional area by the end of the 8 weeks of training. Thus, the strength gains were largely the result of increased neural activation. Long-term increases in strength generally result from hypertrophy of the trained muscle. However, because it takes time to build protein through a decrease in protein degradation, an increase in protein synthesis, or both, early strength gains are typically due to changes in the pattern by which nerves activate the muscle fibers. Most research shows that neural factors contribute prominently to strength gains during the first 8 to 10 weeks of training. Hypertrophy contributes little during these initial weeks of training but progressively increases its contribution, becoming the major contributor after 10 weeks of training. However, not all studies concur with this pattern of strength development. One 6month study of strength-trained athletes showed that neural activation explained most of the strength gains during the most intensive training months and that hypertrophy was not a major factor. A D A P TAT I O N S T O R E S I S TA N C E T R A I N I N G MUSCLE ATROPHY AND DECREASED STRENTGH wasting away or decrease in the size of the muscle WITH INACTIVITY Muscle Atrophy tissue results from lack of muscle use and the loss of muscle protein that accompanies the inactivity IMMOBILIZATION first 6h of immobilization, the rate of protein synthesis decreases strength decrease ave. 3%-4% per day ⚬ associated w/ decreased neuromuscular activity affects both type I and Type II fibers cross-sectional fiber area decreases; effect is greater in type I fibers muscles can recover but longer than the period of immobilization Muscle Atrophy and Decreased Strength With Inactivity When a normally active or highly trained person reduces his or her level of activity or ceases training altogether, changes occur in both muscle structure and function. This is illustrated by the results of two types of studies: studies in which entire limbs have been immobilized and studies in which highly trained people stop training—so-called detraining. Immobilization When a trained muscle suddenly becomes inactive through immobilization, major changes are initiated within that muscle in a matter of hours. During the first 6 h of immobilization, the rate of protein synthesis starts to decrease. This decrease likely initiates muscular atrophy, which is the wasting away or decrease in the size of muscle tissue. Atrophy results from lack of muscle use and the consequent loss of muscle protein that accompanies the inactivity. Strength decreases are most dramatic during the first week of immobilization, averaging 3% to 4% per day. This is associated with the atrophy but also with decreased neuromuscular activity of the immobilized muscle. Immobilization appears to affect both type I and type II fibers. From various studies, researchers have observed disintegrated myofibrils, streaming Z-disks (discontinuity of Zdisks and fusion of the myofibrils), and mitochondrial damage. When muscle atrophies, the cross-sectional fiber area decreases. Several studies have shown the effect to be greater in type I fibers, including a decrease in the percentage of type I fibers, thereby increasing the relative percentage of type II fibers. Muscles can and often do recover from immobilization when activity is resumed. The recovery period is substantially longer than the period of immobilization. See chapter 14 for more on the effects of muscle immobilization. A D A P TAT I O N S T O R E S I S TA N C E T R A I N I N G MUSCLE ATROPHY AND DECREASED STRENTGH WITH INACTIVITY Muscle Atrophy CESSATION OF TRAINING Bosquet, L., Berryman N., Dupuy, O., et. al (2013) Decreases all components of muscular strength Submaximal strength; Maximal power decreases with cessation of resistance training. Effect was found to be larger in older people (>65 y.o). Effect was larger in inactive people when compared with recreational athletes (for maximal force and maximal power) Magnitude of effect differs to training status, age or duration of training cessation. Effect of training cessation on muscular performance: a meta-analysis L Bosquet 1, N Berryman, O Dupuy, S Mekary, D Arvisais, L Bherer, I Mujika Affiliations expand PMID: 23347054 DOI: 10.1111/sms.12047 Abstract The purpose of this study was to assess the effect of resistance training cessation on strength performance through a meta-analysis. Seven databases were searched from which 103 of 284 potential studies met inclusion criteria. Training status, sex, age, and the duration of training cessation were used as moderators. Standardized mean difference (SMD) in muscular performance was calculated and weighted by the inverse of variance to calculate an overall effect and its 95% confidence interval (CI). Results indicated a detrimental effect of resistance training cessation on all components of muscular performance: [submaximal strength; SMD (95% CI) = -0.62 (-0.80 to -0.45), P < 0.01], [maximal force; SMD (95% CI) = -0.46 (-0.54 to -0.37), P < 0.01], [maximal power; SMD (95% CI) = -0.20 (-0.28 to -0.13), P < 0.01]. A dose-response relationship between the amplitude of SMD and the duration of training cessation was identified. The effect of resistance training cessation was found to be larger in older people (> 65 years old). The effect was also larger in inactive people for maximal force and maximal power when compared with recreational athletes. Resistance training cessation decreases all components of muscular strength. The magnitude of the effect differs according to training status, age or the duration of training cessation. A D A P TAT I O N S T O R E S I S TA N C E T R A I N I N G FIBER TYPE Can muscle fibers change from one type to another through resistance ALTERATION training? possible through cross-innervation ⚬ fibers began to take on certain characteristics of the opposite fiber type if the training was of the opposite kind (e.g., type II fibers might become more oxidative with aerobic training) possible through chronic, low-frequency nerve stimulation ; transforms type II motor units to type I motor units (animal models) Staron and coworkers found evidence of fiber type transformation in women as a result of heavy resistance training ⚬ mean percentage of type IIx fibers decreased significantly, but the mean percentage of type IIa fibers increased other studies demonstrate that a combination of high intensity resistance training and short-interval speed work can lead to a conversion of type I to type IIa fibers. Can muscle fibers change from one type to another through resistance training? The earliest research concluded that neither speed (anaerobic) nor endurance (aerobic) training could alter the basic fiber type, specifically from type I to type II or from type II to type I. CROSS-INNERVATION: typeII motor unit is experimentally innexvated by a type I motor neuron or a type I motor unit is innervated by type II motor unit. These early studies did show, however, that fibers began to take on certain characteristics of the opposite fiber type if the training was of the opposite kind (e.g., type II fibers might become more oxidative with aerobic training). Research using animal models has shown that fiber type conversion is indeed possible under conditions of cross-innervation, in which a type II motor unit is experimentally innervated by a type I motor neuron or a type I motor unit is experimentally innervated by a type II motor neuron. Also, chronic, low-frequency nerve stimulation transforms type II motor units into type I motor units within a matter of weeks. Muscle fiber types in rats have changed in response to 15 weeks of high-intensity treadmill training, resulting in an increase in type I and type IIa fibers and a decrease in type IIx fibers. The transition of fibers from type IIx to type IIa and from type IIa to type I was confirmed by several different histochemical techniques. Staron and coworkers found evidence of fiber type transformation in women as a result of heavy resistance training. Substantial increases in static strength and in the crosssectional area of all fiber types were noted following a 20-week heavy resistance training program for the lower extremity. The mean percentage of type IIx fibers decreased significantly, but the mean percentage of type IIa fibers increased. The transition of type IIx fibers to type IIa fibers with resistance training has been consistently reported in a number of subsequent studies. Further, other studies demonstrate that a combination of high intensity resistance training and short-interval speed work can lead to a conversion of type I to type IIa fibers. A D A P TAT I O N S T O R E S I S TA N C E T R A I N I N G IN REVIEW Neural adaptations always accompany the strength gains that result from resistance training, but hypertrophy may or may not take place. Neural mechanisms leading to strength gains can include an increase in frequency of stimulation, or rate coding; recruitment of more motor units; more synchronous recruitment of motor units; and decreases in autogenic inhibition from the Golgi tendon organs. Early gains in strength appear to result more from changes in neural factors, but later long-term gains are largely the result of muscle hypertrophy. Transient muscle hypertrophy is the temporary enlargement of muscle resulting from edema immediately after an exercise bout. Chronic muscle hypertrophy occurs from repeated resistance training and reflects actual structural changes in the muscle. Most muscle hypertrophy results from an increase in the size of individual muscle fibers (fiber hypertrophy). Fiber hypertrophy increases the numbers of myofibrils and actin and myosin filaments, which provides more cross-bridges for force production. Muscle fiber hyperplasia has been clearly shown to occur in animal models with the use of resistance training to induce muscle hypertrophy. Only a few studies suggest evidence of hyperplasia in humans. Muscles atrophy (decrease in size and strength) when they become inactive, as with injury, immobilization, or cessation of training. Atrophy begins very quickly if training is stopped; but training can be reduced, as in a maintenance program, without resulting in atrophy or loss of strength. With resistance training there is a transition of type IIx to type IIa fibers. Evidence indicates that one fiber type can actually be converted to the other type (e.g., type I to type II, or vice versa) as a result of cross-innervation or chronic stimulation, and possibly with training. Neural adaptations always accompany the strength gains that result from resistance training, but hypertrophy may or may not take place Neural mechanisms leading to strength gains can include an increase in frequency of stimulation, or rate coding; recruitment of more motor units; more synchronous recruitment of motor units; and decreases in autogenic inhibition from the Golgi tendon organs. Most muscle hypertrophy results from an increase in the size of individual muscle fibers (fiber hypertrophy). Muscles atrophy (decrease in size and strength) when they become inactive, as with injury, immobilization, or cessation of training. With resistance training there is a transition of type IIx to type IIa fibers.