Globular Proteins Lecture Notes PDF
Document Details
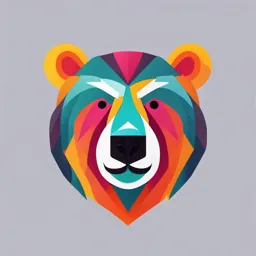
Uploaded by TerrificCerberus
Suez Canal University
Noha M. Mesbah
Tags
Summary
These lecture notes detail the structures and functions of globular proteins, with a specific focus on hemeproteins like hemoglobin and myoglobin. The notes discuss oxygen binding and transport, as well as various factors affecting these processes.
Full Transcript
Globular Proteins Noha M. Mesbah Department of Biochemistry Faculty of Pharmacy, Suez Canal University Globular Hemeproteins Proteins with heme as a prosthetic group (prosthetic group is a non-protein molecule associated with protein and necessary for its activi...
Globular Proteins Noha M. Mesbah Department of Biochemistry Faculty of Pharmacy, Suez Canal University Globular Hemeproteins Proteins with heme as a prosthetic group (prosthetic group is a non-protein molecule associated with protein and necessary for its activity) Heme group in proteins can have different functions: – Hemoglobin / myoglobin: reversible binding of oxygen – Catalase: involved in breakdown of hydrogen peroxide – Cytochrome: electron carrier Structure of Heme Complex of protoporphyrin IX and ferrous iron (Fe2+ ) Iron can form six bonds: 4 with nitrogens of porphyrin ring 1 with a histidine residue in the globin protein 1 binds oxygen Structure and Function of Myoglobin Present in heart and skeletal muscle Functions as: – Reservoir for oxygen – Oxygen carrier, increases rate of oxygen transport in muscle cells Composed of a single polypeptide chain (no quaternary structure) Structure of Myoglobin 1. α-helical content: 80% of the polypeptide chain folded into eight α- helices α-helices labeled A to H α-helices terminated by proline and connected by β-bends and loops stabilized by hydrogen and ionic bonds Structure of Myoglobin 2. Location of polar and nonpolar amino acids: Interior of myoglobin molecule composed of nonpolar amino acids Interior stabilized by hydrophobic interactions Surface of molecule composed of charged amino acids Charged amino acids on surface form hydrogen bonds with each other and surrounding water Structure of Myoglobin 3. Binding of heme: Sits in the center of the molecule, lined with nonpolar amino acids Two histidine residues: F8 proximal histidine binds iron of heme Distal histidine (E7), Protein of myoglobin facilitates stabilizes binding of oxygen to ferrous ion the reversible binding of oxygen Structure and Function of Hemoglobin Present only in red blood cells Functions to transport oxygen from lungs to capillaries of all tissues Hemoglobin A is composed of 4 polypeptide chains (two α-chains, two β-chains) Polypeptide chains held together by noncovalent interactions Structure of Hemoglobin Four polypeptide chains form 4 subunits, hemoglobin is a tetrameric protein Each subunit has stretches of α-helices Heme binding site similar to that of myoglobin, with two histidine molecules (4 heme binding sites, one in each subunit) Properties of Hemoglobin Hemoglobin can transport H+ and CO2 from tissues to lungs (myoglobin transports only O2) Hemoglobin can carry 4 molecules of oxygen from the lungs to tissues in the body (myoglobin only carries one oxygen molecule) Oxygen-binding properties of hemoglobin regulated by interaction with allosteric effectors Structure of Hemoglobin Quaternary Structure of Hemoglobin Hemoglobin is a tetramer, with 4 subunits Hemoglobin is often viewed as having two identical dimers, (αβ)1 and (αβ)2 Each dimer contains 2 polypeptide chains Polypeptide chains within dimers held together primarily by hydrophobic interactions Two dimers are held together by polar bonds Structure of Hemoglobin Quaternary Structure of Hemoglobin Interactions holding the two dimers together are weaker than the interactions within each dimer Both dimers are able to move with respect to each other Both dimers occupy different relative positions in deoxyhemoglobin compared with oxyhemoglobin Structure of Hemoglobin Quaternary Structure of Hemoglobin Two forms of hemoglobin: 1. T-form: – Taut or tense form – Deoxy form – Low-oxygen-affinity form – Ionic and hydrogen bonds between the two dimers constrain movement of polypeptide chains 2. R-form: – Relaxed form – Oxygenated form – Binding of oxygen ruptures some ionic and hydrogen bonds between the two dimers, polypeptides have freedom of movement – High-oxygen-affinity form Structure of Hemoglobin Quaternary Structure of Hemoglobin Binding of Oxygen to Hemoglobin and Myoglobin Myoglobin binds one oxygen molecule Hemoglobin binds 4 oxygen molecules Y: degree of saturation of oxygen-binding sites Oxygen dissociation curve: plot of Y at different partial pressures of oxygen (pO2) Binding of Oxygen to Myoglobin and Hemoglobin Myoglobin has higher oxygen affinity than hemoglobin Myoglobin binds oxygen and becomes saturated at lower oxygen concentrations Oxygen dissociation curve for myoglobin is hyperbolic Binding of Oxygen to Myoglobin and Hemoglobin Hemoglobin has a lower affinity for oxygen Hemoglobin becomes saturated at higher concentrations of oxygen Oxygen dissociation curve for hemoglobin is sigmoidal; subunits cooperate to bind oxygen Binding of Oxygen to Myoglobin and Hemoglobin Cooperativity in oxygen binding in hemoglobin: Binding of oxygen at one heme group increases affinity of remaining heme groups in the same hemoglobin molecule Also called heme-heme interaction Binding of first oxygen is difficult, binding of remaining oxygens occurs with high affinity Differences between Hemoglobin and Myoglobin Hemoglobin Myoglobin 4 polypeptides, tetramer 1 polypeptide, monomer (quaternary structure) (tertiary structure only) Binds 4 molecules of Binds one molecule of oxygen with lower affinity oxygen with higher affinity Sigmoidal oxygen Hyperbolic oxygen dissociation curve dissociation curve Functions to transport Functions to store oxygen oxygen from lungs to in skeletal muscle and tissues heart Factors Affecting Binding of Oxygen by Hemoglobin Oxygen binding by hemoglobin is affected by: 1. Oxygen concentration pO2 2. pH 3. Carbon dioxide concentration pCO2 4. 2,3-bisphosphoglycerate Allosteric effectors “other site effectors”: interact with hemoglobin molecule at a site different than the oxygen binding site Oxygen binding to myoglobin is not affected by allosteric effectors 1. Effect of pO2 Cooperative binding of oxygen, affinity to last oxygen bound is 300 times higher than affinity to first oxygen bound Sigmoidal oxygen dissociation curve permits effective release of oxygen to tissues 2. Effect of pH and the Bohr Effect: Acid pH decreases oxygen affinity of hemoglobin Oxygen release is enhanced at lower pH Oxygen dissociation curve shifts to the right Increase in pH increases affinity for oxygen, shifts dissociation curve to the left Factors Affecting Binding of Oxygen by Hemoglobin 3. Effect of pCO2 (Bohr effect) Increase pCO2 causes decrease in oxygen affinity of hemoglobin Oxygen release from hemoglobin is enhanced Oxygen dissociation curve shifts to the right Decrease in CO2 concentration causes greater oxygen affinity, dissociation curve shifts to left Source of Protons Producing the Bohr Effect Metabolically active peripheral tissues have higher concentrations of CO2 and H+ than the lungs CO2 in tissues is converted to carbonic acid: CO2 + H2O ↔ H2CO3 Carbonic acid spontaneously deprotonates: H2CO3 ↔ HCO3- + H+ H+ released lowers the pH of tissues (it becomes less than pH in lungs) pH difference between lungs and tissues favors loading of hemoglobin with oxygen in lungs and unloading of oxygen in tissues Mechanism of the Bohr Effect Deoxyhemoglobin has greater affinity to protons than oxyhemoglobin Increased H+ concentration causes N-terminal α- amino groups to become protonated Charged groups form ionic bonds and stabilize deoxy form of hemoglobin HbO2 + H+ ↔ HBH + O2 Increase in protons shifts equilibrium to right Increase in oxygen shifts equilibrium to the left Factors Affecting Binding of Oxygen by Hemoglobin 4. Effect of 2,3-bisphosphoglycerate on oxygen affinity: Also called 2,3-BPG Most abundant organic phosphate in RBC’s Decreases oxygen affinity of hemoglobin by binding to deoxyhemoglobin, stabilizes the T- form 4. Effect of 2,3- bisphosphoglycerate on oxygen affinity: One molecule of 2,3-BPG binds to a pocket in the center of the deoxyhemoglobin tetramer Pocket contains positively charged amino acids which bind to the negatively charged phosphate groups 2,3-BPG is expelled from the tetramer on oxygenation Factors Affecting Binding of Oxygen by Hemoglobin 4. Effect of 2,3-bisphosphoglycerate on oxygen affinity: Concentration of 2,3-BPG in red blood cells increases in response to chronic hypoxia: – Obstructive pulmonary emphysema – High altitudes – Chronic anemia 4. Effect of 2,3- bisphosphoglycerate on oxygen affinity: During hypoxia, hemoglobin does not receive enough oxygen Increase in the level of 2,3-BPG decreases the oxygen affinity of hemoglobin, allows greater unloading of oxygen to the tissues Role of 2,3-BPG in Transfused Blood 2,3-BPG is essential for normal oxygen transport by hemoglobin Chemicals used to preserve blood e.g. acid- citrate-dextrose, cause decrease in 2,3-BPG in blood Blood has very high affinity for oxygen, does not release oxygen to tissues “oxygen trap” Solution: add inosine (hypoxanthine-ribose). It releases ribose, ribose enters hexose- monophosphate pathway which produces 2,3- BPG Binding of CO2 to Hemoglobin Most metabolic CO2 is hydrated and transported as bicarbonate Some CO2 is carried as carbamate bound to uncharged α-amino groups of hemoglobin, forming carbamino-hemoglobin Hb-NH2+CO2↔ Hb-NH-COO-+H+ CO2 binding stabilizes the T-form (deoxy form) of hemoglobin, causes decrease in oxygen affinity Allows dissociation of CO2 from hemoglobin in the lungs Binding of Carbon Monoxide (CO) to Hemoglobin CO binds to iron of hemoglobin (reversible), forms carbon monoxyhemoglobin Binding of CO to a site on heme increases the affinity of other oxygen binding sites, oxygen dissociation curve moves to left Affected hemoglobin is not able to unload oxygen to tissues Affinity of hemoglobin for CO is 220 times greater than affinity for oxygen, CO is very toxic CO poisoning is treated with 100 % oxygen, facilitates dissociation of CO from hemoglobin Minor Hemoglobins Fetal Hemoglobin HbF Major hemoglobin in the fetus and newborn Higher affinity for oxygen than HbA Binds weakly to 2,3-BPG Facilitates transfer of oxygen from maternal circulation across placenta to red blood cells of the fetus Hemoglobin HbA1c HbA is slowly glycosylated under physiological conditions Extent of glycosylation depends on plasma concentration of hexose HbA1c has glucose residues attached to amino groups of N-terminal valines Increased amounts of HbA1c found in blood of diabetic patients Fibrous Proteins Fibrous Proteins Fibrous proteins have structural functions: – Skin – Connective tissue – Blood vessel walls – Cornea Examples of fibrous proteins include collagen and elastin Collagen Most abundant protein in the human body Three polypeptide chains wound around each other (α-chains) Collagen has a structural role: – Eye: supports vitreous humor – Tendons: tight fibers provide strength Types of Collagen Type Tissue Distribution Fibril-forming I Skin, bone, tendon, blood vessels, cornea II Cartilage, intervertebral Long, stiff rope-like structures disk, vitreous body III Blood vessels, fetal skin Network forming IV Basement membrane VII Beneath stratified Assemble into sheets squamous epithelia Fibril-associated IX Cartilage Link collage fibrils with XII Tendon, ligaments components in extracellular matrix Structure of Collagen 1. Amino acid sequence: Collagen has repeating sequence: -Gly – X – Y – Gly – X – Y – Gly- Glycine present in every third position of polypeptide Proline at position X Proline essential for helical conformation of each α-chain (α-chain cannot be an α-helix due to presence of proline) Y is hydroxyproline or hydroxylysine Structure of Collagen 2. Triple-helical structure: Collagen composed of three polypeptide chains intertwined to form a triple-stranded helix Elongated structure (not globular, compact structure) Most amino acid side chains are exposed on the surface, this allows bond formation between α- chains and collagen monomers (hydrophobic interactions are not involved in stabilization) Structure of Collagen 3. Hydroxyproline and hydroxylysine: Hyp and Hyl are not present in most proteins Pro and lys residues are hydroxylated after incorporation into polypeptide chains Hyp stabilizes triple-helical structure of collagen because it maximizes interchain hydrogen bond formation Hydroxylation requires vitamin C (ascorbic acid) Vitamin C deficiency causes scurvy: decreases strength of collagen fibrils causes capillary fragility and excessive bruising Structure of Collagen 4. Glycosylation Hydroxyl group of Hyl can be glycosylated enzymatically Glucose and galactose are added sequentially to polypeptide chain prior to triple-helix formation Collagen Diseases 1. Ehlers-Danlos syndrome: Occurs due to mutations in the gene needed for production of type III collagen Serious vascular problems occur (due to vascular weakness) Patients also have defects in collagen type I, resulting in stretchy, fragile skin Collagen Diseases 2. Osteogenesis imperfecta: Called brittle bone syndrome Occurs due to mutations in the genes for synthesis of type I collagen Type I collagen cannot form the triple-helical conformation Bones easily bend and fracture Elastin Connective tissue protein with rubber-like properties Present in lungs, walls of large arteries, elastic ligaments Can be stretched to several times their length Structure of Elastin Insoluble protein polymer Precursor: tropoelastin Linear polypeptide Amino acids small and non-polar: Gly, Ala, Val Elastin rich in Pro and Lys, very little Hyp, and NO Hyl Tropoelastin is secreted, then interacts with microfibrils (e.g. fibrillin) Structure of Elastin Elastin is an extensively interconnected network Elastin can stretch and bend in any direction when stressed Marfan syndrome: mutations in the fibrillin protein, impaired structural integrity of the skeleton, eye and cardiovascular system Degradation of Elastin α1-Antitrypsin: – Also called α1-antiproteinase – Inhibits proteases (or proteolytic enzymes) – Synthesized by the liver – Present in plasma in large amounts – Inhibits neutrophil elastase, a protease that degrades elastin in the alveolar walls of the lungs Degradation of Elastin Role of α1-antitrypsin in the lungs: – Alveoli are exposed to neutrophil elastase secreted by degenerated neutrophils – Neutrophil elastase degrades elastin in alveolar walls – α1-antitrypsin inhibits the action of neutrophil elastase Degradation of Elastin Emphysema and α1-antitrypsin deficiency: – Mutation in the gene for α1-antitrypsin (substitution of Lys for Glu) results in deficiency of α1-antitrypsin – Alveoli are not protected from neutrophil elastase, results in emphysema Degradation of Elastin