Module 5: Actuators PDF
Document Details
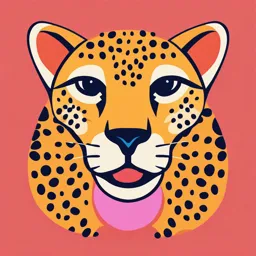
Uploaded by InnocuousForsythia
Vellore Institute of Technology
Dr. R.BHARANIDARAN
Tags
Summary
This document provides an introduction to actuators, focusing on their role in mechatronic systems and various types of actuators. The document also describes electromagnetic principles, relays, and different motor types.
Full Transcript
BMEE210L - Mechatronics and Measurement Systems Module – 5: Actuators Dr. R.BHARANIDARAN Professor, School of Mechanical Engineering, Vellore Institute of Technology, Vellore, India Actuators Phy...
BMEE210L - Mechatronics and Measurement Systems Module – 5: Actuators Dr. R.BHARANIDARAN Professor, School of Mechanical Engineering, Vellore Institute of Technology, Vellore, India Actuators Physically, a mechatronic system is composed of four prime components. They are sensors, actuators, controllers and mechanical components. Figure shows a schematic diagram of a mechatronic system integrated with all the above components. Translational Motion Rotational Actuators Electrical Electromechanical Energy Electromagnetic Hydraulic Actuators are the devices used to produce this motion or action. Pneumatic Electrical Actuators An actuator obtaining electrical energy from the mechanical system is called electric actuators. Electric actuators are generally referred to ad being those where an electric motor drives the robot links through some mechanical transmission i.e., gears. Electrical actuators comprise the following : Drive system: DC motor, AC motor, Stepper motor Switching Device: – Mechanical switch: Solenoids, Relays – Solid-state switch: Diodes, Thyristor, Transistors Electromagnetic principles When a current carrying conductor is moved in a magnetic field, a force is produced in a direction perpendicular to the current and magnetic field directions. Lorentz’s force law, which relates force on a conductor to the current in the conductor and the external magnetic field, in vector form is Electromagnetic Principles When a DC current passes through a long straight conductor a magnetizing force and a static magnetic field is developed around it. The magnetic flux developed around the coil being proportional to the amount of current flowing in the coil's windings. Michael Faraday’s law of electromagnetic induction states: “that a voltage is induced in a circuit whenever relative motion exists between a conductor and a magnetic field and that the magnitude of this voltage is proportional to the rate of change of the flux”. Faraday’s Law Equation Solenoids Solenoids Solenoids Solenoids Voice Coil actuator Voice Coil Actuator Solenoid Force Low to medium High Stroke 5 inches maximum ¼ inch maximum Constant Force Yes No Reversible Yes No Position/Force Yes No Control Cost Moderate Low Electromagnetic Relays Relays are electrical switches that open or close another circuit under certain conditions Isolate controlling circuit from controlled circuit. Control high voltage system with low voltage. Control high current system with low current. Logic Function Relay Operation Relays Electromechanical Relay Type of relay which function using a magnetic field produced by an electromagnetic coil when a control signal is applied to it. It has moving contacts in the output circuit which are operated by applying an electrical signal. Classification of EMRs based on their applications General Purpose Relays – Such as miniature relays, latching relays, timer relays, contactors, machine tool relays, hybrid relays, smart relays, signal relays, automobile relays and PCB relays etc. Protection Relays – Such as thermal overload relays, earth fault relays, under or over voltage relays, under or over current relays, buchholz relay, differential relays, distance protection relays, sequence protection relays, electronic relays etc. Classification of EMRs based on contact configuration Single-Pole Single-Throw (SPST) Single-Pole Double-Throw (SPDT) Double-Pole single-Throw (DPST) Double-Pole Double-Throw (DPDT) Classification of EMRs based on their construction Electromagnetic Attraction Type - Attraction armature type EMR, solenoid type EMR, balanced beam type EMR. Electromagnetic Induction Type - shaded pole type EMR, watt-hour meter type EMR, induction cup type EMR. Motor Principle- Force on Current Carrying Wire in Magnetic Field Electric Motors Configuration classification of electric motors Motor construction and terminology Electric machines can be classified in terms of their energy conversion characteristics. ❑Generators convert mechanical energy from a prime mover (e.g., an internal combustion engine) to electrical form. Examples of generators are those used in power-generating plants, or automotive alternator. ❑Motors convert electrical energy to mechanical form. Electric motors provide forces and torques to generate motion in countless industrial applications. For Example Machine tools, robots, punches, presses, mills, and propulsion systems for electric vehicles are but a few examples of the application of electric machines in engineering. Distinction can be made between different types of windings characterized by the nature of the current they carry. ❖ If the current serves the purpose of providing a magnetic field and is independent of the load, (it is called a magnetizing, or excitation, current) the winding is termed a field winding. (nearly always DC and are of relatively low power, since their only purpose is to magnetize the core). ❖ However, if the winding carries only the load current, it is called an armature. In DC and AC synchronous machines, separate windings exist to carry field and armature currents. A Motor/Generator are made of ❖ Stator: This is the stationary part Separated by an air gap ❖ Rotor: This is the rotating part ❑ The rotor and stator each consist of ❖ Magnetic core, ❖ Electrical insulation, and ❖ Windings necessary to establish a magnetic flux (unless this is created by a permanent magnet). ❑ The rotor is mounted on a bearing-supported shaft, which can be connected to: ❖ Mechanical loads (functioning as a motor), or ❖ A prime mover (functioning as a generator) by means of belts, pulleys, chains, or other mechanical couplings. ❑ The windings carry electric currents that generate magnetic fields (by virtue of Faraday’s law) + + + = Rotor: Commutator Brushes Stator DC Machine Armature Mechanical Electrical Produces an conductor are rectifier connector external flux connected to the converts ac to between Commutator dc armature and power Made of copper segment Pressure is insulated by adjusted using mica the spring ❑ When a current carrying conductor is placed in a magnetic field, the conductor experience a mechanical force. ❑ Direction is given by Flemings left hand rule ( F- B; S-I; T- M) ❑ Magnitude is F=B.I.L Consider a motor with one pair of poles, an armature with a single Explanation conductor coil and a commutator with only two segments, If is field current supplied to the field winding to establish the main field between the poles N and S. Ia is armature current via the carbon brushes. This current produces magnetic fields around the armature conductors Magnitude is F=BIL F F Magnetic field due to Stator and Filed Stator and Filed Magnetic interaction ❑ As the coil rotates an emf is induced in each conductor which opposes the externally supplied armature current, Ia. ❑ The external supply must overcome this emf if the machine is to continue motoring and deliver mechanical power through its shaft. ❑ Faraday’s Law states that the emf induced in a conductor = rate of change of flux linkages ❑ Taken over a period of time Average emf induced in conductor = total flux linkage total time of linkage So, When conductor 1 is close to N-pole: Total flux emanating from that pole = φ Average emf induced in conductor1 = total flux linkage = = 2nφ Volts total time of linkage 1 Therefore, if the coil rotating at n rev sec-1 2n Each conductor will be close to a particular pole 2n times per rotation 37 1 Each conductor will link with its magnetic flux for sec per rotation 2n ❑ Number of poles affects the induced emf ❑ Machines have several pairs of poles. ❑ For a machine with p pole pairs, the average emf in each conductor is given by: φ ❑ average emf induced = total flux per pole = = 2pnφ Volts 1 1 in a conductor total time conductor is under a pole p 2n Total emf induced = average emf induced number of armatur in armature winding in one conductor conductors in series E = 2pn As The number of poles (2p) and the number of armature conductors in series (As) are constant for a particular machine. Therefore k = 2p As E = kn Volts k Since the angular velocity, = 2n E= φω Volts 2π Electrical power delivered = Armature emf Armature current to the armature Pa = E Ia This power creates the torque to make the armature rotate. Electrical torque developed = Electrical power delivered to the armature in the armature Angular velocity Remember: Power is the rate at which work is done; Work done in 1 s = force × distance Power = work done / time taken Pa k 1 k Te = = φ ω Ia = φ Ia Newton meters ω 2π ω 2π Mechanical torque|at the shaft = Electrical torque - “Lost” torque|due to frictional and other losses The “lost” torque is small and will be ignored ❑ The figure represents an equivalent circuit of an armature ❑ E is the induced emf Ia ❑ Ra is the armature resistance Ra ❑ The armature terminal voltage is given by: Va Va = E + IaRa E The field and armature windings may be connected to: ❖ Independent supplies - separately excited ❖ Common supply - self excited Shunt wound: The field and armature windings are connected in parallel Series wound: The field and armature windings are in series Compound wound: Has two field windings; o One connected parallel with the armature and o Other in series with the armature Field Winding: iN If Nf φ= = S S Where, S is the reluctance, N is the number of turns in the coil and i is the coil current. Armature Winding: Armature terminal voltage, V = E + IaRa V = kn + IaRa V : External supply voltage are normally constant, hence keeping If and Rf : field winding resistance hence constant. with constant, let K1 = k V = K1n + IaRa V − Ia Ra The steady state speed = n = K1 speed, n V/K1 V − Ia Ra From Last Slide n= K1 armature current, Ia torque, T V − Ia Ra n= (from last slide) K1 k Using Te = φ Ia 2π kφ With φ constant, K2 = 2π armature current, Ia k T = K 2 Ia or T= φ Ia 2π V − Ia Ra We had: n= K1 V − K 1n Ia = Ra K2 T= [V - K 1n] Ra The torque-speed curve shows that shunt motors can be used to drive fairly constant speed from no load to full torque Therefore, ideal for use with machine tools, pumps, compressors etc. k (Vs/rev) 6 4 2 Field current (A) 0.0 0.5 1.0 1.5 ❑ A 220V dc shunt motor has an armature resistance of 0.8 and field winding resistance of 220. The motor field characteristic [k versus field current] is shown in Figure a) Calculate the field current If the motor drives a constant load torque of 17.5Nm, calculate b) armature current c) speed 220 − 16 n = 5.5 Speed = 37.1 rev sec-1 = 2225 rev min-1. I Induced armature emf, E Rf field winding V Nf turns V Ra armature winding E Current, I In the series motor current, I flows through both field and armature windings so: V = E + I(Ra + Rf) let R = Ra + Rf V = E + IR E = V - IR I Rf field All dc motors, flux, field winding current Nf turns winding V For Series wound motor I; = K3I Ra N.B. this assumption only applies for low currents. armature winding E E = kn E = kK3In E = K4In where K4 = kK3 steady state speed, n V = K4.I.n + I.R 1 V n= I − R K4 current, I torque, T torque, T 1 V n= − R steady state K 4 I speed, n current, I kI a kK 3 T= = I.I 2 2 kK 3 T = K 5I 2 where K 5 = 2 ❑ The series motor is a variable speed machine V ideally suited to drive permanently coupled loads. I= K 4n + R ❑ They are often used for electric traction and lifts. V 2 They must never be used on “no load” as the speed T = K5 will become dangerously high. K 4n + R A 220V dc series motor has armature and field resistances of 0.2 and 0.5 respectively. When running at 1000 rev min-1 the motor draws 10A from the supply. Calculate the torque delivered. For accurate speed control it is advisable to use a separately excited motor i.e. Armature and Field Windings supplied through independent dc rectifiers AC SUPPLY If Ra Rf CONTROLLED DIODE RECTIFIER Va Vf RECTIFIER E ❑ The diode rectifier supplies constant field current maintaining a fixed value of flux, . ❑ The controlled rectifier (supplying the armature winding) provides a fully variable armature terminal voltage, Va. Ia If AC SUPPLY Ra Rf If Va Vf Ra Rf CONTROLLED Va Vf DIODE E Nf RECTIFIER RECTIFIER E armature field winding winding Va − I a R a Va = E + IaRa But, E = k..n Va = kn + IaRa n= kφ ❖ Ra is usually small so Va > IaRa. Thus with constant the speed n, is almost directly proportional to Va. ❖ Used for accurate speed control. (a) series-wound motor, (b) shunt-wound motor, (c) compound motor (d) separately excited motor, (e) torque–speed characteristics Control of brush-type dc Motor The speed of the dc motor depends on the current through the armature coil PWM: (a) principles of PWM circuit, (b) varying the armature voltage by chopping the d.c. voltage Control of brush-type dc Motor -Two direction control of DC motor -direction and speed control of dc motor (with additional logic circuit) (a) Basic transistor circuit, (b) H-circuit, (c) H-circuit with logic gates Control of brush-type dc Motor Speed control with feedback AC Motor (a) Single-phase induction motor, (b) three-phase induction motor, (c) three-phase synchronous motor Variable speed a.c. motor Servomotors Sometimes called control motors, are electric motors that are specially designed and built for use in feedback control system as output actuators. Ratings: fractional of watts to several 100 watts Higher speed response, smaller in diameter and longer in length Normally operate at low or zero speed Used in robots, radar, computers, tracking and guidance systems and in process control. Both DC and AC servomotors are used at present DC Servomotors AC Servomotors Most ac servomotors in control systems are of the two-phase squirrel cage IM Operating frequency normally 60 or 400Hz higher frequency is preferred in airborne system. A two-phase ac servomotor is shown below: Stepper Motor A stepper motor rotates by specıfıc number of degrees ın response to an ınput electrıcal pulse. Typical step size are 20, 2.50,50, 7.50, and 150 for each electical pulse It is electromechanical increamental actuator that can convert digital pulses into analog output shaft motion. In normal situation No position sensor or feedback is required to make the output response Typical applications: printers, tape and disk drives, machine tools, process control, X-Y recorders or motion, and robotics Stepper Motor Stepper motors have been built to follow signals as rapid as 1200 pulses per second with power ratings up to several horsepower Types of Stepper motor: 1- Variable reluctance: a- single stack b- multistack 2- Permanent magnet type Variable Reluctance Stepper Motor Single stack Stepper Motor A basic of 4 phase – 2 pole, single stack stepper motor is shown When the stator phases are excited with dc current in proper sequence, the resultant airgap field steps around and the rotor follows the axis of the airgap field by virtue of reluctance torque. This reluctance torque is generated because of the tendency of the ferromagnetic rotor to align itself along the direction of the resultant magnetic field Single stack Stepper Motor Figures show the mode of operation for 450 step in the clockwise direction. The windings are energized in the sequence A, A+B, B, B+C, and so forth The direction of rotation can be reversed by reversing the sequence of switching the windings, that is A, A+D, D, D+C, etc Single stack Stepper Motor In order to obtain smaller step sizes, multipole rotor construction is used. Figure shows four phase, six pole stepper motor When phase A is excited pole P1 is aligned with the axis of phase A, next phase A+B is excited, the resultant field axis moves in the clockwise direction by 450 and pole P2, nearest to this new resultant field axis is pulled to align with it. The motor therefore steps in the anticlockwise direction by 150. So, if the windings are energized in the sequence A, A+B, B, B+C, etc, the rotor rotates in step of 150 in anticlckwise direction. Setp size= 360 / (No. Of phases X no of rotoBroltotn,eMe echtah tro) nics PowerPoints, 4 th Edition, © Pearson Education Limited 2008 Multistack Stepper Motor They are widely used to give smaller step sizes The motor is divided along its axial length into magnetically isolated sections (“stacks”), and each of these sections can be excited by separate winding “phase” Three-phase arrangements are most common, but motors with up to seven stacks and phases are available. Longitudinal cross section of a three-stack variable reluctance stepper motor Multistack Stepper Motor The stator of each stack has a number of poles. Both stator and rotor has the same number of teeth. Therefore when a particular phase is excited, the position of the rotor relative to the stator in that stack is accurately 360 defined. Stepsiz = NRotorTeeth When stack A is energized, the rotor and stator teeth in stack A are aligned but those in stacks B and C are not Next if excitation is changed to B, the stator and rotor teeth in stack B are aligned, this is made possible by a rotor movement in the clockwise direction. Permanent Magnet Stepper Motor It has a stator construction similar to that of the single –stack variable reluctance type, but the rotor is made of permanent magnet material. Figure shows two pole permanent magnet stepper motor. The rotor poles align with two stator teeth (or poles) according to the winding excitation The current polarity is imoprtant in the pm stepper motor. PM two-phase stepper motor with 90°steps. (a), (b), (c) and (d) show the positions of the magnet rotor as the coils are energised in different directionBoslton, Mechatronics PowerPoints, 4 th Edition, © Pearson Education Limited 2008 Hybrid Stepper Motor Stepper motor control: Drive circuit When a two phase motor have 4 connecting wires for signals to generate the required signals, it termed bipolar motor Figure 9.26 (a) Bipolar motor, (b) H-circuit Switching sequence for full-stepping bipolar stepper Half-steps for bipolar stepper Stepper motor control: Drive circuit When a two phase motor have 6 connecting wires for signals to generate the required signals, it termed bipolar motor, they can be switched with just 4 transistor Figure 9.27 Unipolar motor Switching sequence for full-stepping unipolar stepper Switching sequence for Half-steps for unipolar stepper Bolton, Mechatronics PowerPoints, 4th Edition, © Pearson Education Limited 2008 Analysis of Stepper Motor Drives Advantages High power conversion efficiency. The widespread availability of power supply. No pollution of the working environment. The basic drive element in an electric motor is usually lighter than that for fluid power. Structural components can be lightweight. The drive system is well suited to electronic control. Disadvantages 1. A larger and heavier motor must be used which is costly. 2. Poor dynamics response. 3. Compliance and wear problems are causing inaccuracies. 4. Conventional gear-driven creates a backlash. 5. Electric motors are not intrinsically safe. They cannot, therefore, be used in for explosive atmospheres. Hydraulic Power System With a hydraulic system, pressurized oil (fluid) is provided by a pump driven by an electrical motor The pump pumps oil from a sump through a non return valve and an accumulator to the system, from which it return to the sump. The pressure relief valve is to release the pressure if it rises above a safe level Hydraulic Power System Hydraulic Power System Accumulator The accumulator is to smooth out any short term fluctuations in the output oil pressure The Accumulator Work Accumulator is a container in which the oil is held under pressure against an external force, which involves gas within bladder in the chamber containing the hydraulic fluid If the oil pressure rises then the bladder contracts increase the volume the oil can occupy and so reduces the pressure If the oil pressure falls the bladder expands to reduce the volume occupied by the oil and so increases its pressure. Pneumatic Power System Pneumatic Power System The air inlet to the compressor is likely to be filtered and via a silencer to reduce the noise level. In pneumatic power system an electric motor drives an air compressor. A pressure relief valve provides protection against the pressure in the system rising above a safe level. Pneumatic Power System Since the compressor increase the temperature of the air, there likely to be a cooling system and to remove contamination and water from the air, a filter with water trap is used. An air receiver increases the volume of air in the system and smoothes out any short-term pressure fluctuations. Directional Control Valves Pneumatic and hydraulic systems use directional control valves to direct the flow of fluid through a system; its ON/OFF devices either completely open or closed They might be activated to switch the fluid flow direction by means of mechanical, electrical or fluid pressure signal Common Types: 1-Spool valve: Move horizontally within the valve body to control flow 2-Rotary spool valve: have the same idea, when rotates opens and closes ports 3-Poppet valve: This valve is normally in closed condition. In this valve, balls, discs or cones are used in conjunction with valve seats to control the flow. When the pushbutton is depressed, the ball is pushed out of its seat and flow occurs as a result of port 1 being connected to port 2. When the button is released, the spring forces the ball back up against its seat and so closes off the flow. Directional valves Free flow can only occur in one direction through the valve, flow in the other direction is blocked by spring. Valve Symbols The valve symbol consists of square for each of its switching positions. As for the poppet valve there are two positions, one with the button not pressed and one with it pressed. Thus two positions valve will have two squares, a three positions valve have three squares Arrow headed lines are used to indicate the directions of flow in each position closed flow lines; blocked off Symbols of Valve actuation It indicates the various ways the valves can be actuated Ports are labelled 1 (or P) for pressure supply 3 (or T) for hydraulic return port 3 or 5 (or R or S) for pneumatic exhaust ports 2 or 5 (B or A) for output ports Thus, for Poppet Valve Solenoid operated spool valve The valve is actuated by a current passing through the solenoid and return to its original position by spring A simple example of an application of valves in a Pneumatic lift system Pilot-operated valves In pilot operated system one valve is used to control a second valve. The pilot valve is small size and can be operated manually or by a solenoid It is used to overcome when the force required to move the ball shuttle in a valve can often be too large for manual or solenoid operation Pressure control valve Three main types 1- Pressure limiting valve: used to limit the pressure in a circuit to below some value 2- Pressure regulation valve: used to control the Operating pressure in a circuit and maintain it at Constant value. 3-Pressure sequence valve: are used to sense the pressure of an external line and give a signal when it reaches some preset value. (a) Pressure sequence valve symbol, (b) a sequential system Hydraulic/Pneumatic linear actuators, Cylinders Both hydraulic and pneumatic actuators have the same principles, differences being in size The cylinder consists of a cylindrical tube along which a piston/ram can slide They are of two types: Single acting and double acting Cylinders: Single acting Single acting: The control pressure is applied to one side of the piston When a current passes through the solenoid, the valve switches position and pressure is applied to move the piston along the cylinder. When current ceases, the valve reverts to it is initial position and the air is vented from the cylinder. Control of a single-acting cylinder with (a) no current through solenoid, (b) a current through the solenoid Cylinders: Double acting Are used when control pressure are applied to both side of the piston. A difference in pressure between the two sides results in motion of the piston (No spring) Current through one solenoid causes the piston to move in one direction Control of a double-acting cylinder with solenoid, (a) not activated, b) activated Cylinder sequencing Many pneumatic or hydraulic control systems may require a sequence of extension and retraction of cylinders to occur Suppose we have two cylinders: A and B If the start button pressed; { piston A extends; If fully extended then piston B extends; If both A and B fully extended then Piston A retracts; If A is fully retracted have piston B retract} INTRODUCTION Pumps The function of a pump is to convert mechanical energy into hydraulicenergy. It is the heart of any hydraulic system because it generates the force necessary to move theload. Mechanical energy is delivered to the pump using a prime mover such as an electricmotor. Partial vacuum is created at the inlet due to the mechanical rotation of pump shaft. Vacuum permits atmospheric pressure to force the fluid through the inlet line and into thepump. The pump then pushes the fluid mechanically into thefluid poweractuateddevices suchasamotororacylinder. 11 HYDRAULIC PUMPS 11 Gear Pumps Gear pumps are less expensive but limited to pressures below 140 bar. It is noisy in operation than either vane or piston pumps. Gear pumps are invariably of fixed displacement type, which means that the amount of fluid displaced for each revolution of the drive shaft is theoreticallyconstant. 11 B-Vane pumps The operation of the vane pump is based on , the rotor which contain radial slots rotate by a shaft and rotate in cam ring (housing), each slot contain a vane design as to comes out from the slot as the rotorturns. During one half of the rotation the oil inters between the vane and the housing then this area starts to decrease in the second half which permit the pressure to be produced , then the oil comes out pressurizes to the outputport. Types of vane pump 1 Fixed Displacement vane pump 2 Variable Displacement vane pump 11 Unbalanced vane pump In this type of pump the eccentricity between pump cam-ring and rotor is fixed and pump discharge always remain same at a particular pressure. There are two types of fixed displacement VanePump: 1. Unbalanced Vane Pump 2. Balanced Vane Pump 11 Unbalanced vane pump A slotted rotor is eccentrically supported in a cycloidal cam. The rotor is located close to the wall of the cam so a crescent-shaped cavity is formed. The rotor is sealed into the cam by two side plates. Vanes or blades fit within the slots of the impeller. 11 11 11 2- Balanced vane pump: A balanced vane pump is one that has two intake and two outlet ports diametrically opposite eachother. Pressure ports are opposite each other and a complete hydraulic balance is achieved. One disadvantage of the balanced vane pump is that it can not be designed as a variable displacementunit. It have elliptical housing which formed two separate pumping chambers on opposite side of the rotor. This kind give higher operating pressure. 11 12 12 2-Variable Displacement Vane Pump In variable displacement the discharge of pump can be changed by varying the eccentricity between rotor and pump cam- ring. As eccentricity increases pump dischargeincreases. With decrease in eccentricity discharge decreases and oil flow completely stop when rotor becomes concentric to pump cam ring. 12 12 Compressors 12 Advantage of vanepump 1- low noise but higher than screwpump. 2- range of work from 500 – 1800r.p.m 3- semi continuous flow 4- pressure of work between 50 – 80bar 5-the vane motor must have spring backward to the vane to face the flow. 12 Piston pump Piston pump mainly divided into two main types, axial design which having pistons that are parallel to the axis of the cylinder block. Axial design have threekinds, 1 bent axis pump. 2 swash plate pump. The second type is the radial design, which has pistons arranged radially in a cylinderblock. 12 12 Bent Axis Design Swashplate Principle Radial Piston Pump Screw pumps