PDF 4th Class Edition 3 Part A Introduction to Process Measurement
Document Details
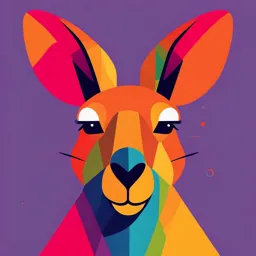
Uploaded by Reusl
Tags
Summary
This document is an introduction to process measurement, focusing on flow measurement techniques and devices, such as nutating disk meters and orifice plates. It provides descriptions and diagrams to clarify the concepts.
Full Transcript
Introduction to Process Measurement • Chapter 2 Objective 3 Describe the types of flow sensing and measuring devices. Flow Measurement Flow is one of the most widely measured process variables. In power plants, feedwater, makeup water, steam and fuel flows are essential for determining and optimiz...
Introduction to Process Measurement • Chapter 2 Objective 3 Describe the types of flow sensing and measuring devices. Flow Measurement Flow is one of the most widely measured process variables. In power plants, feedwater, makeup water, steam and fuel flows are essential for determining and optimizing plant operating conditions. Many methods are used to measure flow. The instruments used must be suited to the temperature, pressure, density, and cleanliness of the fluid being measured. Here, the most common flow instruments are discussed. Nutating Disk Meter A nutating disc meter, as shown in Figure 28, has a flat circular disc with a ball-like structure at the center. The bottom part of the ball rests in a socket, while the top center of the ball has a small shaft that turns a gear. The gear drives small dials that rotate to indicate flow (in, say litres/minute), and a counter (or integrator) that totalizes the actual flow (in say m3). The flat disc has a slot with a fixed vertical partition. This division plate directs the fluid flow through the meter from the inlet to the outlet. When fluid flows through the meter, the disc wobbles on its ball and socket. This causes the small shaft to nutate; it follows a circular path around the centre of the plate. Each nutation of the wobble disc permits a discrete volume of fluid to pass through. The rate at which the plate wobbles is proportional to the volumetric flow rate of the fluid. These meters are commonly used to measure the flow of clean water. Typical power plant applications include measuring: • Building potable water flow • Cooling tower makeup water flow • Boiler water makeup flow Nutating discs are not suitable for high pressure or high temperature service. Typically, they are limited to 1035 kPa and 120°C. As well, they are not suitable for slurries, dirty fluids, or viscous fluids. Many nutating disc meters are equipped with digital operating heads and transmitters to provide consumption information to central control stations. Figure 28 – Nutating Disc Flow Meter Operation Shaft Gear Disc Ball 4th Class Edition 3 • Part A 2-25 Unit A-9 • Energy Plant Instrumentation and Controls Figure 29 shows two disassembled nutating disc meters. On the left-hand image, the operating head has been removed. The nutating disc, ball, and shaft can be clearly seen. In the right-hand image, the head is in place, and the gear drive for the integrator mechanism is visible. Figure 29 – Nutating Disc Flow Meter Integrator Shaft Disc Inlet Ball Division Plate Outlet Head meters Head type or differential pressure flow meters include a number of sensing devices for fluid flow measurement, such as orifice plates, venturi tubes, flow nozzles, and pitot tubes. Orifice plates, venturi tubes, and flow nozzles cause restriction in flow, which causes pressure to decrease across the restriction. The pressure difference across the restriction is related to the fluid velocity and the flow rate. If the net cross-sectional area of the fluid stream is reduced, the velocity of flow increases. This results in an increase in kinetic energy. Since energy cannot be created or destroyed, the increase in kinetic energy comes as a result of a decrease in potential energy (pressure) downstream of the restriction. Orifice Plates An orifice plate (Figure 30) is the most common form of head meter that is used in flow measurement. It consists of a flat metal plate with an opening of a fixed area. The concentric type, shown in Figure 30(a), is the most common. The eccentric and segmental in Figures 30(b) and 30(c) are used in special applications. The outside of the plate is designed to fit inside the bolt circle on standard flanges. Figure 30 – Orifice Plates Concentric Orifice (a) 2-26 Eccentric Orifice (b) 4th Class Edition 3 • Part A Segmental Orifice (c) Introduction to Process Measurement • Chapter 2 Figure 31 illustrates the pressure drop (the differential pressure) across an orifice plate. Note that the flow pattern decreases in cross-section, downstream of the plate. The point of maximum fluid velocity and greatest static pressure drop occurs at the narrowest point of flow, which is called the vena contracta. Downstream of the vena contracta, some of the pressure recovers to its previous value. However, turbulence and friction create a significant permanent pressure loss. Figure 31 – Pressure Drop Across an Orifice Plate Orifice Plate Static Pressure Vena Contracta The pressure differential across an orifice is measured using two pressure connections, one upstream of the plate, and another downstream. Figures 32(a) and 32(b) show two types of connections. In Figure 32(a), the pressure connections (called taps) are located directly on the flanges, while in Figure 32(b) the taps are located on the pipe at a specific distance from the orifice plate. Figure 32 – Flange and Pipe Taps 2½ times the Diameter 9.5mm (3/8”) Extra Heavy Coupling Weld Weld 12.7mm (½”) Hole Remove Burrs Flow Flange Taps (a) 8 times the Diameter Pipe Taps (b) 4th Class Edition 3 • Part A 2-27 Unit A-9 • Energy Plant Instrumentation and Controls Figure 33 – Pipe Flange Figure 33 shows a pipe flange with an installed orifice plate and flange taps. Orifice plates are easy to install and replace. They are inexpensive and available in different sizes to suit the required flow range. However, orifice plates are the least accurate flow-sensing element, and create the greatest permanent pressure loss of all the flow-sensing elements. Venturi Tube The venturi tube is a carefully designed length of pipe, designed to be installed between pipe flanges (Figure 34). Its cross-sectional area converges to a minimum, called the throat, and then diverges to the original pipe size. High- and low-pressure connections are installed at specific locations as indicated. The venturi tube produces the least permanent pressure loss than both an orifice plate and the flow nozzle (90% or more of the upstream pressure is recovered downstream). On the other hand, it has the disadvantages of higher cost and bulkiness. Venturi tubes are frequently used to measure large flows of water. Figure 34 – Venturi Tube High Pressure Low Pressure Throat Section Main Section 2-28 4th Class Edition 3 • Part A Introduction to Process Measurement • Chapter 2 Flow Nozzle The flow nozzle (Figure 35) is like a venturi tube without the diverging section. Its pressure recovery is better than that of the orifice plate, but not as good as the venturi tube. Flow nozzles are mainly used to measure high velocity flows. The high-pressure connection is located one internal pipe diameter before the inlet face of the nozzle. The low-pressure tap is usually one-half the pipe diameter downstream. Figure 35 – Flow Nozzle D D d Pitot Tube The principle of operation of the pitot tube is shown in Figure 36. The tube on the left measures the static pressure inside the pipe. The tube on the right measures both the static pressure and the pressure due to the fluid velocity. The difference between these two measurements is used to calculate the volumetric fluid flow within the pipe. Figure 36 – Pitot Tube Measurement Principle P(total) P(static) P(dynamic) = P(total) P(static) 4th Class Edition 3 • Part A 2-29 Unit A-9 • Energy Plant Instrumentation and Controls Variable Area Meter The variable area meter (or rotameter) consists of a clear tapered tube marked with a scale. A float inside the tube moves upwards due to the flow of the fluid. As the flow increases, the float moves up the tube until the differential pressure across the float reaches zero. Figure 37(a) shows a rotameter used to measure and control the flow of air or some other gas. The gas flow can be adjusted by using the flow control knob at the bottom of the tapered tube. An increase in the flow moves the ball float upwards in the tube. The flow rate (in SCFH or standard cubic feet per hour) is read from the scale at the middle of the ball float. Figure 37(b) is a rotameter used to measure the flow of a liquid. The float moves upward in the tapered tube when flow increases. In this type of meter, the top of the float indicates the flow. The measurement units are shown in GPM (gallons per minute) or LPM (litres per minute). Figure 37 – Rotameters Tapered Glass Tube Measurement Unit Scale Float Flow Control (a) (b) Weir Weirs (Figure 38) are structures used to measure liquid flow. The weir itself is a partial obstruction, often made of metal plate, and placed across an open channel. The weir has a carefully shaped opening or notch that allows liquid to flow through. A float, bubbler, or ultrasonic sensor is placed upstream of the weir to detect the height of water above the base of the weir notch. The weir causes the height (or head) of the upstream liquid to increase. The flow rate through a weir depends on the head upstream of the weir. 2-30 4th Class Edition 3 • Part A Introduction to Process Measurement • Chapter 2 Figure 38 – Weir Flow Measurement Principle Float Fluid Height Above Notch Weir Figure 38 shows a weir with a trapezoidal notch, and a float-type level indicator to measure the height of the upstream liquid. Weirs can be used to measure the flow of water, wastewater, and sewage. They are not well suited for viscous fluids or slurries. The base of the weir notch is called the crest. A broad-crested weir has a long crest that runs across the entire flow channel, just below the surface of the water. These weirs are used to measure flows of dam spillways or rivers, and are generally constructed of concrete. Ultrasonic Ultrasonic flow meters use ultrasonic transducers to transmit and measure ultrasound passing through a fluid stream. There are two types of ultrasonic flow meters commonly in use: 1. Transit-time flowmeter 2. Doppler flowmeter The first type compares the transit time of the sound wave travelling with the liquid flow and the transit time of the sound wave travelling against the flow. The flow measurement is calculated based on the difference between the two transit times. Figure 39 shows an example of a transittime flowmeter. The Doppler flowmeter measures the shift in frequency from a sound wave travelling with the flow to a sound wave travelling against the flow (Doppler shift). This method is less accurate than the transit-time measuring system. The ultrasonic flow meter is accurate and has no moving parts. Ultrasonic transducers may be permanently installed in the pipe, or temporarily attached to the outside of a pipe. If attached to the outside of a pipe, the ultrasonic transducers do not contact the process fluid, which can be a distinct advantage. These meters are well suited for measuring the flow of gases, clear liquids, and liquids containing sound reflecting particles. 4th Class Edition 3 • Part A 2-31 Unit A-9 • Energy Plant Instrumentation and Controls Figure 39 – Ultrasonic Transit-time Flow Meter Pipe Ultrasonic Signal 1 Transducer 2 Flow Process Fluid Flow Transducer 1 Ultrasonic Signal 2 Mass Flow Meters Most flow meters measure the volumetric flow rate. To find the mass flow rate, the volumetric flow rate must be multiplied by the fluid density. This method is fine in many situations. However, if the density of the fluid varies due to changes in temperature, pressure, or composition, this method is very inaccurate. Mass flow meters compensate for density changes by measuring the mass flowing past a specific point over a unit of time (e.g. kilograms per second). Changes in the density of the fluid will not result in meter inaccuracy. Figure 40 shows a mass flow meter called a Coriolis meter. It has two parallel tubes through which fluid flows. The tubes have a vibration induced into them by an electromagnetic device. The vibration causes the tubes to vibrate toward and away from each other, like a tuning fork. When there is no flow, the distance between the tubes at the inlet will be the same as the distance between the tubes at the outlet. When there is flow through the tubes, the frequency of vibration shifts, changing the distance between the tubes at the inlet and the outlet. The greater the mass passing through the tubes, the greater the difference in the distance between the tubes. This distance between the tubes is measured near the inlet and near the outlet and the difference is used to determine the mass flow rate. Figure 41 shows a compact Coriolis meter installed in a pipe and with an attached flow indicator transmitter. 2-32 4th Class Edition 3 • Part A Introduction to Process Measurement • Chapter 2 Figure 40 – Coriolis Flow Meter Principle Induced Vibration h1 h2 Induced Vibration a) No flow - symmetrical vibrations (h 1 = h2) Induced Vibration h2 h1 Induced Vibration b) Full flow - asymmetrical vibrations (h 1 > h2) Figure 41 – Coriolis-Type Flow Indicator Transmitter Flow Indicator Transmitter Coriolis Meter Coriolis meters are used successfully in the petro-chemical, food and beverage, pulp and paper and pharmaceutical industries. In part, this is because the Coriolis meter can accurately measure viscous fluids and slurries, as well as low viscosity and clean fluids. 4th Class Edition 3 • Part A 2-33