Instrumental Analysis Lecture 4 PDF
Document Details
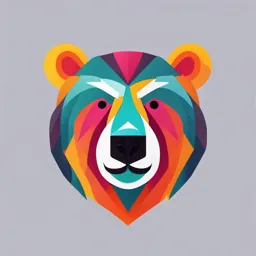
Uploaded by SparklingLitotes
Pharmacy
2024
Dr. Anwar Salem
Tags
Related
Summary
This lecture provides an overview of instrumental analysis, focusing specifically on atomic spectroscopy techniques such as atomic absorption spectroscopy (AAS), atomic emission spectroscopy (AES), and atomic fluorescence spectroscopy (AFS). It covers fundamental principles, instrumentation, and sample preparation methods crucial for analytical chemistry applications.
Full Transcript
Instrumental analysIs lecture 4 MARCH 7, 2024 2ND YEAR PHARMACY Dr. Anwar Salem Atomic Spectrometry Spectroscopy is the study of interactions between matter and different forms of electromagnetic radiation; when practiced to quantitative analysis, the term spectrome...
Instrumental analysIs lecture 4 MARCH 7, 2024 2ND YEAR PHARMACY Dr. Anwar Salem Atomic Spectrometry Spectroscopy is the study of interactions between matter and different forms of electromagnetic radiation; when practiced to quantitative analysis, the term spectrometry is used. Atomic spectroscopy includes the techniques of atomic absorption spectroscopy (AAS), atomic emission spectroscopy (AES), atomic fluorescence spectroscopy (AFS), X-ray fluorescence (XRF), and inorganic mass spectroscopy (MS). AAS, AES, and AFS exploit interactions between UV-visible light and the valence electrons of free gaseous atoms. Atomic spectroscopy: general principles Every element has a characteristic atomic structure, with a small, positively charged nucleus surrounded by a sufficient number of electrons necessary to maintain neutrality. Electrons settle into orbitals within an atom and one of the electrons can also jump from one energy level to the higher level by acquiring the necessitated energy (Figure). This energy is provided by colliding with other atoms, such as heating-AES, photons derived from light-AAS and AFS, or high- energy electrons-XRF. Possible transitions happen, when the required energy reaches to the difference between two energy states (ΔE). A neutral atom may exist at a low energy shell or ground state (E0), or at any of a group of excited states depending on how many electrons have been jumped to higher energy levels (E′’) although it is normal to think for the first transition. Each element has a unique energy level and the ΔEs associated with transitions between those levels. Figure. Energy level diagrams to show transitions associated with (a) AAS, (b) AES, and (c) AFS. The vertical arrows indicate absorption or emission of light. When light of a specific wavelength enters an analytical system, outer shell electrons of the corresponding atoms will be excited as energy is absorbed. As a result, the amount of light transmitted from the system to detector will be reduced, this is understood as AAS (Figure a). Under appropriate circumstances, outer shell electrons of vaporized atoms may be excited by heating. As these electrons return to the more stable ground state, energy is lost. As Figure b shows, some of this energy is emitted as light, which can be measured with a detector, this is AES. Some of the radiant energy absorbed by ground state atoms can be emitted as light as the atom returns to the ground state i.e. AFS (Figure c). Atomic spectroscopy: instrumentation Formation of the atomic vapor i.e. atomization is the major principle of emission, absorption, and fluorescence techniques. The most critical component of instruments used in atomic spectroscopy is the atomization sources and sample introduction devices with an associated spectrometer for wavelength selection and detection of light. Atomization involves the several key (the basic) steps: solvent removal, separation from anion and other elements of the matrix, and reduction of ions to the ground state atom. The design of an AFS instrument is similar to those for AAS and AES except that the light source and the detector are located at a right angle Figure. Schematic diagram of an AAS, AES, and AFS instrument. A light source which emits the sharp atomic lines of the element to be determined is selected. There are two types of light sources used in these instruments: continuous sources and line sources. A continuous source, also called to as a broad- band source, emits radiation over a broad range of wavelengths. A line source, on the other hand, emits radiation at specific wavelengths, but this source of radiation is not as pure as radiation from a laser. Table 1 provides a list of most common kinds of lamps considered to be light sources. The atomizer is any device which will produce ground state atoms as a vapor into the light path. Many atomizers utilized for AFS are similar to those used for AAS and AES. The atomizers most commonly used in these techniques are flames and electrothermal atomizers The flame provides for easy and fast measurements with few interferences and is preferred at any appropriate concentration for the analyte. Flame atomizers contain a pneumatic nebulizer, an expansion chamber, and an air-acetylene laminar flame with a 10 cm path length. The typical pneumatic nebulizer for sample introduction is insufficient, and although elements such as Na and K can be determined in biological samples by flame AES, flame atomization is more suitable for AAS and AFS. AAS measurements can detect concentrations at approximately 1 μg/ml (ppm) or more. Devices are being developed to overcome these limitations of the typical nebulizer. Atomization can be reached to 100% and the devices can also generate the sample as a pulse flow rather than the continuous flow. Most systems use a graphite tube which is heated electrical energy, a technique called graphite furnace atomization, although other materials are sometimes employed. A programmed sequence of the furnace temperature is used in electrically heated graphite tube. With this atomizer, 10–50 μl of test solution is dried, organic material is destroyed, and the analyte ions dissociated from anions for reduction to ground state atoms. This atomizer also produces temperatures up to 3000 K which allows to form an atomic vapor of refractory elements such as aluminum and chromium. Since the analyte is atomized and retained within a small volume furnace, this procures a dense atom population. The technique is extremely sensitive as it allows one to detect a few μg/ml concentrations of the analyte. Although the technique is widely used for AAS, electrothermal atomization will provide a better performance for both AES and sample introduction into an inductively coupled plasma. Traditional sources usually include arcs and sparks but modern instruments use argon or some other inert gas to create plasma. An ideal wavelength selector has a high throughput of radiation and a narrow effective bandwidth. There are two major types of wavelength selectors —filters and monochromators. A simple example of an absorption filter is a piece of colored glass. Absorption filters provide effective bandwidths of 30–250 nm, although the throughput can be only 10% of the source’s emission intensity at the low end of this range. Interference filters constructed of a several optical layers deposited on a glass or transparent material. Typically, effective bandwidth is 10–20 nm, with maximum throughputs of at least 40%. A monochromator is used to convert a polychromatic source of radiation at the entrance slit to a monochromatic source of restricted effective bandwidth at the exit slit. These devices are classified as either fixed-wavelength or scanning. The wavelength selects by manually rotating the grating in a fixed-wavelength monochromator. A scanning monochromator includes a drive mechanism that continuously rotates the grating, allowing sequential wavelengths to exit from the monochromator (Figure) Figure. Schematic diagram of wavelength selectors: (a) filters and (b) a diffraction grating monochromator. Detectors use a sensitive transducer that converts a signal comes from light energy into electrons. An ideal detector produces signal, S, is a linear function of the electromagnetic radiation’s power, P, S=kP+D where k is the detector’s sensitivity and D is the detector’s dark current, or the background current when no radiation of source reached to the detector. Atomic spectroscopy: sample preparation An ideal sample preparation should remove interfering components from the matrix and to adjust of analyte to facilitate the actual measurement. Methods for destruction of the organic matrix by simple heating or by acid digestion have been developed and are thoroughly approved. Microwave heating is used for this purpose, with the specifically designed a compatible equipment to avoid dangerous of excessive pressure within reaction flask. Although the number of samples that can be processed is not large, microwave heating affords rapid digestion and low reagent blanks. More recent developments include continuous flow systems for automated digestion which has a direct link with the instrument. Liquid-liquid portioning has been widely applied for preconcentration procedure. Analyte atoms in a large volume of aqueous solution are complexed with a suitable agent and collected into a small volume of solvent. Vapor generation procedures permit the rapid introduction of 100% of the sample into the atomizer and are used for AAS, AES, AFS, and ICP-MS. Certain elements such as arsenic, selenium, and bismuth readily evolve gaseous hydrides and transferred by a flow of inert gas to an AES, and ICP-MS or to a heated silica tube positioned in the light path for AAS, AFS. The tube can be heated using the air-acetylene flame or an electric current. The obtained heat is enough to cause decomposition of the hydride and atomization of the analyte. Thus, there is no loss off analyte, which in all the atoms flow the light path with in few seconds and they are trapped within the silica tube that was retarded their dispersion. Any sample volume added to the reaction container, hydride generation AAS has detection limits a few nanograms of analyte. Mercury can quickly form a vapor in the ambient temperature, and this property is the basis for cold vapor generation. When a reducing agent is added to sample solution, Hg2+ converts to the elemental mercury. Agitation or bubbling of gas through the solution is used to enhance rapid vaporization of the atomic mercury and to improve the transfer of mercury to a flow through cell located in the light path. As with hydride generation, the detection limit is a few nanogram and some manufacturers have been developed common instrumentation to accomplish both procedures. Chromatographic or electrophoretic techniques have been also developed that are coupled directly to the atomic spectroscopic instrument to develop integrated analytical arrangements Atomic spectroscopy: detection limits The detection limits are important parameters of analytical techniques. AAS detection limits are generally better in all cases where the element can be atomized. Detection limits for refractory elements such as born, titanium, and vanadium are better by ICP than by AAS. Nonmetals and the halogens can only be determined by ICP. Optimum detection of nonmetals such as sulfur, nitrogen, and halogens by ICP-ES can only be achieved when a vacuum monochromator is used. For mercury and those elements that form hydrides, the cold vapor mercury or hydride generation techniques offer exceptional detection limits Atomic absorption spectroscopy? Atomic absorption spectroscopy (AAS or AA spectroscopy) is one of the earliest elemental analysis techniques to be commercially developed. So, what is atomic absorption spectroscopy? How does atomic absorption spectroscopy work? Flame atomic absorption spectroscopy (Flame AAS or FAAS) was developed in 1952 and first commercially released as an analytical technique in the 1960s. Since then, the technique has remained popular for its reliability and simplicity. AAS is an analytical technique used to determine how much of certain elements are in a sample. It uses the principle that atoms (and ions) can absorb light at a specific, unique wavelength. When this specific wavelength of light is provided, the energy (light) is absorbed by the atom. Electrons in the atom move from the ground state to an excited state. The amount of light absorbed is measured and the concentration of the element in the sample can be calculated. An electron is excited from the ground state to higher energy level by absorbing energy (light) at a specific wavelength. In atomic absorption spectroscopy, the wavelength of absorbed light is determined by the type of atom (which element it is) and the energy levels the electrons are moving to. How much light is absorbed is determined by the concentration of the element in the sample. AA Spectroscopy, an example In AAS, a solution containing the analyte is introduced into a flame. The flame converts samples into free ground state atoms that can be excited. A lamp emitting light at a wavelength specific to the atoms is passed through the flame, and as the light energy is absorbed, the electrons in the atoms are elevated to an excited state. The Beer-Lambert Law describes the relationship between light absorption and concentration of the element. According to the law, the amount of light absorbed is proportional to the number of atoms excited from the ground state in the flame. The following diagram shows the energy levels in a lead (Pb) atom. Energy from the heat of the flame causes atoms to freely dissociate. The amount of energy required for the electrons to move between energy levels corresponds to specific wavelengths of light. As the diagram shows, moving an electron from the ground state of a Pb atom to the first energy level (E1) requires energy equivalent to light at 283.3 nm. It requires more energy to move an electron from the ground state to the second energy level (which is further away from the nucleus). For AAS analysis, the wavelength of the ground state to the E1 level is frequently of most interest, as it is the most intense. A strong absorbance band gives the best (lowest) detection limits. In samples where the concentration of the element is higher, an alternate wavelength can be used. Energy level diagram for lead (Pb). E0 to E1 is typically the transition of most interest for AAS analysis. Alternate wavelengths can be used depending on the sensitivity of the measurement required. How does an atomic absorption spectrometer work? Atomic absorption spectroscopy is studied using an atomic absorption spectrometer (AAS or AA spectrometer). The AA spectrometer works by: 1. Creating a steady state of freely dissociated ground state atoms using a heat source (flame) 2. Passing light of a specific wavelength through the flame. The wavelength corresponds to the amount of energy required to excite an electron from (typically) the ground to first excited state for a specific element. 3. Measuring the amount of the light absorbed by the atoms as they move to the excited state (the atomic absorption). 4. Using the measured absorbance to calculate the concentration of the element in a solution, based on a calibration graph. Let’s look at the components inside the AA spectrometer. A simple flame atomic absorption spectrometer includes: 1. A sample introduction system 2. The burner (flame) and its associated gas supplies: air-acetylene or nitrous oxide-acetylene 3. A light source, the hollow cathode lamp (HCL) 4. A monochromator (the optical components inside the box in the diagram) 5. An optical detector (photomultiplier tube or PMT) 6. Computerized instrument control, data collection, and analysis. AA spectrometer sample introduction system The liquid sample is transported via capillary tubing into the nebulizer. The pneumatic nebulizer makes use of the Venturi effect, the principle that fluid flows at a higher velocity through a narrower tube, to accelerate the solution stream. The fluid then impacts a glass bead to create a fine spray of droplets, known as an aerosol. Larger droplets drain to waste, while the fine aerosol is passed up into the spray chamber. The mixing paddles assist to further remove large droplets to maintain a homogenous flow of fine droplets into the spray chamber and burner. Mixing paddles also assist in minimizing burner blockage and ensuring thorough mixing of the oxidant/acetylene gases with the sample droplets. As the design requires the drain trap to be partially filled with liquid, a float is included to ensure that the liquid level is always maintained. The spray chamber bung is a safety device. It will safely release upon any abnormal build-up of gases in the sample introduction system. The solution passes through a nebulizer onto the glass bead. Large droplets are removed to the drain. The flame AA spectrometer burner The atomic absorption spectrometer (AAS) burner provides a steady state of ground state atoms. In flame AAS, the burner converts the aerosol/gas mixture created by the spray chamber and nebulizer, into free, ground state atoms. There are two common gas mixtures that are burnt to fuel the flame. They are air- acetylene and nitrous oxide-acetylene. Producing a flame around 2300 °C, air- acetylene is suitable for most elements. At around 2700 °C, the nitrous oxide- acetylene flame creates a more reducing environment, suitable for elements that are prone to form oxides. There are four main stages the aerosolized analyte solution goes through in a flame AAS burner. They are: 1. Desolvation, or drying. The solvent is evaporated, resulting in dry nanoparticles of the sample remaining. 2. Vaporization. The particles are converted to the gaseous phase 3. Atomization. The key stage at which the population of ground state, freely dissociated atoms is created. Ground state atoms are the target for AAS analysis. 4. Ionization. Some, but not all, free atoms will be converted to ions. This will depend on the flame conditions (gas mix) and the ionization potential of the analytes on solution. What are the benefits of Atomic Absorption Spectroscopy? Despite being one of the first elemental analytical techniques on the market, Atomic Absorption Spectroscopy (AAS) is still in widespread use across many industries. This is largely because the benefits of AAS are simplicity, reliability, and low cost while still delivering precise, accurate results. Flame atomic absorption spectrometry (FAAS) is an entry-level spectroscopy technique that is ideally suited to labs in which a small number of elements are routinely measured. Compared with other atomic spectroscopic techniques, flame AAS: 1. Has the lowest capital cost, making it a more affordable option 2. Is a good technique for those new to spectroscopic analysis, due to the simplicity of operation 3. Can deliver excellent sample throughput for a single element analysis 4. Can measure samples over a wide concentration range, from low ppm to percent levels, without the need to dilute the sample 5. Delivers accurate results 6. Can easily be operated manually or can be automated with an additional autosampler. This makes flame atomic absorption a very useful technique for most laboratories that are preparing their labs for elemental analysis, as well as established laboratories that have a particular need for a low-cost technique for the analysis of a handful of elements. However, there are some limitations of flame AAS that mean alternative techniques can be more favorable. Conventional FAAS systems can be slow, requiring the measurement of the same sample multiple times, once for each element. This can be time-consuming and is less than ideal when only a small amount of sample is available. Summary: In this lecture we learnt about: