Final Instrumental Analysis (1) PDF
Document Details
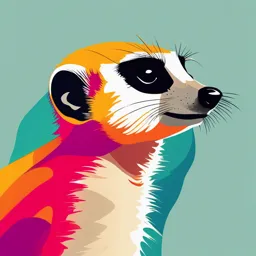
Uploaded by DistinctiveSpinel
Tags
Summary
This document appears to be lecture notes or study material on instrumental analysis, covering topics like polarimetry, atomic spectroscopy, and chromatography. It includes various techniques and their associated concepts.
Full Transcript
Table of Contents Table of Contents To Do Key All Instrumentation Diagrams Polarimetry Polarized Light Chirality Optical Rotation Instrument Atomic Spectroscopy Types of Atomic Spectroscopy Emission Absorption Fluorescents Difference...
Table of Contents Table of Contents To Do Key All Instrumentation Diagrams Polarimetry Polarized Light Chirality Optical Rotation Instrument Atomic Spectroscopy Types of Atomic Spectroscopy Emission Absorption Fluorescents Difference b/t atomic & molecular spectroscopy Flames, Furnaces, & Plasmas Flames Graphite Furnaces Comparison Flames vs Furance Plasmas Just incase what I have doesn’t make sense: Chat ICP Process: Temperature Affects Atomic Spectroscopy Boltzmann Distribution Effects on Temperature for Absorption vs Emission Instrumentation Principal Differences b/t Atomic & Ordinary Molecular Spectroscopy Atomic Linewidths Hollow Cathode Lamp Multi-Element Detection: Atomic Emission Background Correction Detection Limits Interference Spectral Interference Physical Interference Chemical Interference Ionization Interference ICP-MS Disadvantages: Collision and Dynamic Reaction Cells X-Ray Spectroscopy Instrumentation Sources Detectors Scanning Electron Microscopy Energy Dispersive X-Ray Spectrometer Wavelength Dispersive X-ray Fluorescence Data Interpretation X-Ray Diffraction Bragg’s Law Instrumentation Sample Preparation Guide to Atomic Spectrometry Technique Selection Analytical Separations Solvent Extraction pH Effects Metal Chelators Chromatography Adsorption Chromatography Partition Chromatography Ion exchange chromatography Size Exclusion Chromatography Affinity chromatography Plumber’s View Efficiency of Separation Resolution Diffusion Column Efficiency: Plate Height Why Bands Spread Plate Height Equation Longitudinal Diffusion (B/ 𝜇𝑥) Mass Transfer (C𝜇𝑥) Multiple Flow Paths (A) Column Type Asymmetrical Peaks Thin Layer Chromatography Plates Sample Application Plate Development Processing Plates Applications Solvent Systems Gas Chromatography Separation Process in Gas Chromatography Retention Instrumentation Column Temperature Programing Pressure Programing Carrier Gas Guard Columns & Retention Gaps Sample Injection Injection Port Detectors Qualitative Analysis Quantitative Analysis Detector Classifications Thermal Conductivity Detector Flame Ionization Detector Electron Capture Detector Mass Spectrometry Other Detectors Sample Preparation Solid Phase Microextraction (SPME) Stir-bar Sorptive Extraction Purge & Trap Pyrolysis Carbon Strips GC Quantitation Peak Height Peak Area Calibration Standards & Curve Internal Standard Method High-Performance Liquid Chromatography The Chromatographic Process Columns Stationary Phase The Elution Process: NP vs RP Mobile Phase Composition Solvents Chromatogram Peak Shape Instrumentation Pumps & Injection Valves Detectors Liquid chromatography Quantitation To Do Polarimetry Atomic Spectroscopy Atomic Absorption & Emission ICP MS X-Ray Spectroscopy X-ray diffraction Analytical Separation TLC GC GC Quantitation HPLC LC Quantitation Key Pink Highlight= Equation Blue Highlight= Definition Purple highlight= Important Green Light= Components All Instrumentation Diagrams Figure 1. Atomic Absorption Figure 2. ICP-MS Figure 3. Gas Chromatograph Figure 4. Polarimeter Polarimetry Certain molecules can rotate polarized light ○ This is due to the structure of the molecule, specifically carbon’s tetrahedral structure The change of the direction of vibration of polarized light when it interacts with an optically active material (chiral compound) is measured Polarized Light White light is unpolarized light ○ It vibrates randomly in all directions Plane polarized light ○ Composed of waves that vibrate in only one plane Unpolarized light passes through a polarizing filter, to get the light passing though is vibrating in only one direction Chirality A compound is optically active (chiral) if its molecules are not superimposable on their mirror images ○ Chiral compounds rotate the plane of polarized light ○ When polarized light is passed through an optically active substance, it emerges vibrating in a different plane A compound is optically inactive (achiral) if its molecules are superimposable on their mirror images Non-superimposable, mirror-image molecules are known as enantiomers ○ When enantiomers are mixed in equal ratios, the rotation caused by one enantiomer is cancelled by the other enantiomer. ○ This mixture is called a racemate and is optically inactive ○ The symbols (±) and (d/l) are used to describe racemic mixtures ○ Enantiomers possess identical physical properties Melting points, boiling points, and densities To correctly describe the three-dimensional arrangements of atoms around a chiral center, we use d and l nomenclature ○ d- and l- compounds rotate the plane of polarized light equally, but in opposite directions ○ Light rotated to the right (clockwise) is given the symbol (+ or d) – dextrorotatory ○ Light rotated to the left (counterclockwise) is given the symbol (- or l), levorotatory Optical Rotation The amount of rotation depends on how many chiral molecules the light encounters ○ The light will encounter more molecules as cell length and concentration increase ○ Directly proportional to optical rotation The amount of rotation also depends on the temperature and wavelength ○ The wavelength typically used in 589nm, but any can be used The amount of rotation is called optical rotation: Specific rotation is a physical property like melting point, boiling point, density or refractive index Instrument The instrument used to determine the angle through which the plane of polarization is rotated upon passing through an optically active substance. Consists of a light source, two identical polarizers, a sample tube between the two polarizers, and a detector ○ Source: tungsten filament ○ Polarizing filter: creates plane-polarized light ○ Sample cell: specific path length to hold sample ○ Analyzer: second polarizing filter that is rotated ○ Detector: photomultiplier tube The second polarizer is called the analyzer, but is optically identical to the first polarizer Process: ○ The instrument rotates the analyzer until the detector sees the minimum amount of light when the sample tube is filled with solvent only ○ When a chiral sample, dissolved in the same solvent used for the blank is placed in the tube, the instrument rotates the analyzer until the detector again sees a minimum amount of light The degrees of rotation of the analyzer are equal to the degrees of rotation of the plane of polarization of light from the chiral sample, giving rise to polarimetric measurements These measurements are given as either positive (+) or negative (-) values, depending upon the direction of rotation Atomic Spectroscopy Chapter 21 Types of Atomic Spectroscopy Emission In atomic emission, an excited state atom in the flame emits light (the atomic emission signal), which passes through a monochromator to a detector. Atomic emission transitions include emission from various excited states to the ground state. Collision in flame or plasma promote some atoms to excited electronic states from which they can emit photons to return to lower energy states No lamp is needed Emission intensity is proportional to concentration of element in sample Emission from atoms in plasma is dominant form of atomic spectroscopy Absorption In atomic absorption, light from a hollow cathode lamp passes through a flame. Atoms in the flame absorb some of the light, and the remainder (the atomic absorption signal) passes through a monochromator to a detector. Atomic absorption transitions include absorption from the ground state to various excited states. Figure 1. Atomic Absorption A liquid sample is aspirated through a plastic tube into the flame (2000-3000K) ○ Liquid evaporates and the remaining solid is atomized in the flame A hollow-cathode lamp contains an iron cathode: Cathode is made of same element whose emission is desired ○ When bombarded with energetic Ne+ or Ar+, excited Fe vaporize and emit light with the same frequencies absorbed by the analyte Fe in the flame Detector measures the amount of light that passes through the flame Fluorescents In atomic fluorescence, light from a line source is sent through a flame. The light excites the atoms in the flame, which then emit light as they relax to the ground state (the atomic fluorescence signal), which passes through a monochromator to a detector. ○ Atomic fluorescence transitions include absorption from the ground state to an excited state, nonradiative transition from a higher excited state to a lower excited state, and emission from various excited states to the ground state. Difference b/t atomic & molecular spectroscopy Width of absorption or emission bands ○ Spectra of liquids & solid molecules: bandwidths ~10-100 nm ○ Spectra of atoms of gaseous state: sharp lines, widths of ~0.001 nm Lines are so sharp that there is little overlap between spectra of different elements in the same sample Some instruments can measure more than 70 elements simultaneously What is the significance of a fuel and oxidant Flames, Furnaces, & Plasmas In atomic spectroscopy, analyte is atomized in a flame, an electrically heated furnace, or a plasma Flames Use a premix burner (see image!) ○ A fuel, oxidant, and sample are mixed prior to introduction into the flame Process of Sample in premix burner 1. Sample solution is drawn into the pneumatic nebulizer by rapid flow of oxidant past the tip of the sample capillary Nebulizer: this device breaks the liquid sample into a mist of fine droplets; creates aerosol Pneumatic Nebulizer: operates by using a high-pressure gas (typically air, argon, or nitrogen) to atomize a liquid sample. ○ liquid sample is aspirated into the nebulizer by a gas flow (*not all use gas) Sample Capillary: small, typically narrow tube that is immersed in the liquid sample; connected to the nebulizer; allows the liquid sample to be drawn into the nebulizer when a gas (usually air or argon) is introduced at high pressure Aerosal: A fine suspension of liquid (or solid) particles in a gas 2. Liquid breaks into a fine mist as it leaves the capillary The spray is directed against a glass bead and the droplets break into smaller particles (nebulization) 3. The mist, oxidant, and fuel flow past baffles that promote further mixing and block large droplets of liquid 4. Excess liquid collects at the bottom of the spray chamber and flows out to a drain 5. Aerosal reaching the flame has only ~5% of original sample a spray chamber helps to optimize atomization by removing larger droplets ○ Smaller droplets (around 2-5 µm) are more likely to efficiently vaporize in the flame or plasma, improving the efficiency of the atomization process. ○ Nebulizer Droplet Size: The nebulizer initially creates a broad range of droplet sizes, but larger droplets are less effective for atomization. Most common fuel-oxidizer combination use air/acetylene giving temps of 2400 - 2700K ○ Fuel provides the energy necessary for the combustion process and influences the temperature and reducing properties of the flame. ○ Oxidant ensures the proper combustion of the fuel and controls the overall chemical environment within the flame, impacting temperature and the oxidation/reduction conditions that can affect atomization and interference. Using a rich flame (excess fuel) increases sensitivity because excess carbon can reduce the metal oxides and hydroxides Using a lean flame (excess oxidant) gives a hotter flame Flames emit light that must be subtracted from the total signal to get the analyte signal Graphite Furnaces Curved L’vov platform: serves as a support for the liquid sample during the atomization process. Its primary role is to enhance the atomization efficiency by controlling the heat distribution and preventing the loss of sample during the heating process. More sensitive than a flame and requires less sample ○ 1-100 μL of sample is injected into the furnace through a hole at the center Light from a hollow cathode lamp travels through the window at each end of the graphite tube ○ To prevent oxidation, Ar gas is passed over the furnace Process 1. When the sample is injected it should be done on the floor of the furnace If injected too high, the precision can be poor 2. A furnace is used to dry samples at 125°C for 20s 3. Drying is followed by charring at 1400°C to destroy organic matter 4. Samples is then atomized at 2100°C for 10s Absorbance reaches maximum then decreases as atomevaporates from oven 5. After atomization furnace is heated at 2500oC for 3s to clean out remaining residue In direct solid sampling, a solid is analyzed without sample preparation Everything in the sample other than the analyte is called the matrix A matrix modifier is a substance added to the sample to reduce the loss of the analyte during the charring by making the matrix more volatile or the analyte less volatile Figure 1.2. Reduction of interference by using a matrix modifier Transverse Heated Graphite Furance The sample is injected onto the platform, which is heated by radiation from the furnace wall The sample will not be vaporized until the wall reaches a constant temperature Transverse heating provides a uniform temperature over the whole furnace Interference from previous runs, called a memory effect, is reduced in a transversely heated furnace ○ heated transversely (from side to side) to provide nearly uniform temperature over the whole furnace. ○ In furnaces with longitudinal (end-to-end) heating, the center of the furnace is hotter than the ends Comparison Flames vs Furance Plasmas Inductively Coupled Plasma ○ Twice as hot and more stable than the flame ○ Inert Ar environment, along with temperature and stability, eliminates much of the interference seen with flames ○ Simultaneous multi-element analysis ○ Costs more to purchase and operate than the flame ○ Process: 1. To introduce the mist into the torch, liquid sample is pumped slowly into the nebulizer 2. Liquid emerging from the nebulizer is sprayed into a cyclone chamber where coarse particles fall to the bottom 3. Fine mist exiting the chamber goes to the plasma torch, where emission is observed from excited atoms and ions 4. Use an ultrasonic nebulizer The sample is directed onto a oscillating piezoelectric crystal A piezoelectric crystal is one whose dimensions changed in an applied electric field A sinusoidal voltage applied between two faces of the crystal causes it to oscillate The concentration of analyte needed for adequate signal is reduced by an order of magnitude using the ultrasonic nebulizer 5. Analyte reaches the plasma flame as an aerosol of dry, solid particles 6. ICP Consists of two turns of a radio frequency induction coil wrapped around the upper opening of the quartz apparatus 7. High-purity Ar is fed into the plasma gas inlet 8. A spark from a Tesla coil initiates ionization of Ar Creates free e- that are accelerated by the radio frequency field 9. Electrons collide with atoms and transfer their energy to the entire gas 10. The quartz torch is protected from overheating by Ar coolant gas Microwave Plasma ○ Lower cost alternative to ICP ○ A microwave magnetic field forms a plasma using nitrogen extracted from air ○ Sample is nebulized and the aerosol is dried, decomposed, atomized, and excited in the plasma Just incase what I have doesn’t make sense: Chat ICP Process: 1. Sample Introduction: Liquid sample is pumped slowly into the nebulizer. (This is the first step where the liquid sample is introduced into the system.) The sample is directed onto an oscillating piezoelectric crystal (if using an ultrasonic nebulizer). ○ A piezoelectric crystal changes dimensions in an applied electric field. ○ A sinusoidal voltage applied between two faces of the crystal causes it to oscillate. The concentration of analyte needed for adequate signal is reduced by an order of magnitude using the ultrasonic nebulizer. (This helps create a more efficient aerosol for better atomization in the plasma.) 2. Nebulization and Aerosol Creation: Liquid emerging from the nebulizer is sprayed into a cyclone chamber where coarse particles fall to the bottom. Fine mist exiting the chamber goes to the plasma torch. (This is the transition from liquid sample to an aerosol of fine particles, ready for atomization.) 3. Plasma Generation: ICP consists of two turns of a radio frequency induction coil wrapped around the upper opening of the quartz apparatus. (This coil generates the RF field needed to create the plasma.) High-purity Ar (argon) is fed into the plasma gas inlet. (Argon is used as the plasma gas.) A spark from a Tesla coil initiates ionization of Ar, creating free electrons that are accelerated by the RF field. Electrons collide with atoms and transfer their energy to the entire gas, ionizing the gas and maintaining the plasma state. The quartz torch is protected from overheating by the Ar coolant gas. (Argon also serves to cool the torch, preventing damage from the high temperatures of the plasma.) 4. Emission and Detection: Emission is observed from excited atoms and ions within the plasma. (This is the key step where the sample's elements are excited and their emission spectra are recorded for analysis.) Analyte reaches the plasma flame as an aerosol of dry, solid particles. (This refers to the state of the analyte as it is introduced into the plasma, where it undergoes atomization and excitation.) Temperature Affects Atomic Spectroscopy Temperature determines: ○ the degree to which a sample breaks down into atoms ○ the extent to which a given atom is found in its ground, excited, or ionized states. ○ *Each of these effects influences the strength of the signal we observe. Boltzmann Distribution The Boltzmann distribution is a statistical distribution that describes the distribution of energies among the particles (atoms, molecules, etc.) in a system at equilibrium. It is particularly relevant in atomic spectroscopy because it helps explain how the temperature of a sample influences the distribution of atoms between different energy levels, which is crucial for understanding both atomic absorption and atomic emission processes. Consider an atom with energy levels E0 and E* separated by ΔE ○ The figure shows three states at E* and two at E0 ○ Degeneracy: the number of states at each energy An atom (or molecule) may have more than one state at a given energy The figure shows three states at E* and two at E0 Boltzmann distribution: describes the relative populations of different states at thermal equilibrium Effects on Temperature for Absorption vs Emission *This includes Boltzmann information in the sub-bullets Atomic absorption spectroscopy is more acceptable to temperature variation ○ Varying the temperature by 10K hardly affects the ground-state population and would not noticeably affect the signal in atomic absorption We see that more than 99.98% of the sodium atoms are in their ground state at 2600K Atomic emission spectroscopy requires that the flame be very stable, or emission intensity will vary significantly ○ Plasmas are used for atomic emission due to a more stable temperature ○ Atomic emission occurs from the excited state The intensity is proportional to the population of the excited state Therefore 4% increase in the excited state would increase the emission intensity by 4% Instrumentation Principal Differences b/t Atomic & Ordinary Molecular Spectroscopy Light Source (or lack of) Sample Container (flame, furnace, or plasma) The need to subtract background emission Atomic Linewidths The linewidth of the radiation must be narrower than the linewidth of the absorbing sample ○ This must be the case to satisfy Beer’s Law ○ If this is not satisfied, the measured absorbance will not be proportionate to the concentration of the sample The Heisenberg uncertainty principle governs the natural linewidth ○ The shorter the lifetime of the excited state, the more uncertain is its energy ○ According to the Heisenberg uncertainty principle, the shorter the lifetime of the excited state, the broader the spectral line. ○ The natural linewidth is inversely proportional to the lifetime of the excited state, with a broader line corresponding to a shorter lifetime. Heisenberg Uncertainty Principle ○ Two mechanisms cause line-broadening ○ The Doppler Effect ○ Pressure Broadening The Doppler Effect ○ An atom moving toward the radiation source experiences more oscillations of the electromagnetic wave in a given time period than one moving away from the source ○ In the laboratory frame of reference, the atom moving toward the source absorbs lower frequency light than that absorbed by the one moving away ○ ○ Effect on Spectrum: Atoms moving towards the light source will experience a blue shift (increase in frequency) Atoms moving away will experience a red shift (decrease in frequency) This leads to a broader spectral line, as different atoms in the sample contribute to the absorption or emission at slightly different frequencies. ○ Impact: Doppler broadening increases with temperature, and at high temperatures, it becomes a significant contributor to the overall linewidth. Pressure Broadening ○ Caused by collisions between atoms As the pressure increases, the frequency of collisions between particles increases, leading to a broadening of the spectral lines. ○ Collisions shorten the lifetime of the excited state ○ Uncertainty in the frequency of atomic absorption and emission lines is roughly numerically equal to the collision frequency between atoms and is proportional to pressure ○ Unlike Doppler broadening, which is temperature-dependent, pressure broadening is primarily dependent on gas density or pressure. Hollow Cathode Lamp To produce narrow lines of the correct frequency, we use a hollow-cathode lamp containing a vapor of the same element as that being analyzed The cathode is made of the element whose emission lines are desired When a voltage is applied between the anode and cathode, gas is ionized ○ Positive ions are accelerated toward the cathode After ionization occurs, the lamp is maintained at a constant current by a lower voltage Cations strike the cathode with enough energy to generate metal atoms from the cathode into the gas phase Gaseous atoms excited by collisions with high-energy electrons emit photons This atomic radiation has the same frequency absorbed by analyte in the flame or furnace ○ Aka the light emitted by the hollow-cathode lamp matches the absorption wavelengths of the analyte atoms in the flame or furnace during atomic spectroscopy. Lamp emission is sufficiently narrower than the width of the absorption line of atoms in the flame – nearly monochromatic ○ The purpose of a monochromator in atomic spectroscopy is to select one line from the lamp and to reject excess emission from the flame or furnace A different lamp is usually required for each element Multi-Element Detection: Atomic Emission ICP or microwave plasma spectrometers do not require lamps and can detect many elements at the same time Entering emission is reflected by a collimating mirror, dispersed in the vertical plane by a prism, and then dispersed in the horizontal plane by a grating Dispersed radiation lands on a charge injection device (CID) detector Charged Injection Device ○ Each pixel of a CID detector can be read individually at any time ○ If the pixel is read, the charge in the pixel is then neutralized to reset the pixel to zero ○ The pixel can then accumulate more charge and be read several times while other pixels are filling up at a slower pace ○ This process increases the dynamic range of the detector ○ *Strong signals in one pixel are less prone to spill over into neighboring pixels Background Correction The aim of background correction is to separate the signal corresponding to the analyte (the element of interest) from any interfering signals, improving the precision and reliability of the results. Scattering Correction ○ aims to account for this unwanted scattered light so that only the signal due to analyte absorption is measured. ○ If scattering is not corrected, it could lead to an overestimation of the analyte’s concentration. ○ Scattering occurs when light interacts with particles in the sample, causing the light to change direction; result from particles in the sample (e.g., droplets in the flame or furnace, dust, or aerosols) that do not absorb the light but reflect or scatter it Deuterium Lamps ○ used as a background light source for background correction. the light from the deuterium lamp does not overlap with the wavelengths of interest for the analyte. Therefore, useful for background correction because any absorption due to the sample can be compared to the deuterium lamp’s emission, which will include scattered light but no analyte absorption. ○ Process: 1. Broad emission from a D2 lamp is passed through the flame in alternation with that from the hollow cathode 2. Light from the hollow cathode lamp is absorbed by the analyte as well as absorbed and scattered by the background 3. Light from the D2 lamp is only absorbed and scattered by the background 4. The difference between the two is the absorbance of the analyte Zeeman Correction ○ A magnetic field applied parallel to the light path through the furnace that splits the analyte signal (aka analyte’s absorption lines) into three components One is shifted to a lower wavelength One is shifted higher One is not shifted ○ The strong magnetic field is pulsed on and off Sample and background are observed when the field is off Ie the absorption lines are not split Only background is observed when the field is on Ie the absorption lines are split The difference between them is the corrected signal any background absorption (from scattering or other non-analyte components) will be uniform across the spectrum and not split like the analyte’s absorption lines. Detection Limits Concentration of an element that gives a signal equal to three times the standard deviation of signal from a blank Interference Interference is any effect that changes the signal while analyte concentration remains unchanged Four types of interference: ○ Spectral ○ Physical ○ Chemical ○ Ionization Spectral Interference Unwanted (other element/molecules) signals overlapping with the analyte signal Signal from the flame can be corrected with D2 or Zeeman Can also utilize a different wavelength for analysis Use a high resolution spectrometer Physical Interference A component of the sample altering nebulization and transport of analyte Peristaltic pumps are used to correct by ensureing steadier solution flow to the flame or plasma Chemical Interference A component of the sample altering the chemical processes involved in atomization of analyte Chemical reactions decreasing the concentration of analyte Corrections ○ Releasing agents Chemicals added to a sample to decrease chemical interference ○ A fuel-rich flame Reduces certain oxides that hinder atomization ○ Higher flame temperatures Eliminates many kinds of chemical interference Ionization Interference A problem in the analysis of samples with low ionization potentials ○ Ions have energy levels different from those of neutral atoms, so the neutral atom concentration and signal is decreased Correction ○ An ionization suppressor decreases the extent of ionization of analyte ICP-MS Inductively Coupled Plasma-Mass Spectrometry Chapter 21-6 Remember ICP used an Ar plasma ○ This is because the ionization energy is higher than almost all other elements ○ Analyte elements are ionized by colliding with Ar+, excited Ar atoms, or electrons The Ar plasma can be directed towards a Mass Spectrometer ○ MS separates ions by their mass to charge ratio ○ Allows for a wide range of elements to be analyzed (bulk to trace) Disadvantages: Need for high vacuum ○ Need to avoid collisions between analyte ions and gas molecules (background) ○ Use a collision cell or dynamic reaction cell to remove isobaric interference ○ Isobaric interferences are when the interfering species has the same mass-to-charge ratio as the analyte ion Very low detection limit ○ It is important to minimize any contamination Dust, skin, hair, jewelry, cosmetics, sample containers & reagents ○ Wear PPE Subject to matrix effects and drift ○ An internal standard with nearly the same ionization energy as analyte corrects for both Internal standards with just one major isotope should be selected for maximum response Atomic Isobaric Interference ○ Isotopes of other elements or polyatomic species that have the same mass-to-charge ratio as analyte ○ Example: 64Zn and 64Ni will both give peaks at m/z 64 ○ ○ It is possible to mathematically correct for isobaric interferences by using known abundances of isotopes Collision and Dynamic Reaction Cells Used to correct for isobaric interferences Collision cells ○ Low pressure of inert gas such as He or N2 ○ Fast-moving ions from the plasma collide with the inert gas decreasing the speed of the plasma ions Dynamic reaction cells ○ Low pressure of a reactive gas such as NH3, CH4, N2O, CO, O2 ○ Uses thermodynamically favorable reactions to reduce interference ○ Electric field of the reactive gas is configured to select specific ion masses to pass through the cell Summary: ○ Collision cell neutral gas slows polyatomic interferents for kinetic energy discrimination ○ Dynamic reaction cell reaction with added gas removes interferents while leaving analyte signal unaffected X-Ray Spectroscopy X-Ray Fluorescence Chapter 21-8 X-rays are high-energy photons generated when high-energy electrons (10 to 50kV) are accelerated into an anode X-ray fluorescence is the emission of X-rays following the absorption of X-rays by a material Each element that is present emits characteristic X-ray peaks Elements are identified by their peak energies and quantified by the number of photons in each peak X-rays are generated in four ways: ○ Bombardment of a metal target with a beam of high energy electrons ○ Exposure of a substance to a primary beam of X-rays Generates secondary X-ray fluorescence ○ Use of a radioactive source where decay produces X-rays ○ From a synchrotron radiation source X-rays are generated by the transition of electrons within an atom ○ Atoms are structured with the nucleus centered around shells of electrons ○ These shells are names K, L, M, N (now known as 1s, 2s, 2p, etc.) ○ When an electron is ejected from an inner shell by some radiation source a hole is formed An outer shell electron will fill the hole X-rays nomenclature is important when interpreting a spectrum ○ Electrons ejected from a shell will be named after that shell ○ Ejected K electrons will be called “K” Further distinctions are defined as ⍺, β, or ɣ ○ These are assigned based on where the electron is coming from to fill the hole ○ Example: K hole filled with L electron is K⍺ where filled with an M electron is Kβ These transitions are unique to each element The energies are independent to the chemical form of the element ○ Iron will always have the same energy transitions Instrumentation Sources X-ray Tube ○ Contains a W filament with a very strong metal anode ○ Under vacuum ○ Converts electrical input into a beam of x-rays Electron Gun (W or LaB6 filament) ○ Heated filament under vacuum produces a beam of electrons ○ Typical for SEM ○ Detectors Photon Counting: pulses of a charge are absorbed and counted For Energy Dispersive instruments there is no monochromator needed SiLi: pn junction needs to be cooled by liquid N2; when an X-ray photon passes through, it causes a swarm of electron-hole pairs to form, and this causes a voltage pulse Silicon Drift: measure the energy and quantity of incoming photons by the amount of ionization it produces in the detector material Scanning Electron Microscopy Can perform energy dispersive X-ray fluorescence microanalysis Uses EDS as a detector Incident energetic electrons in the form of a beam knock out electrons from K or L shells Elemental composition is determined with small spatial resolution from a specific location of a sample Energy Dispersive X-Ray Spectrometer X-ray tube, sample holder, detector No monochromator needed Nondestructive of samples Low limits of detection (except for lighter elements) No sample preparation (except mounting) Minimal run times Precautions should be taken to minimize exposure when using x-rays Wavelength Dispersive X-ray Fluorescence Source, monochromator, detector Single channel vs. multichannel Disperse X-ray fluorescence using Bragg diffraction Provide greater spectral resolution over EDS Can detect lighter elements Requires a more powerful x-ray source Data Interpretation To identify and element, always verify that all appropriate K, L, and/or M peaks are observed at the proper intensity ratio Artifacts might be present in the spectrum that cause difficulty in interpreting what elements are present ○ Bremsstrahlung Like a background – it is from the continuous spectrum of x-rays from the x-ray tube Large broad peak that can mask some analyte peaks Artifacts ○ Sum Peaks When two photons from with the same energy strike the detector at the same time – the energies are added ○ Escape Peaks When the Si in the detector absorbs Si K⍺ energy and shifts the peak lower by 1.74keV X-Ray Diffraction When a sample is radiated with X-Ray radiation, scattering occurs When X-rays are scattered by the ordered environment of a crystal (crystal structure), scattering is either constructive or destructive ○ The result is Diffraction X-ray diffraction provides qualitative identification of crystalline compounds ○ Determines structures of steroids, vitamins, antibiotics, etc. Bragg’s Law When X-ray beam strikes a crystal at angle θ, part of the beam is scattered by the layer of atoms at the surface Any x-rays that are not scattered will penetrate farther into the crystal scattering as it goes The cumulative effect of the scattering from the layers is similar to a diffraction grating For diffraction to occur… ○ The spacing between crystal layers must be the same as the wavelength of radiation ○ The scattering centers must be consistently spatially distributed Instrumentation Sample Preparation Sample is ground to a fine homogenous powder Sample holders typically contain some sort of depression or cavity to mount the sample and then covered ○ Can be rotated Source: X ray tube Detector: photographic method Cylindrical camera holds a strip of film where each set of lines produced represents a diffraction from the crystal planes Guide to Atomic Spectrometry Technique Selection Analytical Separations Chapter 23 Solvent Extraction Extraction is the transfer of a solute from one phase to another The goal is to isolate or concentrate the desired analyte ○ Separates analytes from species that would interfere with its analysis The most common extraction is using two immiscible liquids ○ Aqueous (typically water, highly polar) and organic liquids form two layers ○ The organic liquid (low polarity) will either sit on top or on bottom of the aqueous layer depending on its density ○ Two liquids are miscible if they form a single phase(layer) when mixed in any ratio ○ Immiscible liquids remain in separate phases (layers) Extraction relies on the solubility of a compound in different phases ○ Based on the principle of “Like dissolves like” ○ Solute is more soluble in a phase whose polarity is similar to that of the solute (hyrophobic/hydrophilic) pH Effects If the solute is an acid or base, its charge changes as the pH is changed A neutral species is usually more soluble in organic solvent where charged species are more soluble in aqueous solution To extract a base into water, use a pH low enough to convert B into BH+ To extract the acid into water, use a pH high enough to convert into HA into A- Metal Chelators One way that metals can be separated from one another is to selectively complex one ion with an organic liquid and extract it into an organic solvent Ligands often used are weak acids which lose a proton when they bind to a metal ion Each ligand can react with many different metal ions, but some selectivity is achieved by controlling the pH Chromatography Chromatography operates on the same principle as extraction There are two phases ○ Stationary Phase Viscous liquid Attached to a column ○ Mobile Phase Liquid or Gas Sample and mobile phase entering the column is eluent and leaving is the eluate Columns: either packed or open tubular ○ Packed columns have the stationary phase filled in to the column ○ Open tubular have the stationary phase coated on the inner wall More common + efficient The main principle of chromatography is the interaction of a sample with the stationary phase The type of interaction dictates the type of chromatography ○ Adsorption Chromatography ○ Partition Chromatography ○ Ion-Exchange Chromatography ○ Size Exclusion Chromatography ○ Affinity Chromatography Adsorption Chromatography Solid stationary phase Gas or liquid mobile phase Solute (sample) is adsorbed onto the stationary phase Partition Chromatography Liquid stationary phase Gas or liquid mobile phase Solute equilibrates between the stationary and the mobile phases Ion exchange chromatography Solid stationary phase (resin) Liquid mobile phase Anions or cations are covalently attached to the stationary phase Size Exclusion Chromatography Porous gel stationary phase Gas or liquid mobile phase There is no attractive interaction between the stationary phase and the solute (size only) ○ Large samples don’t “fit” in the pores Affinity chromatography An immobilized molecule stationary phase Gas or liquid mobile phase Solute molecules binds specifically to the stationary phase When a mixture is passed through the column, only the one that interacts with the immobilized antibody is retained by the column Plumber’s View The speed of the mobile phase passing through a chromatography column is expressed either as a volume flow rate or as a linear velocity ○ Flow Rate Volume of solvent per unit time traveling through column, F ○ Linear Velocity Distance per unit time traveled by solvent, ux Linear velocity is determined by dividing the column length by tm ux = L/tm Chromatogram ○ Data graph produced from a chromatography experiment ○ Signal (detector) response as a function of time (minutes) Retention time, tR ○ The time it takes a sample to elute through a stationary phase and strike the detector ○ *True retention time needs to be adjusted Mobile phase or an unretained solute travels through the column in the minimum possible time, tm t’R = tR - tm ○ Retention volume, VR ○ The volume of mobile phase required to elute a particular solute from the column ○ *F= Volume of solvent per unit time traveling through column Since Volume is proportional to Time: ○ Chromatography can be used in two ways: ○ Analytical To separate and identify components ○ Preparative To separate and purify a mixture Efficiency of Separation Good chromatography can be observed by looking at the peaks in the chromatogram Resolution: Are the peaks able to be differentiated (separated)? Diffusion: Are the peaks spreading and becoming broader/wider? Resolution Solute eluting from the column will produce a Gaussian peak in the chromatogram The peak width is typically measured at ½ height (w½) or at the baseline (w) ○ This width is important when measuring the resolution ○ Where N is the Number of plates ○ L is column length ○ K= retention factor ○ alpha= separation factor Diffusion Where molecules move randomly from a region of high concentration to a region of low concentration Causes solute to move in and out of phase that could take longer to reach the detector Theory Behind: ○ Bands broaden as a solute moves through the column ○ A narrow Gaussian peak is desired ○ There are circumstances where the peak will broaden, and even become asymmetrical Column Efficiency: Plate Height Why Bands Spread Band spreading is an inevitable process in chromatography The total spreading is a sum of all variances during the chromatographic run Spreading (or broadening) occurs due to: ○ Injection volume ○ Detection volume ○ Connecting tubing – flow in the tubing decreases near the walls Plate Height Equation Plate height is proportional to the variance (STDEV) Van Deemter equation how the column and linear velocity affect H Where is the 𝜇𝑥 is linear velocity and A, B, and C are constants for a given column and stationary phase The type of column will change the inclusion of the Van Deemter factors ○ Packed Columns: A, B, C≠ 0 ○ Open Tubular column: A=0 ○ Capillary electrophoresis: A=C=0 Longitudinal Diffusion (B/ 𝜇𝑥) Occurs while the band is transported along the column by solvent flow Bands slowly broaden as molecules diffuse from the high concentration within the band to regions of lower concentration Higher linear flow decreases longitudinal diffusion Gas mobile phase is faster than liquid mobile phase Mass Transfer (C𝜇𝑥) Finite time available for solute to move between the mobile and stationary phases Solute must diffuse from the mobile phase to the surface of the stationary phase for equilibration to occur Increasing temperature decreases the mass transfer factor Multiple Flow Paths (A) Also called the Eddy Diffusion term Sample flow paths can vary in “length” Molecules entering the column at the same time on the left are eluted at different times on the right Column Type Open Tubular ○ Column of choice ○ Advantage ○ Higher resolution Reduced plate height (increased plate number) Shorter analysis time Increased sensitivity ○ Disadvantage: Lower sample capacity Packed ○ Do not allow for fast linear velocity Asymmetrical Peaks Gaussian peak shape results when the distribution constant (KD) is independent of the concentration of solute ○ Theoretically: KD is independent of concentration ○ Reality: KD changes as the concentration of solute increases Causes a skewed chromatographic peak Typically fronting or tailing, but can also be splitting A graph of Cs versus Cm at a given temperature is called an isotherm ○ Fronting and tailing is usually a result from overloading the column ○ Fronting ○ ○ There is a gradual rise and abrupt fall of the peak ○ Increasing sample concentration causes the sample to be more soluble in the stationary phase ○ The severity of fronting increases with the amount of excess solute injected Tailing ○ ○ The peak jumps up quickly and then gradually falls ○ If the column has limited retention capacity, a concentrated sample will saturate the stationary phase ○ Saturation causes the peak maximum to shift to a shorter retention time ○ The shift forward (shorter) in retention time increases with increased overload Thin Layer Chromatography Considered a method of planar chromatography Utilizes a material that is coated onto glass, plastic, or metal plate → Stationary Phase Liquid mobile phase is moved up the plate by capillary action TLC is faster, better resolution, and more sensitive than paper chromatography TLC is similar to HPLC ○ HPLC can be automated Plates Typically uses glass plates with a thin stationary layer ○ Divided particles – silica gel ○ Plates come in varying sizes Conventional vs. high performance ○ Conventional phase thickness is ~200-250μm with a particle size of 20μm ○ High performance phase thickness is ~100μm with a particle size of 5μm HP plates are faster and have better resolution but have smaller sample capacity Sample Application Samples are placed onto the plate in a “spot” This spot is ~1-2cm above the edge of the plate Spot size should be less than 5mm ○ Smaller for quantitative analysis Application is done manually by placing a capillary tube into the sample and then onto the plate A line in pencil should be drawn to place the sample spot The sample solvent should be evaporated Plate Development Elution for TLC – liquid MP travels up the SP Plate is placed into a closed tank/chamber that has had time to equilibrate Saturated with vapors of the solvent system but still having the MP liquid present *The sample should not be immersed into the MP The MP should travel ~2/3 up the plate The plate is then dried and ready for processing Processing Plates To view the separated sample, the TLC plate needs to be developed Different techniques: ○ Sulfuric acid spray ○ Iodine ○ Dying reagents (e.g. ninhydrin) ○ UV light (long wave and short wave) Need fluorescent SP Applications Qualitative TLC Rf data is not sufficient to conduct an identification ○ There are many samples that might have the same value ○ The Rf can be varied based on concentration, the TLC plate, and the development conditions TLC can be helpful if run with known standards and the Rf can be compared TLC plates can be scraped and then analyzed using another technique to provide qualitative information Two-planar development – TLC plate processed in two dimensions (x and y) ○ The plate is developed in solvent A then rotated 90° and developed in solvent B ○ Solvent Systems The ratio of solvents can affect the results ○ To increase the Rf value, increase the polarity of the solvent or increase the ratio of the polar solvent Some common solvents used in TLC include: ○ Hexanes (or petroleum ethers/ligroin) ○ Diethyl ether ○ Acetone (a ketone) ○ Ethyl acetate (an ester) ○ Alcohols (methanol, ethanol) ○ Acetic acid (a carboxylic acid) Solvent systems ○ Polar compounds: 100% EtOAc or 5% MeOH/dichloromethane ○ Normal compounds: 10-50% EtOAc/Hexane ○ Nonpolar compounds: 5% EtOAc/hexane, 5% ether/hexane, 100% hexane Gas Chromatography Separation Process in Gas Chromatography Gaseous analyte (sample) is transported through the column by a gaseous mobile phase Gas-liquid partition chromatography ○ The stationary phase is a nonvolatile liquid-like polymer bonded to the inside of the column or to a fine solid support Gas-solid adsorption chromatography ○ Analyte is adsorbed directly on solid particles of stationary phase Process: 1. The sample is injected through the heated injection port which consists of a rubber septum where is quickly vaporizes 2. The vapor is collected by the carrier gas (mobile phase) into the column 3. Separation occurs in the column and the separated components are eluted into the detector Retention Nonpolar compounds are eluted in order of increasing boiling point from nonpolar and polar stationary phases ○ A principal determinant of retention is the volatility of the solutes ○ Analytes may be introduced below their boiling point, and only partly vaporize Strongly polar stationary phase retains the strongly polar solutes and vice versa Increases with boiling point for compounds of the same chemical class (homologous series) Greater retention also results from “like dissolves like” ○ Alcohols are more retained relative to alkanes on the polar Retention index (I) provides a scale of relative retention that remains constant for all columns with the same stationary phase during isothermal analysis ○ For a linear alkane, the retention index equals 100x the number of carbon atoms e.g. octane ≡ 800 Instrumentation Four main parts to a Gas Chromatograph ○ Injection Port ○ Oven ○ Column ○ Detector ○ Readout Column Two Types of columns ○ Open tubular ○ Packed Packed column Column filled with solid stationary phase particles Filled with fine particles of solid support coated with a nonvolatile liquid or the solid itself Used for preparative separations Advantage: ○ Greater sample capacity Disadvantage: ○ Broader peaks ○ Longer retention times ○ Less resolution Packed Column Parameters ○ Diameters: 2-4mm ○ Lengths: 1-5m ○ Stationary Phase (SP) particle size (mesh size): 100-200 Open Tubular Typically used over packed Advantages: ○ Higher resolution ○ Shorter analysis time ○ Greater sensitivity Disadvantge: ○ Lower sample capacity Types ○ Wall-coated tubular column Liquid stationary phase on inside wall of column Most common GC column ○ Porous-layer tubular column Solid stationary phase particles on inside wall of column OT Column Parameter ○ Diameters: 0.10-0.53mm ○ Lengths: 10-30m ○ SP Thickness: ~0.25𝜇m Narrow, long, thicker stationary phase columns provide better resolution, but less sample capacity and longer tr The choice of liquid stationary phase (for OT Column) is based on the rule “like dissolves like” Nonpolar columns are best for nonpolar solutes Columns of intermediate polarity are best for intermediate polarity solutes Polar columns are best for strongly polar solutes Chiral (optically active) bonded phases for separating optical isomers Packed column Column filled with solid stationary phase particles Temperature Programing The temperature of the column (oven) is raised during the separation to increase analyte vapor pressure and decrease retention times of late-eluting components ○ Better and faster separation General elution problem ○ The inability of an isothermal column temperature to provide separation for samples containing compounds with very different boiling points with adequate retention time The initial temperature is far below the atmospheric- pressure boiling temperature of most compounds to be strongly retained at the beginning of the column Once the column temperature is high enough for a compound to have enough vapor pressure, it starts moving down the column The temperature continues to increase and the compound becomes less retained and emerges as a sharp peak Initial temperature is set for a certain amount of time Rate of increase is some °C per minute until the final temperature is reached ○ Final temperature is set by the analyst Pressure Programing GC instruments can control the pressure of the carrier gas to keep flow rate or linear velocity constant Increasing the inlet pressure increases the flow of mobile phase and decreases retention time Carrier Gas Helium is the most common He, N2, and H2 give similar plate heights Optimal velocity depends on column diameter and stationary phase, but always increases in the order N2