Module 16 Basic Concepts of Sensation and Perception PDF
Document Details
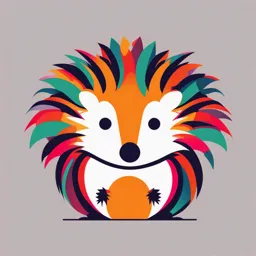
Uploaded by DarlingCarnation
Tags
Summary
This document is a psychology module about basic concepts of sensation and perception. It explains the processes of bottom-up and top-down processing, selective attention, and inattentional blindness. It explores how these concepts relate to real-world situations and provides practical examples to illustrate the discussed concepts.
Full Transcript
Module 16 Basic Concepts of Sensation and Perception LEARNING TARGETS 16-1 Describe sensation and perception, and explain the difference between bottom-up processing and top-down processing. 16-2 Discuss how selective attention directs our perceptions. 16-3 Describe the thr...
Module 16 Basic Concepts of Sensation and Perception LEARNING TARGETS 16-1 Describe sensation and perception, and explain the difference between bottom-up processing and top-down processing. 16-2 Discuss how selective attention directs our perceptions. 16-3 Describe the three steps that are basic to all our sensory systems. 16-4 Discuss the difference between absolute thresholds and difference thresholds. 16-5 Discuss how we are affected by subliminal stimuli. 16-6 Explain the function of sensory adaptation. How do we create meaning from the blizzard of sensory stimuli that bombards our bodies 24 hours a day? Meanwhile, in a silent, cushioned, inner world, our brain floats in utter darkness. By itself, it sees nothing. It hears nothing. It feels nothing. So, how does the world out there get in? To phrase the question scientifically: How do we construct our representations of the external world? How do a campfire’s flicker, crackle, and smoky scent activate neural connections? And how, from this living neurochemistry, do we create our conscious experience of the fire’s motion and temperature, its aroma and beauty? In search of answers, let’s examine the basics of sensation and perception. 458 Processing Sensation and Perception 16-1 What are sensation and perception? What do we mean by bottom-up processing and top-down processing? Flip It Video: Top-Down and Bottom-Up Processing Heather Sellers’ curious mix of “perfect vision” and face blindness illustrates the distinction between sensation and perception. When she looks at a friend, her sensation is normal. Her sensory receptors detect the same information yours would, and her nervous system transmits that information to her brain. Her perception—the processes by which her brain organizes and interprets sensory input—is almost normal. Thus, she may recognize people from their hair, gait, voice, or particular physique, just not from their face. Her experience is much like the struggle any human would have trying to recognize a specific penguin. sensation the process by which our sensory receptors and nervous system receive and represent stimulus energies from our environment. sensory receptors sensory nerve endings that respond to stimuli. perception the process of organizing and interpreting sensory information, enabling us to recognize meaningful objects and events. Under normal circumstances, sensation and perception blend into one continuous process. In this module, we slow down that process to study its parts; in real life, your sensory and perceptual processes work together to help you decipher the world around you. Bottom-up processing starts at your sensory receptors and works up to 459 higher levels of processing. Top-down processing constructs perceptions from this sensory input by drawing on your experience and expectations. bottom-up processing analysis that begins with the sensory receptors and works up to the brain’s integration of sensory information. top-down processing information processing guided by higher-level mental processes, as when we construct perceptions drawing on our experience and expectations. As your brain absorbs the information in Figure 16.1, bottom-up processing enables your sensory systems to detect the lines, angles, and colors that form the flower and leaves. Using top-down processing, you interpret what your senses detect. Figure 16.1 What’s going on here? Our sensory and perceptual processes work together to help us sort 460 out complex images, including the hidden couple in Sandro Del- Prete’s drawing, The Flowering of Love. 461 Selective Attention 16-2 How does selective attention direct our perceptions? Through selective attention, our awareness focuses, like a flashlight beam, on a minute aspect of all that we experience. We may think we can fully attend to a conversation or a class lecture while checking and returning text messages. Actually, our consciousness focuses on but one thing at a time. selective attention the focusing of conscious awareness on a particular stimulus. By one estimate, our five senses take in 11,000,000 bits of information per second, of which we consciously process about 40 (Wilson, 2002). Yet our mind’s unconscious track intuitively makes great use of the other 10,999,960 bits. What captures our limited attention? Things we deem important. A classic example of selective attention is the cocktail party effect—your ability to attend to one voice among a sea of other voices. But what happens when another voice speaks your name? Your cognitive radar, operating on your mind’s other track (and in your right frontal cortex), instantly brings that unattended voice into consciousness (Demeter & Woldorff, 2016). This effect might have prevented an embarrassing and dangerous situation in 2009, when two Northwest Airlines pilots “lost track of time.” Focused on their laptops and in conversation, they ignored alarmed air traffic controllers’ attempts to reach them and overflew their Minneapolis destination by 150 miles. If only the controllers had known and spoken the pilots’ names. Selective Attention and Accidents Text, attend to your musical selections, or chat on your phone while 462 driving, and your selective attention will shift back and forth between the road and its electronic competition. Indeed, our attention shifts more often than we realize. One study left people in a room for 28 minutes with full Internet and television access. On average, they guessed their attention switched 15 times. But they were not even close. Eye-tracking revealed eight times that many attentional switches—120 in all (Brasel & Gips, 2011). Rapid toggling between activities is today’s great enemy of sustained, focused attention. When we switch attentional gears, especially when we shift to complex tasks like noticing and avoiding cars around us, we pay a toll—a slight and sometimes fatal delay in coping (Rubenstein et al., 2001). When a driver attends to a conversation, activity in brain areas vital to driving decreases an average of 37 percent (Just et al., 2008). AP® EXAM TIP You may wish to think about how the information on selective attention relates to something a little less dangerous: studying. The same principles apply. The more time you spend texting, tweeting, and social networking, the less focused you’ll be on the material you’re trying to master. A better strategy is to spend 25 minutes doing schoolwork and schoolwork alone. Then you can reward yourself with a few minutes of online time. Texting or phone chatting—something 1 in 4 drivers admit doing— accompanies about 28 percent of traffic accidents (NSC, 2010; Pew, 2011). One video cam study of teen drivers found that driver distraction from passengers or phones occurred right before 58 percent of their crashes (AAA, 2015). Talking with passengers raises the risk of an accident 1.6 times above normal. Using a cell phone (even a hands-free set) carries a risk 4 times higher than normal—equal to the risk of drunk driving (McEvoy et al., 2005, 2007). And while talking is distracting, texting wins the danger game. One 18-month video cam study tracked the 463 driving habits of long-haul truckers. When they were texting, their risk of a collision increased 23 times (Olson et al., 2009)! Many European countries and most American states and Canadian provinces now ban texting while driving (CBC, 2014; Rosenthal, 2009). So the next time you’re behind the wheel, put the brakes on your texts. Your passengers, fellow drivers, and selective attention will thank you. “I wasn’t texting. I was building this ship in a bottle.” Driven to distraction In driving-simulation experiments, people whose attention is diverted by cell-phone conversation make more driving errors. Inattentional Blindness At the level of conscious awareness, we are “blind” to all but a tiny sliver of visual stimuli. Ulric Neisser (1979) and Robert Becklen and Daniel Cervone (1983) demonstrated this inattentional blindness dramatically by 464 showing people a one-minute video of basketball players, three in black shirts and three in white shirts, tossing a ball. Researchers told the viewers to press a key every time they saw a black-shirted player pass the ball. Most viewers were so intent on their task that they failed to notice a young woman carrying an umbrella saunter across the screen midway through the video (Figure 16.2). Watching a replay of the video, viewers were astonished to see her (Mack & Rock, 2000). This inattentional blindness is a by-product of what we are really good at: focusing attention on some part of our environment. inattentional blindness failing to see visible objects when our attention is directed elsewhere. Figure 16.2 Selective inattention Viewers who were attending to basketball tosses among the black- shirted players usually failed to notice the umbrella-toting woman sauntering across the screen (Neisser, 1979). In a repeat of the experiment, smart-aleck researchers sent a gorilla- suited assistant through the swirl of players (Simons & Chabris, 1999). During its 5- to 9-second cameo appearance, the gorilla paused and thumped its chest. But the gorilla did not steal the show: Half the conscientious pass-counting viewers failed to see it. Psychologists like to 465 have fun and have continued to do so with invisible gorillas. One study of “inattentional deafness” delivered, separately to each ear, a recording of men talking and of women talking. When volunteers were assigned to pay attention to the women, 70 percent failed to hear one of the men saying, over and over for 19 seconds, “I’m a gorilla” (Dalton & Fraenkel, 2012). And when 24 radiologists were looking for cancer nodules in lung scans, 20 of them missed the gorilla superimposed in the upper right of Figure 16.3—though, to their credit, their focus enabled them to spot the much tinier cancer tissue (Drew et al., 2013). The serious point to this psychological mischief: Attention is powerfully selective. Your conscious mind is in one place at a time. Figure 16.3 The invisible gorilla strikes again When repeatedly exposed to the gorilla in the upper right while searching for much tinier cancer nodules, radiologists usually failed to see it (Drew et al., 2013). Given that most people miss someone in a gorilla suit while their attention is riveted elsewhere, imagine the fun that others can have by manipulating our selective attention. Misdirect people’s attention and they will miss the hand slipping into the pocket. “Every time you perform a magic trick, you’re engaging in experimental psychology,” says magician Teller, a master of mind-messing methods (2009). Clever thieves know this, too. One Swedish psychologist was surprised in Stockholm by a 466 woman exposing herself; only later did he realize that he had been pickpocketed, outwitted by thieves who understood the power of selective inattention (Gallace, 2012). In other experiments, people exhibited a form of inattentional blindness called change blindness. In laboratory experiments, viewers failed to notice that, after a brief visual interruption, a big Coke bottle had disappeared, a railing had risen, clothing had changed color—and construction workers had changed places (Figure 16.4). (Chabris & Simons, 2010; Resnick et al., 1997). Out of sight, out of mind. change blindness failing to notice changes in the environment; a form of inattentional blindness. Figure 16.4 Change blindness While a man (in red) provides directions to a construction worker, two experimenters rudely pass between them carrying a door. During this interruption, the original worker switches places with another person wearing different-colored clothing. Most people, focused on their direction giving, do not notice the switch (Simons & Levin, 1998). A Swedish research team discovered that people’s inattentional blindness extends to their own choices. Petter Johansson and his colleagues (2005, 2014) showed 120 volunteers two female faces and asked which face was more attractive. After putting both photos face 467 down, they handed viewers the one chosen and invited them to explain why they preferred it. But on 3 of 15 occasions, the researchers secretly switched the photos—showing viewers the face they had not chosen (Figure 16.5). People noticed the switch only 13 percent of the time, and readily explained why they preferred the face they had actually rejected. “I chose her because she smiled,” said one person (after picking the solemn- faced one). Asked later whether they would notice such a switch in a “hypothetical experiment,” 84 percent insisted they would. Figure 16.5 Choice blindness Petter Johansson, Lars Hall, and others (2005) invited people to choose preferred faces. On occasion, they asked people to explain their preference for the unchosen photo. Most—failing to notice the switch—readily did so. Change deafness can also occur. In one experiment, 40 percent of people focused on repeating a list of words that someone spoke failed to notice a change in the person speaking (Vitevitch, 2003). In two follow-up phone interview experiments, only 2 of 40 people noticed that the female interviewer changed after the third question—a change that was noticeable if people were forewarned of a possible interviewer change (Fenn et al., 2011). Some stimuli, however, are so powerful, so strikingly distinct, that 468 we experience popout. For example, when the female phone interviewer changed to a male interviewer, virtually everyone noticed. 469 Transduction 16-3 What three steps are basic to all our sensory systems? Every second of every day, our sensory systems perform an amazing feat: They convert one form of energy into another. Vision processes light energy. Hearing processes sound waves. All our senses receive sensory stimulation, often using specialized receptor cells. transform that stimulation into neural impulses. deliver the neural information to our brain. The process of converting one form of energy into another form that our brain can use is called transduction. Transduction is rather like translation—of a physical energy such as light waves into the brain’s electrochemical language. Later in this unit, we’ll focus on individual sensory systems. How do we see? Hear? Feel pain? Taste? Smell? Keep our balance? In each case, one of our sensory systems receives, transforms, and delivers the information to our brain. The field of psychophysics studies the relationships between the physical energy we can detect and its effects on our psychological experiences. transduction conversion of one form of energy into another. In sensation, the transforming of stimulus energies, such as sights, sounds, and smells, into neural impulses our brain can interpret. psychophysics the study of relationships between the physical characteristics of stimuli, such as their intensity, and our psychological experience of them. Let’s explore some strengths and weaknesses in our ability to detect and interpret stimuli in the vast sea of energy around us. 470 Thresholds 16-4 How do absolute thresholds and difference thresholds differ? At this moment, we are being struck by X-rays and radio waves, ultraviolet and infrared light, and sound waves of very high and very low frequencies. To all of these we are blind and deaf. Other animals with differing needs detect a world that lies beyond our experience. Migrating birds stay on course aided by an internal magnetic compass. Bats and dolphins locate prey using sonar, bouncing echoing sound off objects. Bees navigate on cloudy days by detecting invisible (to us) polarized light. Our senses open the shades just a crack, allowing us a restricted awareness of this vast sea of energy. But for our needs, this is enough. TRY THIS Try out this old riddle on a couple of friends. “You’re driving a bus with 12 passengers. At your first stop, 6 passengers get off. At the second stop, 3 get off. At the third stop, 2 more get off but 3 new people get on. What color are the bus driver’s eyes?” Do your friends detect the signal—who is the bus driver?—amid the accompanying noise? Absolute Thresholds Flip It Video: Signal Detection Theory To some kinds of stimuli we are exquisitely sensitive. Standing atop a mountain on an utterly dark, perfectly clear night, most of us could see a candle flame atop another mountain 30 miles away. We could feel the wing of a bee falling on our cheek. We could smell a single drop of perfume in a three-room apartment (Galanter, 1962). German scientist and philosopher Gustav Fechner (1801–1887) studied the edge of our awareness of these faint stimuli, which he called their absolute thresholds—the minimum stimulation necessary to detect a particular light, sound, pressure, taste, or odor 50 percent of the time. To test your absolute threshold for sounds, a hearing specialist would send 471 tones, at varying levels, into each of your ears and record whether you could hear each tone (Figure 16.6). The test results would show the point where, for any sound frequency, half the time you could detect the sound and half the time you could not. That 50-50 point would define your absolute threshold. absolute threshold the minimum stimulus energy needed to detect a particular stimulus 50 percent of the time. Figure 16.6 Absolute threshold Can I detect this sound? An absolute threshold is the intensity at which a person can detect a stimulus half the time. Hearing tests locate these thresholds for various frequencies. Detecting a weak stimulus, or signal (such as a hearing-test tone), depends not only on its strength but also on our psychological state—our experience, expectations, motivation, and alertness. Signal detection theory predicts when we will detect weak signals (measured as our ratio of “hits” to “false alarms”). Signal detection theorists seek to understand why people respond differently to the same stimuli (have you noticed that some teachers are more likely than others to detect students texting during 472 class?), and why the same person’s reactions vary as circumstances change —as when creaking sounds trigger fear for someone home alone after watching a scary movie. signal detection theory a theory predicting how and when we detect the presence of a faint stimulus (signal) amid background stimulation (noise). Assumes there is no single absolute threshold and that detection depends partly on a person’s experience, expectations, motivation, and alertness. Stimuli you cannot consciously detect 50 percent of the time are subliminal—below your absolute threshold (Figure 16.6). Can we be controlled by subliminal messages? For more on that question, see Thinking Critically About: Subliminal Sensation and Subliminal Persuasion. subliminal below one’s absolute threshold for conscious awareness. Difference Thresholds The difference threshold In this computer-generated copy of the Twenty- 473 third Psalm, each line of the typeface increases slightly. How many lines are required for you to experience a just noticeable difference? To function effectively, we need absolute thresholds low enough to allow us to detect important sights, sounds, textures, tastes, and smells. We also need to detect small differences among stimuli. A musician must detect minute discrepancies when tuning an instrument. Students in the hallway will detect the sound of their friends’ voices amid all the other voices. Even after I [DM] had spent two years in Scotland, all lambs’ baas sounded alike to my ears. But not to their mothers’, I observed. After shearing, each ewe would streak directly to the baa of her lamb amid the chorus of other distressed lambs. The difference threshold (or the just noticeable difference [jnd]) is the minimum stimulus difference a person can detect half the time. That detectable difference increases with the size of the stimulus. If we listen to our music at 40 decibels, we might barely detect an added 5 decibels (the jnd). But if we increase the volume to 110 decibels, we probably won’t then detect an additional 5-decibel change. difference threshold the minimum difference between two stimuli required for detection 50 percent of the time. We experience the difference threshold as a just noticeable 474 difference (or jnd). priming the activation, often unconsciously, of certain associations, thus predisposing one’s perception, memory, or response. In the late 1800s, Ernst Weber described this with a principle so simple and so widely applicable that we still refer to it as Weber’s law: For an average person to perceive a difference, two stimuli must differ by a constant minimum percentage (not a constant amount). The exact 475 percentage varies, depending on the stimulus. Two lights, for example, must differ in intensity by 8 percent. Two objects must differ in weight by 2 percent. And two tones must differ in frequency by only 0.3 percent (Teghtsoonian, 1971). Weber’s law the principle that, to be perceived as different, two stimuli must differ by a constant minimum percentage (rather than a constant amount). 476 Sensory Adaptation 16-6 What is the function of sensory adaptation? Sitting down in class, you notice a nearby student’s heavy perfume. You wonder how she endures it, but within minutes you no longer notice. Sensory adaptation has come to your rescue. When constantly exposed to an unchanging stimulus, we become less aware of it because our nerve cells fire less frequently. (To experience sensory adaptation, put a pen behind your ear. You will feel it—but only for a few moments.) sensory adaptation diminished sensitivity as a consequence of constant stimulation. Why, then, if we stare at an object without flinching, does it not vanish from sight? Because, unnoticed by us, our eyes are always moving. This continual flitting from one spot to another ensures that stimulation on the eyes’ receptors continually changes (Figure 16.7). Figure 16.7 The jumpy eye Our gaze jumps from one spot to another every third of a second or so, as eye-tracking equipment illustrated as a person looked at this 477 photograph of Edinburgh’s Princes Street Gardens (Henderson, 2007). The circles represent visual fixations, and the numbers indicate the time of fixation in milliseconds (300 milliseconds = 3/10ths of a second). What if we actually could stop our eyes from moving? Would sights seem to vanish, as odors do? To find out, psychologists have devised ingenious instruments that maintain a constant image on the eye’s inner surface. Imagine that we have fitted a volunteer, Mary, with such an instrument—a miniature projector mounted on a contact lens (Figure 16.8). When Mary’s eye moves, the image from the projector moves as well. So everywhere that Mary looks, the scene is sure to go. Figure 16.8 Sensory adaptation: Now you see it, now you don’t! (a) A projector mounted on a contact lens makes the projected image move with the eye. (b) Initially, the person sees the stabilized image —but soon, thanks to sensory adaptation, her eye becomes accustomed to the unchanging stimulus. Rather than the full image, she begins to see fragments fading and reappearing. If we project images through this instrument, what does Mary see? At first, she sees the complete image. But within a few seconds, as her sensory system begins to fatigue, things get weird. Bit by bit, the image vanishes, only to reappear and then disappear—often in fragments (Figure 16.8b). 478 “ We need above all to know about changes; no one wants or needs to be reminded 16 hours a day that his shoes are on.” Neuroscientist David Hubel (1979) Although sensory adaptation reduces our sensitivity, it offers an important benefit: freedom to focus on informative changes in our environment. The attention-grabbing power of changing stimulation helps explain why a smart phone’s notifications are so hard to ignore. New tweets, likes, snapchats, and breaking news stories pop up and capture our attention. If we’re performing other tasks, these intrusions can harm our performance (Stothart et al., 2015). Ditto for TV, which led one researcher to marvel at how TV cuts, zooms, and pans distract, even during interesting conversations: “I cannot for the life of me stop from periodically glancing over to the screen” (Tannenbaum, 2002). Sensory adaptation even influences how we perceive emotions. By creating a 50-50 morphed blend of an angry face and a scared face, researchers showed that our visual system adapts to a facial expression (as on the left or right of Figure 16.9) by becoming less responsive to it (Butler et al., 2008). The effect is created by our brain, not our retinas. We know this because the illusion also works when we view either side image with one eye, and the center image with the other eye. Figure 16.9 Emotion adaptation Gaze at the angry face on the left for 20 to 30 seconds, then look at 479 the center face (looks scared, yes?). Then gaze at the scared face on the right for 20 to 30 seconds, before returning to the center face (now looks angry, yes?). (From Butler et al., 2008.) The point to remember: Our sensory system is alert to novelty; bore it with repetition and it frees our attention for more important things. We will see this principle again and again: We perceive the world not exactly as it is, but as it is useful for us to perceive it. Check Your Understanding Ask Yourself Can you recall a recent time when, your attention focused on one thing, you were oblivious to something else (perhaps to pain, to someone’s approach, or to background music)? Test Yourself Explain how Heather Sellers’ experience of prosopagnosia illustrates the difference between sensation and perception. Explain three attentional principles that magicians may use to fool us. Using sound as your example, explain how these concepts differ: absolute threshold, subliminal stimulation, and difference threshold. Why is it that after wearing shoes for a while, you cease to notice them? Answers to the Test Yourself questions can be found in Appendix E at the end of the book. 480 Module 16 REVIEW 16-1 What are sensation and perception? What do we mean by bottom-up processing and top-down processing? Sensation is the process by which our sensory receptors and nervous system receive and represent stimulus energies from our environment. Perception is the process of organizing and interpreting this information, enabling recognition of meaningful events. Sensation and perception are actually parts of one continuous process. Bottom-up processing is sensory analysis that begins at the entry level, with information flowing from the sensory receptors to the brain. Top- down processing is information processing guided by high-level mental processes, as when we construct perceptions by filtering information through our experience and expectations. 16-2 How does selective attention direct our perceptions? We selectively attend to, and process, a very limited portion of incoming information, blocking out much and often shifting the spotlight of our attention from one thing to another. Focused intently on one task, we often display inattentional blindness (including change blindness) to other events and changes around us. 16-3 What three steps are basic to all our sensory systems? Our senses (1) receive sensory stimulation (often using specialized receptor cells), (2) transform that stimulation into neural impulses, and (3) deliver the neural information to the brain. Transduction is the process of converting one form of energy into another. Researchers in psychophysics study the relationships between stimuli’s physical characteristics and our psychological experience of them. 481 16-4 How do absolute thresholds and difference thresholds differ? Our absolute threshold for any stimulus is the minimum stimulation necessary for us to be consciously aware of it 50 percent of the time. Signal detection theory predicts how and when we will detect a faint stimulus amid background noise. Individual absolute thresholds vary, depending on the strength of the signal and also on our experience, expectations, motivation, and alertness. Our difference threshold (also called just noticeable difference, or jnd) is the minimum stimulus difference we can discern 50 percent of the time. Weber’s law states that two stimuli must differ by a constant percentage (not a constant amount) to be perceived as different. 16-5 How are we affected by subliminal stimuli? Priming shows that we can be affected by stimuli so weak that we don’t consciously notice them, and we can evaluate a stimulus even when we’re not consciously aware of it. While we can be primed by subliminal stimuli, however, research indicates that such stimuli cannot persuade us or change our behavior. 16-6 What is the function of sensory adaptation? Sensory adaptation (our diminished sensitivity to constant odors, sights, sounds, and touches) focuses our attention on informative changes in our environment. Multiple-Choice Questions 1. As she looks at a face, Jamie is able to recognize it as the face of her mother. Which of the following explains why she can recognize her mother’s face? a. Selective attention 482 b. Transduction c. Bottom-up processing d. Top-down processing e. Signal detection theory 2. Charles and his wife are at a loud baseball game, yet are able to have a conversation with each other in spite of all the noise around them. Which principle best explains this scenario? a. Bottom-up processing b. Weber’s law c. The cocktail party effect d. Top-down processing e. Sensory adaptation 3. As Jeff reads his psychology textbook he is able to convert the light waves into signals that his brain can interpret due to the concept of a. transduction. b. perception. c. priming. d. signal detection theory. e. threshold. 4. Natalia is washing her hands, and she adjusts the faucet handle until the water feels just slightly hotter than it did before. Natalia’s adjustment until she feels the change in temperature is an example of a. a subliminal stimulus. b. an absolute threshold. c. a difference threshold. d. signal detection. e. perceptual constancy. 5. Tyshane went swimming with friends who did not want to get into the pool because the water felt cold. Tyshane jumped in and after a few minutes declared, “It was cold when I first got in, but now it’s fine. Come on in!” Tyshane’s body became accustomed to the water 483 temperature due to a. priming. b. absolute threshold. c. difference threshold. d. selective attention. e. sensory adaptation. Practice FRQs 1. Explain how the following are involved when you listen to your favorite song: Bottom-up processing Top-down processing Answer 1 point: Bottom-up processing will take place in the ear and allow you to hear the individual notes and words of the song. Page 158 1 point: Top-down processing will take place in the brain and allow you to recognize the song as your favorite song. Page 158 2. Marisol is planning a ski trip for winter break. Explain how each of the following might play a role in her perception of the winter weather she will experience: Difference threshold Absolute threshold Selective attention (3 points) 484 Module 17 Influences on Perception LEARNING TARGETS 17-1 Analyze the ways in which our expectations, contexts, motivation, and emotions influence our perceptions. 17-2 Describe the claims of ESP, and discuss what most research psychologists have concluded after putting these claims to the test. 485 Perceptual Set 17-1 How do our expectations, contexts, motivation, and emotions influence our perceptions? To see is to believe. As we less fully appreciate, to believe is to see. Through experience, we come to expect certain results. Those expectations may give us a perceptual set, a set of mental tendencies and assumptions that affects, top-down, what we hear, taste, feel, and see. perceptual set a mental predisposition to perceive one thing and not another. Consider: Is the center image in Figure 17.1 an old or young woman? What we see in such a drawing can be influenced by first looking at either of the two unambiguous versions (Boring, 1930). Likewise, in the figure below Figure 17.1: Reading from left to right, our expectations cause us to perceive the middle script differently than when reading from top to bottom. Figure 17.1 Perceptual set Show a friend either the left or right image. Then show the center image and ask, “What do you see?” Whether your friend reports 486 seeing an old woman’s face or young woman’s profile may depend on which of the other two drawings was viewed first. In each of those images, the meaning is clear, and it will establish perceptual expectations. Everyday examples abound of perceptual set—of “mind over mind.” In 1972, a British newspaper published unretouched photographs of a “monster” in Scotland’s Loch Ness—“the most amazing pictures ever taken,” stated the paper. If this information creates in you the same expectations it did in most of the paper’s readers, you, too, will see the monster in a similar photo in Figure 17.2. But when a skeptical researcher approached the original photos with different expectations, he saw a curved tree limb—as had others the day that photo was shot (Campbell, 1986). With this different perceptual set, you may now notice that the object is floating motionless, with ripples outward in all directions—hardly what we would expect of a swimming monster. 487 Figure 17.2 Believing is seeing What do you perceive? Is this Nessie, the Loch Ness monster, or a log? To believe is also to hear. Consider the kindly airline pilot who, on a takeoff run, looked over at his sad co-pilot and said, “Cheer up.” Expecting to hear the usual “Gear up,” the co-pilot promptly raised the wheels—before they left the ground (Reason & Mycielska, 1982). The context of spoken words will determine whether you hear “the stuffy nose” or “the stuff he knows.” And depending on your perceptual set, the weather-forecasting “meteorologist” may become a muscular kidney specialist—your “meaty urologist.” Music lovers have recorded thousands of mishearings at kissthisguy.com, a website named for Jimi Hendrix’s oft- misheard phrase “kiss the sky.” Or consider this odd question: If you said one thing but heard yourself saying another, what would you think you said? To find out, a clever Swedish research team invited people to name a font color, such as saying “gray” when the word green was presented in a gray font (Lind et al., 2014). While the participants heard themselves speaking over a noise- cancelling headset, the experimenters occasionally substituted the participant’s own previously recorded voice, such as saying “green” instead of “gray.” Surprisingly, people usually missed the switch—and experienced the inserted word as self-produced. What they heard controlled their perception of what they had just said. Perceptual set at work. 488 Our expectations can also influence our taste perceptions. In one experiment, preschool children, by a 6-to-1 margin, thought french fries tasted better when served in a McDonald’s bag rather than a plain white bag (Robinson et al., 2007). Another experiment invited campus bar patrons at the Massachusetts Institute of Technology to sample free beer (Lee et al., 2006). When researchers added a few drops of vinegar to a brand-name beer and called it “MIT brew,” the tasters preferred it—unless they had been told they were drinking vinegar-laced beer. In that case, they expected, and usually experienced, a worse taste. There Are Two Errors in The The Title Of This Book Book by Robert M. Martin, 2011 Did you perceive what you expected in this title—and miss the errors? If you are still puzzled, see the explanation below.1 What determines our perceptual set? As Module 47 will explain, through experience we form concepts, or schemas, that organize and interpret unfamiliar information. Our preexisting schemas for monsters and tree trunks influence how we apply top-down processing to interpret ambiguous sensations. In everyday life, stereotypes about gender (another instance of perceptual set) can color perception. Without the obvious cues of pink or blue, people will struggle over whether to call the new baby “he” or “she.” But told an infant is “David,” people (especially children) have perceived “him” as bigger and stronger than when the same infant was called “Diana” (Stern & Karraker, 1989). Some differences, it seems, exist merely in the eyes of their beholders. Context, Motivation, and Emotion 489 Perceptual set influences how we interpret stimuli. But our immediate context, and the motivation and emotion we bring to a situation, also affect our interpretations. Context Social psychologist Lee Ross invited us to recall our own perceptions in different contexts: “Ever notice that when you’re driving you hate pedestrians, the way they saunter through the crosswalk, almost daring you to hit them, but when you’re walking you hate drivers?” (Jaffe, 2004). Some other examples of the power of context: When holding a gun, people become more likely to perceive another person as also gun-toting—a phenomenon that has led to the shooting of some unarmed people who were actually holding their phone or wallet (Witt & Brockmole, 2012). Imagine hearing a noise interrupted by the words “eel is on the wagon.” Likely, you would actually perceive the first word as wheel. Given “eel is on the orange,” you would more likely hear peel. In each case, the context creates an expectation that, top-down, influences our perception of a previously heard phrase (Grossberg, 1995). Cultural context helps inform our perceptions, so it’s not surprising that people from different cultures view things differently, as in Figure 17.3. How tall is the shorter player in Figure 17.4? And how is the woman in Figure 17.5 feeling? The context provided in Figure 17.6 will leave no doubt. 490 Figure 17.3 Culture and context effects What is above the woman’s head? In one classic study, most rural East Africans questioned said the woman was balancing a metal box or can on her head (a typical way to carry water at that time). They also perceived the family as sitting under a tree. Westerners, used to running water and box-like houses with corners, were more likely to perceive the family as being indoors, with the woman sitting under a window (Gregory & Gombrich, 1973). 491 Figure 17.4 Big and “little” The “little guy” shown here is actually a 6’9’’ former Hope College basketball center who would tower over most of us. But he seemed like a short player when matched in a semi-pro game against the world’s tallest basketball player at that time, 7’9’’ Sun Ming Ming from China. 492 Figure 17.5 What emotion is this? (See Figure 17.6.) Figure 17.6 Context makes clearer The Hope College volleyball team celebrates its national championship winning moment. Motivation Motives give us energy as we work toward a goal. Like context, they can bias our interpretations of neutral stimuli. Consider these findings: Desirable objects, such as a water bottle viewed by a thirsty person, 493 seem closer than they really are (Balcetis & Dunning, 2010). This perceptual bias energizes our going for it. A to-be-climbed hill can seem steeper when we are carrying a heavy backpack, and a walking destination further away when we are feeling tired (Burrow et al., 2016; Philbeck & Witt, 2015; Proffitt 2006a,b). Going on a diet can lighten our biological “backpack” (Taylor-Covill & Eves, 2016). When heavy people lose weight, hills and stairs no longer seem so steep. A softball appears bigger when you’re hitting well, as researchers observed after asking players to choose a circle the size of the ball they had just hit well or poorly. There’s also a reciprocal phenomenon: Seeing a target as bigger—as happens when athletes focus directly on a target—improves performance (Witt et al., 2012). Emotion Other clever experiments have demonstrated that emotions can shove our perceptions in one direction or another. Hearing sad music can predispose people to perceive a sad meaning in spoken homophonic words—mourning rather than morning, die rather than dye, pain rather than pane (Halberstadt et al., 1995). When angry, people more often perceive neutral objects as guns (Baumann & DeSteno, 2010). When mildly upset by subliminal exposure to a scowling face, people perceive a neutral face as less attractive and likeable (Anderson et al., 2012). Emotions and motives color our social perceptions, too. People more often perceive solitary confinement, sleep deprivation, and cold temperatures as “torture” when experiencing a small dose of such themselves (Nordgren et al., 2011). Spouses who feel loved and appreciated perceive less threat in stressful marital events—“He’s just having a bad day” (Murray et al., 2003). The moral of all these examples? Much of what we perceive comes not 494 just from what’s “out there,” but also from what’s behind our eyes and between our ears. Through top-down processing, our experiences, assumptions, expectations—and even our context, motivation, and emotions—can shape and color our views of reality. 495 ESP—Perception Without Sensation? 17-2 What are the claims of ESP, and what have most research psychologists concluded after putting these claims to the test? The river of perception is fed by sensation, cognition, and emotion. If perception is the product of these three sources, what can we say about extrasensory perception (ESP), which claims that perception can occur apart from sensory input? Are there indeed people—any people—who can read minds, see through walls, or foretell the future? Nearly half of Americans have agreed there are (AP, 2007; Moore, 2005). extrasensory perception (ESP) the controversial claim that perception can occur apart from sensory input; includes telepathy, clairvoyance, and precognition. If ESP is real, we would need to overturn the scientific understanding that we are creatures whose minds are tied to our physical brains and whose perceptual experiences of the world are built of sensations. The most testable and, for this discussion, most relevant ESP claims are telepathy: mind-to-mind communication. clairvoyance: perceiving remote events, such as a house on fire in another state. precognition: perceiving future events, such as an unexpected death in the next month. Closely linked is psychokinesis, or “mind moving matter,” such as levitating a table or influencing the roll of a die. (The claim, also called telekinesis, is illustrated by the wry request, “Will all those who believe in psychokinesis please raise my hand?”) Most research psychologists and scientists have been skeptical that paranormal phenomena exist. But in several reputable universities, 496 parapsychology researchers perform scientific experiments searching for possible ESP phenomena (Storm et al., 2010a,b; Turpin, 2005). Before seeing how they conduct their research, let’s consider some popular beliefs. parapsychology the study of paranormal phenomena, including ESP and psychokinesis. Premonitions or Pretensions? Can psychics see into the future? Although one might wish for a psychic stock forecaster, the tallied forecasts of “leading psychics” reveal meager accuracy. During the 1990s, the tabloid psychics were all wrong in predicting surprising events. (Madonna did not become a gospel singer, the Statue of Liberty did not lose both its arms in a terrorist blast, Queen Elizabeth did not abdicate her throne to enter a convent.) And the psychics have missed big-news events. Where were the psychics on 9/10 when we needed them? Why, despite a $50 million reward, could no psychics help locate Osama bin Laden after 9/11? And why, when the Chilean government consulted four psychics after a mine collapse trapped 33 miners in 2010, did those psychics sorrowfully decide “They’re all dead” (Kraul, 2010)? Imagine their surprise when all 33 miners were rescued 69 days later. After Amanda Berry went missing in Cleveland in 2003, her distraught and desperate mother turned to a famed TV psychic detective for answers. “She’s not alive, honey,” the psychic told the devastated mom, who died without living to see her daughter rescued in 2013 (Radford, 2013). According to one analysis, this result brought that psychic’s record on 116 missing person and death cases to 83 unknown outcomes, 33 incorrect, and zero mostly correct. To researcher Ryan Shaffer (2013), that’s the record of a “psychic defective.” The psychic visions offered to police departments have been no more accurate than guesses made by others (Nickell, 1994, 2005; Radford, 2010; 497 Reiser, 1982). But their sheer volume does increase the odds of an occasional correct guess, which psychics can then report to the media. Such visions can sound amazingly correct when later retrofitted to match events. Nostradamus, a sixteenth-century French psychic, explained in an unguarded moment that his ambiguous prophecies “could not possibly be understood till they were interpreted after the event and by it.” Are everyday people’s “visions” any more accurate than the psychics’ predictions? Do our dreams foretell the future, or do they only seem to do so when we recall or reconstruct them in light of what has already happened? Are our remembered visions merely revisions? After famed aviator Charles Lindbergh’s baby son was kidnapped and murdered in 1932, but before the body was discovered, two Harvard psychologists invited people to report their dreams about the child (Murray & Wheeler, 1937). How many visionaries replied? 1300. How many accurately envisioned the child dead? Five percent. How many also correctly anticipated the body’s location—buried among trees? Only four. Although this number was surely no better than chance, to those four dreamers, the accuracy of their apparent precognitions must have seemed uncanny. “ To be sure of hitting the target, shoot first and call whatever you hit the target.” Writer-artist Ashleigh Brilliant Given the countless events in the world each day, and given enough 498 days, some stunning coincidences are bound to occur. By one careful estimate, chance alone would predict that more than a thousand times per day, someone on Earth will think of another person and then, within the next five minutes, learn of that person’s death (Charpak & Broch, 2004). Thus, when explaining an astonishing event, we should “give chance a chance” (Lilienfeld, 2009). With enough time and people, the improbable becomes inevitable. “ A person who talks a lot is sometimes right.” Spanish proverb Putting ESP to Experimental Test When faced with claims of mind reading or out-of-body travel or communication with the dead, how can we separate fiction from strange- but-true fact? At the heart of science is a simple answer: Test them to see if they work. If they do, so much the better for the ideas. If they don’t, so much the better for our skepticism. Both believers and skeptics agree that what parapsychology needs is a reproducible phenomenon and a theory to explain it. Parapsychologist Rhea White (1998) spoke for many in saying that “the image of parapsychology that comes to my mind, based on nearly 44 years in the field, is that of a small airplane [that] has been perpetually taxiing down the runway of the Empirical Science Airport since 1882... its movement punctuated occasionally by lifting a few feet off the ground only to bump back down on the tarmac once again. It has never taken off for any sustained flight.” How might we test ESP claims in a controlled, reproducible experiment? An experiment differs from a staged demonstration. In the laboratory, the experimenter controls what the “psychic” sees and hears. On stage, the psychic controls what the audience sees and hears. 499 Testing psychic powers in the British population Psychologists created a “mind machine” to see if people could influence or predict a coin toss (Wiseman & Greening, 2002). Using a touch-sensitive screen, visitors to British festivals were given four attempts to call heads or tails, playing against a computer that kept score. By the time the experiment ended, nearly 28,000 people had predicted 110,959 tosses—with 49.8 percent correct. Daryl Bem, a respected social psychologist, once quipped that “a psychic is an actor playing the role of a psychic” (1984). Yet this one-time skeptic reignited hopes for replicable evidence of ESP with nine experiments that seemed to show people anticipating future events (Bem, 2011). In one, when an erotic scene was about to appear on a screen in one of two randomly selected positions, Cornell University participants guessed the right placement 53.1 percent of the time (beating 50 percent by a small but statistically significant margin). Bem wondered if his “anomalous” findings reflected an evolutionary advantage to those who can precognitively anticipate future events. Despite the paper having survived critical reviews by a top-tier journal, critics scoffed. Some found the methods “badly flawed” (Alcock, 2011) or the statistical analyses “biased” (Wagenmakers et al., 2011). Others predicted the results could not be replicated by “independent and skeptical researchers” (Helfand, 2011). And still others had more sweeping objections: “If any of his claims were true,” wrote one cognitive scientist, “then all of the bases underlying contemporary science would be toppled, and we would have to rethink everything about the nature of the universe” (Hofstadter, 2011). Anticipating such skepticism, Bem has made his research materials 500 available to anyone who wishes to replicate his studies. Multiple attempts have met with minimal success and continuing controversy (Bem et al., 2014; Galak et al., 2012; Ritchie et al., 2012; Wagenmakers, 2014). Regardless, science is doing its work: It has been open to a finding that challenges its own assumptions. Through follow-up research, it has assessed the validity of that finding. And that is how science sifts crazy-sounding ideas, leaving most on the historical waste heap while occasionally surprising us. “ At the heart of science is an essential tension between two seemingly contradictory attitudes—an openness to new ideas, no matter how bizarre or counterintuitive they may be, and the most ruthless skeptical scrutiny of all ideas, old and new. ” Carl Sagan (1987) For nineteen years, one skeptic, magician James Randi, offered $1 million “to anyone who proves a genuine psychic power under proper observing conditions” (Randi, 1999; Thompson, 2010). French, Australian, and Indian groups have made similar offers (CFI, 2003). Large as these sums are, the scientific seal of approval would be worth far more. To refute those who say there is no ESP, one need only produce a single person who can demonstrate a single, reproducible ESP event. (To refute those who say pigs can’t talk would take but one talking pig.) So far, no such person has emerged. Check Your Understanding Ask Yourself Can you recall a time when your expectations have predisposed how you perceived a person or group—perhaps at school, work, or in your community? Test Yourself 501 What type of evidence shows that, indeed, “there is more to perception than meets the senses”? Does perceptual set involve bottom-up or top-down processing? Why? If an ESP event occurred under controlled conditions, what would be the next best step to confirm that ESP really exists? Answers to the Test Yourself questions can be found in Appendix E at the end of the book. 502 Module 17 REVIEW 17-1 How do our expectations, contexts, motivation, and emotions influence our perceptions? Perceptual set is a mental predisposition that functions as a lens through which we perceive the world. Our learned concepts (schemas) prime us to organize and interpret ambiguous stimuli in certain ways. Our motivation, as well as our physical and emotional context, can create expectations and color our interpretation of events and behaviors. 17-2 What are the claims of ESP, and what have most research psychologists concluded after putting these claims to the test? Parapsychology is the study of paranormal phenomena, including extrasensory perception (ESP) and psychokinesis. The three most testable forms of ESP are telepathy (mind-to-mind communication), clairvoyance (perceiving remote events), and precognition (perceiving future events). Skeptics argue that (1) to believe in ESP, you must believe the brain is capable of perceiving without sensory input, and (2) researchers have been unable to replicate ESP phenomena under controlled conditions. Multiple-Choice Questions 1. Tonya’s psychology teacher played some backwards music to the class, and they were not able to make out any words or phrases in the selection. When the teacher told them to listen for the words “The rat ate the cat” when she played it again, most of the class now heard the words. Which principle explains this experience? a. A context effect 503 b. Perceptual set c. Extrasensory perception d. Selective attention e. Weber’s law 2. On a warm summer day, Kimberly tells her brother to put on a suit. Kimberly’s brother knows to put on a swimsuit instead of a business suit because of a. context. b. priming. c. sensory adaptation. d. bottom-up processing. e. clairvoyance. 3. A famous psychic is a YouTube sensation because of his ability to read the minds of people in the audience at his shows. Which type of psychic power would he claim? a. Precognition b. Psychokinesis c. Clairvoyance d. Telepathy e. Bottom-up processing Practice FRQs 1. Martha is convinced she has extrasensory perception. Explain the specific abilities Martha would display if she had each of the following forms of ESP: Telepathy Clairvoyance Precognition Then briefly explain why you should doubt her claims. Answer 504 1 point: Telepathy: Martha would be able to use mind-to-mind communication. For example, she may know what someone in another room is thinking. Page 172 1 point: Clairvoyance: Martha would be able to perceive things happening at a distance. For example, she may know that her cousin who lives in another state has just burned her hand on the oven. Page 172 1 point: Precognition: Martha would be able to see future events happen. For example, she may know that a pop quiz will take place next week. Page 172 1 point: There has never been a conclusive scientific demonstration of extrasensory ability. Page 173 2. David has just witnessed a robbery at his bank. Explain how the following can affect his perceptions of the robbery: Context effects Emotions Motivations (3 points) 505 Module 18 Vision: Sensory and Perceptual Processing LEARNING TARGETS 18-1 Discuss the characteristics of the energy that we see as visible light, and describe the structures in the eye that help focus that energy. 18-2 Describe how the rods and cones process information, and explain the path information travels from the eye to the brain. 18-3 Discuss how we perceive color in the world around us. 18-4 Describe the location and function of feature detectors. 18-5 Explain how the brain uses parallel processing to construct visual perceptions. 506 Light Energy and Eye Structures 18-1 What are the characteristics of the energy that we see as visible light? What structures in the eye help focus that energy? Our eyes receive light energy and transduce (transform) it into neural messages. From this neural input, our brain—in one of life’s greatest wonders—then creates what we consciously see. How does such a taken- for-granted yet extraordinary thing happen? The Stimulus Input: Light Energy When you look at a bright red tulip, the stimuli striking your eyes are not particles of the color red, but pulses of electromagnetic energy that your visual system perceives as red. What we see as visible light is but a thin slice of the wide spectrum of electromagnetic energy shown in Figure 18.1. On the spectrum’s one end are the short gamma waves, no longer than the diameter of an atom. On the other end are the mile-long waves of radio transmission. In between is the narrow band visible to us. Other portions are visible to other animals. Bees, for instance, cannot see what we perceive as red but can see ultraviolet light. 507 Figure 18.1 The wavelengths we see What we see as light is only a tiny slice of a wide spectrum of electromagnetic energy, which ranges from gamma rays as short as the diameter of an atom to radio waves over a mile long. The wavelengths visible to the human eye (shown enlarged) extend from the shorter waves of blue-violet light to the longer waves of red light. Light travels in waves, and the shape of those waves influences what we see. Light’s wavelength is the distance from one wave peak to the next (Figure 18.2a). Wavelength determines hue, the color we experience, such as the tulip’s red petals or green leaves. A light wave’s amplitude, or height, determines its intensity—the amount of energy the wave contains. Intensity influences brightness (Figure 18.2b). 508 wavelength the distance from the peak of one light or sound wave to the peak of the next. Electromagnetic wavelengths vary from the short blips of gamma rays to the long pulses of radio transmission. hue the dimension of color that is determined by the wavelength of light; what we know as the color names blue, green, and so forth. intensity the amount of energy in a light wave or sound wave, which influences what we perceive as brightness or loudness. Intensity is determined by the wave’s amplitude (height). Figure 18.2 The physical properties of waves (a) Waves vary in wavelength (the distance between successive peaks). Frequency, the number of complete wavelengths that can pass a point in a given time, depends on the wavelength. The shorter the wavelength, the higher the frequency. Wavelength determines the perceived color of light. (b) Waves also vary in amplitude (the height from peak to trough). Wave amplitude influences the perceived brightness of colors. 509 To understand how we transform physical energy into color and meaning, we need to know more about vision’s window—the eye. The Eye Light enters the eye through the cornea, which bends light to help provide focus (Figure 18.3). The light then passes through the pupil, a small adjustable opening. Surrounding the pupil and controlling its size is the iris, a colored muscle that dilates or constricts in response to light intensity. Each iris is so distinctive that an iris-scanning machine can confirm your identity. cornea the eye’s clear, protective outer layer, covering the pupil and iris. pupil the adjustable opening in the center of the eye through which light enters. iris a ring of muscle tissue that forms the colored portion of the eye around the pupil and controls the size of the pupil opening. Figure 18.3 The eye Light rays reflected from a candle pass through the cornea, pupil, and lens. The curvature and thickness of the lens change to bring nearby 510 or distant objects into focus on the retina. Rays from the top of the candle strike the bottom of the retina, and those from the left side of the candle strike the right side of the retina. The candle’s image on the retina thus appears upside down and reversed. The iris responds to your cognitive and emotional states. Imagine a sunny sky and your iris will constrict, making your pupil smaller; imagine a dark room and it will dilate (Laeng & Sulutvedt, 2014). The iris also constricts when you are about to answer No to a question, or when you feel disgust (de Gee et al., 2014; Goldinger & Papesh, 2012). And when you’re feeling amorous, your telltale dilated pupils and dark eyes subtly signal your interest. After passing through your pupil, light hits the transparent lens in your eye. The lens then focuses the light rays into an image on your retina, a multilayered tissue on the eyeball’s sensitive inner surface. To focus the rays, the lens changes its curvature and thickness in a process called accommodation. If the lens focuses the image on a point in front of the retina, you see near objects clearly but not distant objects. This nearsightedness—myopia—can be remedied with glasses, contact lenses, or surgery. lens the transparent structure behind the pupil that changes shape to help focus images on the retina. retina the light-sensitive inner surface of the eye, containing the receptor rods and cones plus layers of neurons that begin the processing of visual information. accommodation the process by which the eye’s lens changes shape to focus near or far objects on the retina. 511 For centuries, scientists knew that an image of a candle passing through a small opening will cast an inverted mirror image on a dark wall behind. If the image passing through the pupil casts this sort of upside- down image on the retina, as in Figure 18.3, how can we see the world right side up? The ever-curious Leonardo da Vinci had an idea: Perhaps the eye’s watery fluids bend the light rays, reinverting the image to an upright position as it reaches the retina. Unfortunately for da Vinci, that idea was disproved in 1604, when the astronomer and optics expert Johannes Kepler showed that the retina does receive upside-down images of the world (Crombie, 1964). So how could we understand such a world? “I leave it,” said the befuddled Kepler, “to natural philosophers.” Today’s answer: The retina doesn’t “see” a whole image. Rather, its millions of receptor cells convert particles of light energy into neural impulses and forward those to the brain, which reassembles them into what we perceive as an upright-seeming image. And along the way, visual information processing percolates through progressively more abstract levels. The process is rather like taking a house apart and, with countless specialized workers, rebuilding it elsewhere. That all of this happens continuously and effortlessly is better than cool: It is awe-inspiring. Moreover, it happens with astonishing speed. Consider: As a baseball pitcher’s fastball approaches home plate, the light signals work their way from the batter’s retina to the visual cortex, which then informs the motor cortex, which then sends out orders to contract the muscles—all within the 4/10ths of a second that the ball is in flight. 512 Information Processing in the Eye and Brain Flip It Video: Rods and Cones in the Retina 18-2 How do the rods and cones process information, and what is the path information travels from the eye to the brain? AP® EXAM TIP There’s a lot of vocabulary here. Make sure you understand the name and the function of each part of the eye. To learn how all the parts fit together, it may help to make rough sketches (you don’t need to be an artist to try this!) and then compare your sketches with Figures 18.3 and 18.4. You’ll be better off making several quick, rough sketches than one time-consuming, nicely drawn one. The Eye-to-Brain Pathway Imagine that you could follow a single light-energy particle after it entered your eye. First, you would thread your way through the retina’s sparse outer layer of cells. Then, reaching the back of your eye, you would encounter the retina’s nearly 130 million buried receptor cells, the rods and cones (Figure 18.4). There, you would see the light energy trigger chemical changes. That chemical reaction would spark neural signals in nearby bipolar cells. You could then watch the bipolar cells activate neighboring ganglion cells, whose axons twine together like the strands of a rope to form the optic nerve. After a momentary stopover at the thalamus, the information would fly on to the final destination, your visual cortex, in the occipital lobe at the back of your brain. rods retinal receptors that detect black, white, and gray, and are sensitive to movement; necessary for peripheral and twilight vision, when cones don’t 513 respond. cones retinal receptors that are concentrated near the center of the retina and that function in daylight or in well-lit conditions. Cones detect fine detail and give rise to color sensations. optic nerve the nerve that carries neural impulses from the eye to the brain. Figure 18.4 The retina’s reaction to light The optic nerve is an information highway from the eye to the brain. This nerve can send nearly 1 million messages at once through its nearly 1 million ganglion fibers. (The auditory nerve, which enables hearing, carries much less information through its mere 30,000 fibers.) We pay a small price for this high-speed connection, however. Your eye has a blind spot, an area with no receptor cells, where the optic nerve leaves the eye (Figure 18.5). Close one eye and you won’t see a black hole, however. Without seeking your approval, your brain fills in the hole. 514 blind spot the point at which the optic nerve leaves the eye, creating a “blind” spot because no receptor cells are located there. Figure 18.5 The blind spot There are no receptor cells where the optic nerve leaves the eye. This creates a blind spot in your vision. To demonstrate, close your left eye, look at the spot, and move your face away until one of the cars disappears. (Which one do you predict it will be?) Repeat with your right eye closed—and note that now the other car disappears. Can you explain why?1 Rods and cones are our eyes’ light-sensitive photoreceptors. They differ in where they’re found and in what they do (Table 18.1). Cones cluster in and around the fovea, the retina’s area of central focus (Figure 18.3). Many cones have their own hotline to the brain: One cone transmits its message to a single bipolar cell, which relays the message to the visual cortex (where a large area receives input from the fovea). These direct connections preserve the cones’ precise information, making them better able to detect fine detail. Although cones can detect white, they also enable you to perceive color (Sabesan et al., 2016). But in dim light, they become unresponsive, so you see no colors. TABLE 18.1 Receptors in the Human Eye: Rod-Shaped Rods and Cone-Shaped Cones Cones Rods Number 6 million 120 million Location in retina Center Periphery Sensitivity in dim light Low High 515 Color sensitivity High Low Detail sensitivity High Low fovea the central focal point in the retina, around which the eye’s cones cluster. Unlike cones, which cluster in the center of your retina, rods are located around the retina’s outer regions. Rods remain sensitive in dim light, and they enable black-and-white vision. Rods have no hotline to the brain. If cones are soloists, rods perform as a chorus. Several rods pool their faint energy output and funnel it onto a single bipolar cell, which sends the combined message to your brain. Cones and rods each provide a special sensitivity—cones to detail and color, and rods to faint light and peripheral motion. Stop for a minute and experience this rod-cone difference. Pick a word in this sentence and stare directly at it, focusing its image on the cones in your fovea. Notice that words a few inches off to the side appear blurred? They lack detail because their image is striking your retina’s outer regions, where rods predominate. Thus, when driving or biking, rods help you detect a car in your peripheral vision well before you perceive its details. And in Figure 18.6, which has 12 black dots, you can see barely two at a time, with your brain filling in the less distinct peripheral input (Kitaoka, 2016, adapting Ninio & Stevens, 2000). 516 Figure 18.6 How many dots do you see at once? Look at or near any of the twelve black dots and you can see them, but not in your peripheral vision. When you enter a darkened theater or turn off the light at night, your pupils dilate to allow more light to reach your retina. Your eyes adapt, but fully adapting typically takes 20 minutes or more. This period of dark adaptation matches the average natural twilight transition between the Sun’s setting and darkness. How wonderfully made we are. At the entry level, the retina’s neural layers don’t just pass along electrical impulses; they also help to encode and analyze sensory information. (The third neural layer in a frog’s eye, for example, contains those “bug detector” cells that fire only in response to moving fly-like stimuli.) In human eyes, any given retinal area relays its information to a corresponding location in your visual cortex, in the occipital lobe at the back of your brain (Figure 18.7). 517 Figure 18.7 Pathway from the eyes to the visual cortex Ganglion axons forming the optic nerve run to the thalamus, where they synapse with neurons that run to the visual cortex. The same sensitivity that enables retinal cells to fire messages can lead them to misfire, as you can demonstrate. Turn your eyes to the left, close them, and then gently rub the right side of your right eyelid with your fingertip. Note the patch of light to the left, moving as your finger moves. Why do you see light? Why at the left? This happens because your retinal cells are so responsive that even pressure triggers them. But your brain interprets their firing as light. Moreover, it interprets the light as coming from the left—the normal direction of light that activates the right side of the retina. Check Your Understanding Ask Yourself Consider your activities in the last hour. Which of them relied on your rods? Which relied on your cones? How would these activities be different—or impossible—without these cells’ different abilities? Test Yourself 518 Some nocturnal animals, such as toads, mice, rats, and bats, have impressive night vision thanks to having many more ______________ (rods/cones) than ______________ (rods/cones) in their retinas. These creatures probably have very poor ______________ (color/black-and-white) vision. Cats are able to open their ______________ much wider than we can, which allows more light into their eyes so they can see better at night. Answers to the Test Yourself questions can be found in Appendix E at the end of the book. Color Processing 18-3 How do we perceive color in the world around us? We talk as though objects possess color: “A tomato is red.” Recall the old question, “If a tree falls in the forest and no one hears it, does it make a sound?” We can ask the same of color: If no one sees the tomato, is it red? The answer is No. First, the tomato is everything but red, because it rejects (reflects) the long wavelengths of red. Second, the tomato’s color is our mental construction. As Sir Isaac Newton (1704) noted, “The [light] rays are not colored.” Like all aspects of vision, our perception of color resides not in the object itself but in the theater of our brain, as illustrated by our dreaming in color. “ Only mind has sight and hearing; all things else are deaf and blind.” Epicharmus, Fragments, 550 B.C.E. One of vision’s most basic and intriguing mysteries is how we see the 519 world in color. How, from the light energy striking the retina, does our brain construct our experience of such a multitude of colors? Modern detective work on the mystery of color vision began in the nineteenth century, when Hermann von Helmholtz built on the insights of an English physicist, Thomas Young. They knew that any color can be created by combining the light waves of three primary colors—red, green, and blue. So Young and von Helmholtz formed a hypothesis: The eye must therefore have three corresponding types of color receptors. Years later, researchers confirmed the Young-Helmholtz trichromatic (three-color) theory. By measuring the response of various cones to different color stimuli, they confirmed that the retina does indeed have three types of color receptors, each especially sensitive to the wavelengths of red, green, or blue. When light stimulates combinations of these cones, we see other colors. For example, the retina has no separate receptors especially sensitive to yellow. But when red and green wavelengths stimulate both red-sensitive and green-sensitive cones, we see yellow. Young-Helmholtz trichromatic (three-color) theory the theory that the retina contains three different types of color receptors—one most sensitive to red, one to green, one to blue—which, when stimulated in combination, can produce the perception of any color. By one estimate, we can see differences among more than 1 million color variations (Neitz et al., 2001). At least most of us can. About 1 person in 50 is “colorblind.” That person is usually male, because the defect is genetically sex linked. Most people with color-deficient vision are not actually blind to all colors. They simply lack functioning red- or green- sensitive cones, or sometimes both. Their vision—perhaps unknown to them, because their lifelong vision seems normal—is monochromatic (one- color) or dichromatic (two-color) instead of trichromatic, making it impossible to distinguish the red and green in Figure 18.8 (Boynton, 1979). 520 Dogs, too, lack receptors for the wavelengths of red, giving them only limited, dichromatic color vision (Neitz et al., 1989). Figure 18.8 Flummoxed fans The photo on the left shows how people with red-green deficiency perceived a 2015 Buffalo Bills versus New York Jets football game. “For the 8 percent of Americans like me that are red-green colorblind, this game is a nightmare to watch,” tweeted one fan. “Everyone looks like they’re on the same team,” said another. The photo on the right shows how the game looked for those with normal color vision. But why do people blind to red and green often still see yellow? And why does yellow appear to be a pure color and not a mixture of red and green, the way purple is of red and blue? As physiologist Ewald Hering—a contemporary of von Helmholtz—noted, trichromatic theory leaves some parts of the color vision mystery unsolved. Hering found a clue in afterimages. Stare at a green square for a while and then look at a white sheet of paper, and you will see red, green’s opponent color. Stare at a yellow square, and its opponent color, blue, will appear on the white paper. (To experience this, try the flag demonstration in Figure 18.9.) Hering formed another hypothesis: Color vision must involve two additional color processes, one responsible for red-versus- green perception, and one for blue-versus-yellow perception. 521 Figure 18.9 Negative afterimage Stare at the center of the flag for a minute and then shift your eyes to the dot in the white space beside it. What do you see? (After tiring your neural response to black, green, and yellow, you should see their opponent colors.) Stare at a white wall and note how the size of the flag grows with the projection distance. Indeed, a century later, researchers also confirmed Hering’s hypothesis, now called the opponent-process theory. This concept is tricky, but here is the gist: Color vision depends on three sets of opposing retinal processes—red-green, blue-yellow, and white-black. As impulses travel to the visual cortex, some neurons in both the retina and the thalamus are turned “on” by red but turned “off” by green. Others are turned on by green but off by red (DeValois & DeValois, 1975). Like red and green marbles sent down a narrow tube, “red” and “green” messages cannot both travel at once. Red and green are thus opponents, so we see either red or green, not a reddish-green mixture. But red and blue travel in separate channels, so we can see a reddish-blue magenta. opponent-process theory the theory that opposing retinal processes (red-green, blue-yellow, white-black) enable color vision. For example, some cells are stimulated by green and inhibited by red; others are stimulated by red and inhibited by green. So how does opponent-process theory help us understand negative afterimages, as in the flag demonstration? Here’s the answer (for the green 522 changing to red): First, you stared at green bars, which tired your green response. Then you stared at a white area. White contains all colors, including red. Because you had tired your green response, only the red part of the green-red pairing fired normally. The present solution to the mystery of color vision is therefore roughly this: Color processing occurs in two stages. 1. The retina’s red, green, and blue cones respond in varying degrees to different color stimuli, as the Young-Helmholtz trichromatic theory suggested. 2. The cones’ responses are then processed by opponent-process cells, as Hering’s opponent-process theory proposed. Check Your Understanding Ask Yourself Does it surprise you to learn that colors don’t “live” in the objects we perceive—that in fact, these objects are everything but the color we experience? If someone had asked you “Is grass green?” before you read this section, how would you have responded? Test Yourself What are two key theories of color vision? Are they contradictory or complementary? Explain. Answers to the Test Yourself questions can be found in Appendix E at the end of the book. Feature Detection Flip It Video: Feature Detectors 18-4 Where are feature detectors located, and what do they do? Scientists once likened the brain to a movie screen on which the eye projected images. Then along came David Hubel and Torsten Wiesel 523 (1979), who showed that our visual processing deconstructs visual images and then reassembles them. Hubel and Wiesel received a Nobel Prize for their work on feature detectors, nerve cells in the occipital lobe’s visual cortex that respond to a scene’s edges, lines, angles, and movements. feature detectors nerve cells in the brain’s visual cortex that respond to specific features of the stimulus, such as shape, angle, or movement. Well-developed supercells In this 2011 World Cup match, USA’s Abby Wambach instantly processed visual information about the positions and movements of Brazil’s defenders and goalkeeper and somehow managed to get the ball around them all and into the net. Using microelectrodes, they had discovered that some neurons fired actively when cats were shown lines at one angle, while other neurons responded to lines at a different angle. They surmised that these specialized neurons, now known as feature detectors, receive information from individual ganglion cells in the retina. Feature detectors pass this specific information to other cortical areas, where teams of cells (supercell clusters) respond to more complex patterns. For biologically important objects and events, monkey brains (and surely ours as well) have a “vast visual encyclopedia” distributed as specialized cells (Perrett et al., 1990, 1992, 1994). These cells respond to one type of stimulus, such as a specific gaze, head angle, posture, or body 524 movement. Other supercell clusters integrate this information and fire only when the cues collectively indicate the direction of someone’s attention and approach. This instant analysis, which aided our ancestors’ survival, also helps a soccer player anticipate where to strike the ball, and a driver anticipate a pedestrian’s next movement. As we noted in Module 12, one temporal lobe area by your right ear (Figure 18.10) enables you to perceive faces and, thanks to a specialized neural network, to recognize them from varied viewpoints (Connor, 2010). If stimulated in this fusiform face area, you might spontaneously see faces —as did the participant who reported to an experimenter that “You just turned into someone else. Your face metamorphosed” (Koch, 2015). If this face recognition region were damaged, you might recognize other forms and objects, but not familiar faces. Figure 18.10 Face recognition processing In social animals such as humans, a large right temporal lobe area (shown here in a right-facing brain) is dedicated to the crucial task of face recognition. When researchers temporarily disrupt the brain’s face-processing areas with magnetic pulses, people are unable to recognize faces. But they can still recognize other objects, such as houses, because the brain’s face perception occurs separately from its object perception (McKone et al., 2007; Pitcher et al., 2007). Thus, functional MRI (fMRI) scans have 525 shown different brain areas activating when people viewed varied objects (Downing et al., 2001). Brain activity is so specific that, with the help of brain scans, researchers can tell whether people are “looking at a shoe, a chair, or a face, based on the pattern of their brain activity,” (Haxby, 2001). Parallel Processing 18-5 How does the brain use parallel processing to construct visual perceptions? Our brain achieves these and other remarkable feats by parallel processing: doing many things at once. To analyze a visual scene, the brain divides it into subdimensions—motion, form, depth, color—and works on each aspect simultaneously (Figure 18.11). We then construct our perceptions by integrating (binding) the separate but parallel work of these different visual teams (Livingstone & Hubel, 1988). parallel processing processing many aspects of a problem simultaneously; the brain’s natural mode of information processing for many functions, including vision. Figure 18.11 Parallel processing Studies of patients with brain damage suggest that the brain delegates the work of processing motion, form, depth, and color to different 526 areas. After taking a scene apart, the brain integrates these subdimensions into the perceived image. How does the brain do this? The answer to this binding problem (how does the brain bind multiple sensory inputs into a single perception?) is the Holy Grail of vision research. To recognize a face, your brain integrates information projected by your retinas to several visual cortex areas and compares it with stored information, thus enabling your fusiform face area to recognize the face: Grandmother! Scientists have debated whether this stored information is contained in a single cell or, more likely, distributed over a vast network of cells. Some supercells—actually nicknamed grandmother cells—do appear to respond very selectively to 1 or 2 faces in 100 (Bowers, 2009; Quiroga et al., 2013). The whole face recognition process requires tremendous brain power: 30 percent of the cortex (10 times the brain area devoted to hearing). Destroy or disable the neural workstation for a visual subtask, and something peculiar results, as happened to “Mrs. M.” (Hoffman, 1998). After a stroke damaged areas near the rear of both sides of her brain, she could not perceive motion. People in a room seemed “suddenly here or there but I [had] not seen them moving.” Pouring tea into a cup was a challenge because the fluid appeared frozen—she could not perceive it rising in the cup. After stroke or surgery has damaged the brain’s visual cortex, others have experienced blindsight (a phenomenon we met in Module 13). Shown a series of sticks, they report seeing nothing. Yet when asked to guess whether the sticks are vertical or horizontal, their visual intuition typically offers the correct response. When told, “You got them all right,” they are astounded. There is, it seems, a second “mind”—a parallel processing system—operating unseen. These separate visual systems for perceiving and for acting illustrate once again the astonishing dual processing of our two-track mind. *** 527 Think about the wonders of visual processing. As you read these words, the letters reflect light rays onto your retina, which triggers a process that sends formless nerve impulses to several areas of your brain, which integrate the information and decode its meaning. The amazing result: We have transferred information across time and space, from our minds to your mind (Figure 18.12). That all of this happens instantly, effortlessly, and continuously is indeed awesome. As Roger Sperry (1985) observed, the “insights of science give added, not lessened, reasons for awe, respect, and reverence.” Figure 18.12 A simplified summary of visual information processing “ I am fearfully and wonderfully made.” King David, Psalm 139:14 Check Your Understanding Ask Yourself Do you know anyone with a visual disability, or perhaps you have one yourself? Do you now have a better sense of how the visual process could be disrupted? Test Yourself What is the rapid sequence of events that occurs when you see and recognize a friend? 528 Answers to the Test Yourself questions can be found in Appendix E at the end of the book. 529 Module 18 REVIEW 18-1 What are the characteristics of the energy that we see as visible light? What structures in the eye help focus that energy? The hue we perceive in light depends on its wavelength, and its brightness depends on its intensity. After entering the eye through the cornea, passing through the pupil and iris, and being focused by the lens, light energy particles (from a thin slice of the broad spectrum of electromagnetic energy) strike the eye’s inner surface, the retina. 18-2 How do the rods and cones process information, and what is the path information travels from the eye to the brain? The retina’s light-sensitive rods and color-sensitive cones convert light energy into neural impulses. Cones are found in and around the fovea. Many cones have a direct hotline to the brain, transmitting their message to a single bipolar cell that relays it to the visual cortex. Rods are found in the retina’s outer regions. Several rods together transmit their energy to a single bipolar cell. Cones and rods each provide a special sensitivity—cones to detail and color, rods to faint light and peripheral motion. After processing by bipolar and ganglion cells in the eyes’ retina, neural impulses travel through the optic nerve, to the thalamus, and on to the visual cortex. 18-3 How do we perceive color in the world around us? The Young-Helmholtz trichromatic (three-color) theory proposed that the retina contains three types of color receptors. Contemporary research 530 has found three types of cones, each most sensitive to the wavelengths of one of the three primary colors of light (red, green, or blue). Hering’s opponent-process theory proposed three additional color processes (red-versus-green, blue-versus-yellow, white-versus-black). Contemporary research has confirmed that, en route to the brain, neurons in the retina and the thalamus code the color-related information from the cones into pairs of opponent colors. These two theories, and the research supporting them, show that color processing occurs in two stages. 18-4 Where are feature detectors located, and what do they do? Feature detectors, specialized neurons in the occipital lobe’s visual cortex, respond to specific aspects of the visual stimulus. They receive information from individual ganglion cells in the retina and pass it to other cortical areas, where supercell clusters respond to more complex patterns. 18-5 How does the brain use parallel processing to construct visual perceptions? Through parallel processing, the brain handles many aspects of vision (color, movement, form, and depth) simultaneously. Other neural teams integrate the results, comparing them with stored information and enabling perceptions. Multiple-Choice Questions 1. Which perceptual process explains why you can see varied aspects of your favorite singer’s face and instantly recognize him or her? a. Selective attention b. Accommodation c. Psychokinesis d. Blindsight 531 e. Parallel processing 2. What do we call the specialized neurons in the occipital lobe’s visual cortex that respond to particular edges, lines, angles, and movements? a. Rods b. Cones c. Bipolar cells d. Feature detectors e. Ganglion cells 3. Which of the following structures helps you most in detecting the color of your friend’s shirt? a. Rods b. Cones c. Fovea d. Lens e. Cornea 4. Your best friend decides to paint her room an extremely bright electric blue. Which of the following best describes the physical properties of the color’s light waves? a. No wavelength; large amplitude b. Short wavelength; large amplitude c. Short wavelength; small amplitude d. Long wavelength; large amplitude e. No wavelength; small amplitude 5. If you scratch your eye, which structure are you most likely to damage? a. Pupil b. Iris c. Cornea d. Lens e. Fovea Practice FRQs 532 1. As light reflected off an object reaches your eye, it passes through several structures before it reaches the retina. Describe the functions of the following structures: Cornea Iris Lens (3 points) Answer 1 point: The cornea bends and focuses the light waves. Page 177 1 point: The iris can expand or contract to allow more or less light to pass through the pupil. Page 177 1 point: The lens changes shape to help focus images on the retina. Page 177 2. Yasser is colorblind and is unable to see colors the same way as his wife. Describe which theory of color vision best explains this deficiency and explain which theory of color vision best explains why he might see an afterimage. (2 points) 533 Module 19 Visual Organization and Interpretation LEARNING TARGETS 19-1 Describe the Gestalt psychologists’ understanding of perceptual organization, and explain how figure-ground and grouping principles contribute to our perceptions. 19-2 Explain how we use binocular and monocular cues to perceive the world in three dimensions, and discuss how we perceive motion. 19-3 Explain how perceptual constancies help us construct meaningful perceptions. 19-4 Describe what research on restored vision, sensory restriction, and perceptual adaptation reveals about the effects of experience on perception. 534 Perceptual Organization Flip It Video: Gestalt Psychology 19-1 How did the Gestalt psychologists understand perceptual organization, and how do figure-ground and grouping principles contribute to our perceptions? It’s one thing to understand how we see colors and shapes. But how do we organize and interpret those sights (or sounds, or tastes, or smells) so that they become meaningful perceptions—a rose in bloom, a familiar face, a sunset? Early in the twentieth century, a group of German psychologists noticed that people who are given a cluster of sensations tend to organize them into a gestalt, a German word meaning a “form” or a “whole.” As we look straight ahead, we cannot separate the perceived scene into our left and right fields of view (each as seen with one eye closed). Our conscious perception is, at every moment, a seamless scene—an integrated whole. gestalt an organized whole. Gestalt psychologists emphasized our tendency to integrate pieces of information into meaningful wholes. Consider Figure 19.1: The individual elements of this figure, called a Necker cube, are really nothing but eight blue circles, each containing three converging white lines. When we view these elements all together, however, we see a cube that sometimes reverses direction. This phenomenon nicely illustrates a favorite saying of Gestalt psychologists: In perception, the whole may exceed the sum of its parts. 535 Figure 19.1 A Necker cube What do you see: circles with white lines, or a cube? If you stare at the cube, you may notice that it reverses location, moving the tiny X in the center from the front edge to the back. At times, the cube may seem to float forward, with circles behind it. At other times, the circles may become holes through which the cube appears, as though it were floating behind them. There is far more to perception than meets the eye. (From Bradley et al., 1976.) Over the years, the Gestalt psychologists demonstrated many principles we use to organize our sensations into perceptions (Wagemans et al., 2012a,b). Underlying all of them is a fundamental truth: Our brain does more than register information about the world. Perception is not just opening a shutter and letting a picture print itself on the brain. We filter incoming information and construct perceptions. Mind matters. Form Perception Imagine designing a video-computer system that, like your eye-brain system, could recognize faces at a glance. What abilities would it need? Figure and Ground 536 To start with, the system would need to perceive figure-ground—to separate faces from their backgrounds. In our eye-brain system, this is our first perceptual task—perceiving any object (the figure) as distinct from its surroundings (the ground). As you read, the words are the figure; the white space is the ground. T