Jamming Avoidance Response of Weakly Electric Fish Eigenmannia PDF
Document Details
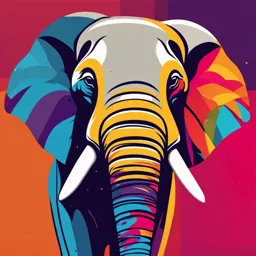
Uploaded by CrispOrchid
State University of New York at Buffalo
Tags
Summary
This document covers the jamming avoidance response of the weakly electric fish Eigenmannia. It details the electric organ discharge (EOD), electrolocation, and electroreceptor function involved in this response. The document also explains how fish adjust their EOD frequency to prevent interference.
Full Transcript
Chapter 8 Jamming Avoidance Response of Weakly Electric Fish Eigenmannia Model systems focus on components of sensory processing and motor function Toads and prey detection Bats and echolocation Barn owls and sound loca...
Chapter 8 Jamming Avoidance Response of Weakly Electric Fish Eigenmannia Model systems focus on components of sensory processing and motor function Toads and prey detection Bats and echolocation Barn owls and sound localization Introduction One of the few model systems where the entire neural pathway involved in generating sensory guided behavior and sensorimotor integration is…… Jamming Avoidance Response in Eigenmannia The Electric Organ Discharge (EOD) in Eigenmannia is the most stable biological oscillator in nature It’s an excellent model system for studying questions of neural coding: temporal coding, rate coding, spike time variability, information transmission, etc. Knifefish Eigenmannia virescens Teleost belonging to order Gymnotiformes 61 different species Fresh water fish in South and Central America Breeds during tropical rainy season Females defend nesting sites in floating weeds Males fiercely territorial and will butt or bite opponents Dominant males use lower frequencies Can produce electric field Achieved by discharging an electric organ in tail The Electric Organ Electric Organ composed of modified muscle cells called electrocytes Exist at end of axons but unable to contract Cylindrical cells with flattened areas of electrically excitable membrane Maintain potential gradient across membrane by Na+/K+ pump When not in use, retain negative charge inside Upon stimulation (acetylcholine), innervated side of cell becomes depolarized before uninnervated side. Use nicotinic acetylcholine receptors Ion channel open on innervated side Na+ influx followed by K+ efflux occurs Gives rise to temporary potential gradient on each membrane Potential gradient is then discharged Each cell produces discharge of tens of millivolts Are arranged in series leading to synchronous depolarization leading to total potential of ~1V Michael R. Markham, Rüdiger Krahe, Eric Fortune; Electrocyte physiology: 50 years later. J Exp Biol1 July 2013; 216 (13): 2451–2458. Used for electrolocation and communication Electric Organ Discharges 200-500 times/sec Corresponds to 200-500Hz Species specific For knifefish, waveform resembles train of sine waves Determined by endogenous oscillator in medulla Pacemaker Nucleus One volley of spikes down spinal cord generates one discharge cycle Frequency of discharge extremely regular Electroreceptors Can sense their own and other fish electric discharges via electroreceptors Distributed all over body, most abundant around head (up to 15 per one square mm of skin) Electroreceptors located in two types of organs: Tuberous Ampullary Both contain 25-35 small receptor cells at base Electroreceptors synapse at base of pit with sensory neuron that sends axon into brain Electrolocation ”Seeing” with the body surface Fish constantly surrounded by its own electric field Electric signals of neighbors are perceived as perturbations in feedback from own discharges Objects in vicinity distort the electric field and alter current pattern Electric image Alteration in the current pattern on the fish’s body surface Problems arise if neighboring fish with similar discharge frequency to fish’s own come close Two electric fields interfere with each other Jamming Results in phase and amplitude modulations of each of the electric signals Effect most detrimental if difference between fish’s and neighbors frequency is 20Hz Avoidance To avoid, fish shift own frequency away from neighbor’s frequency Jamming Avoidance Response Response If neighbor’s frequency higher, fish will lower its discharge frequency If neighbor’s frequency lower, fish will raise its discharge frequency Fish able to determine sign of the frequency difference (Df) Neighbor’s frequency – fish’s frequency Determination of Sign of Frequency Difference Without Internal Reference Fish do not make internal reference to frequency of pacemaker Fish needs to be exposed to mixture of its own signal and interfering signal on parts of body to execute jamming avoidance response Two electric fields have different geometries Causes electric current originating from neighboring fish to affect electroreceptors on different parts of body to different degrees Due to differences in angle and distance current of neighbor hits electroreceptors Variation in the mixing ratio of the currents critical for execution of jamming avoidance response Modulation of Amplitude and Phase Mixing of Sfish and Sneighbor causes modulation of amplitude and phase relative to pure Sfish signal Degree of modulation depends on amplitude ratio of two interfering signals If ratio is not 1:1, phase shift will cycle periodically within ”beat” cycle (difference between two signals) Fish use this information to determine who has higher frequency Critical for fish to: Extract sign of frequency difference by electrosensory processing Translate determination of sign of frequency difference into change in motor output (pacemaker frequency) Example: When fish’s own discharge has a higher frequency, zero crossing of combined signal is first lagging with amplitude decrease, then leads as amplitude increases Fish will determine this and its EOD frequency will be raised Electrosensory Processing I: Electroreceptors Ampullary Receptors Tuned to DC and low frequency signals Role in Jamming Response unclear Changing signals, particularly those with same frequency as fish’s own electric organ discharge Tuberous Receptors Tuned to AC signals in range of own electric organ discharge Detect static or slowly changing voltages Two subtypes P type Receptors “Probability coders” Fire intermittently and increase rate with rise in stimulus amplitude T type Receptors ”Time coders” Fire one spike on each cycle of stimulus (zero crossing) Electrosensory Processing II: Electrosensory Lateral Line Lobe Primary afferents of tuberous electroreceptors project to four maps of electrosensory lateral line lobe in hindbrain (medulla) Lateral Centrolateral Centromedial Medial Each map is somatotopically ordered Preserve spatial order of electroreceptors on body surface Information encoded by T-type and P-type receptors processed separately Parallel Processing Input from T-type receptors received by spherical cells One spherical cell connected by electric synapses Encode phase of stimulus (fine tune zero crossing information) Input from P-type receptors received by Basilar pyramidal cell (excitatory input from P type receptors) Non basilar pyramidal cells (inhibitory input from P type receptors) Excitation of basilar pyramidal cells reflects rise in amplitude of stimulus signal Excitation of non basilar pyramidal cells indicates fall in stimulus amplitude Electrosensory Processing III: Torus Semicircularis Located in Midbrain (like inferior colliculus) Divided into various laminae (topographically organized) Phase coding spherical cells of electrosensory lateral line lobe project to lamina 6 exclusively Phase advanced and phase delay neurons (timing information) Amplitude coding pyramidal cells send axons to lamina 3, 5, 7, & 8 Convergence of amplitude and phase information achieved by vertical connections between different layers Sign selective neurons Higher Frequency EOD from neighbor Phase advance coupled with amplitude decrease Phase delay coupled with amplitude increase Lower Frequency EOD from neighbor Phase delay coupled with amplitude decrease Phase advance coupled with amplitude increase Diencephalon Electrosensory Cells code sign of frequency difference between fish’s signal and neighbor here Processing IV: Sensitivity to phase modulations increases significantly Nucleus Sign selective neurons here resemble jamming avoidance response itself Electrosensorius Essential for execution of jamming avoidance response Nucleus Electrosensorius Electrosensory Acceleration of EOD Innervates central posterior/prepacemaker nucleus in Processing IV: dorsal thalamus Excitatory connection Nucleus Nucleus Electrosensorius Deceleration of EOD Electrosensorius Innervates sublemniscal prepacemaker nucleus in mesencephalon Inhibitory (GABA) connection Final Command for altering fish’s own EOD generated here Central Posterior Nucleus (CP) of the Prepacemaker nucleus (PPnG) Motor Control via Area is excited by nE if fish should increase EOD Gradual frequency rises the Prepacemaker Innervate (via glutamate/AMPA receptor) pacemaker cells Sublemniscal Prepacemaker Nucleus (SPPn) Nucleus Area is inhibited by GABA from nE if fish should decrease EOD Innervate (via glutamate/NMDA receptor) relay cells within pacemaker nucleus Pacemaker Cells Motor Control via the Medulla Oblongata Pacemaker Nucleus Drive relay cells Transmit command pulses generated by pacemaker cells to spinal and Relay Cells motor neurons of electric organ Leads to discharge of electric organ (electrocytes) The Complete Circuit