Auditory & Vestibular Systems PDF Fall 2024
Document Details
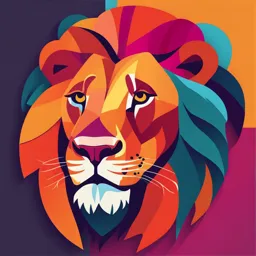
Uploaded by KindlyElegy
2024
Tags
Summary
These lecture notes cover the auditory and vestibular systems, including detailed explanations of the anatomy of the ear, the physics of sound, and the transmission and processing of auditory and vestibular information. They also discuss the physiology of vestibular processing and various related topics, including how sounds are detected and perceived.
Full Transcript
Auditory & Vestibular Systems Chapter 11 Discuss the anatomy of the ear, which serves as the home for receptors of the auditory & vestibular systems Learn about the physics of sound and how it is transduced Learning in the ear objectives Unde...
Auditory & Vestibular Systems Chapter 11 Discuss the anatomy of the ear, which serves as the home for receptors of the auditory & vestibular systems Learn about the physics of sound and how it is transduced Learning in the ear objectives Understand the transmission of auditory information to the brain & how it is processed in the brain Discuss the physiology of vestibular processing in the ear & its information pathway to the brain Both our hearing and our sense of balance involve the ear as the main receptive organ Sense of hearing: audition/auditory system Detect sound Perceive and interpret nuances Sense of balance: vestibular system Head and body location Introduction Head and body movements The ear has three major areas: External (outer) ear: hearing only Middle ear (tympanic cavity): hearing only Internal (inner) ear: hearing and equilibrium Receptors for hearing and balance respond to separate stimuli and are activated independently Anatomy of the ear The external (outer) ear consists of two parts: Auricle (pinna): shell-shaped structure surrounding ear canal that functions to funnel sound waves into auditory canal External acoustic meatus (auditory canal) Short, curved tube lined with skin bearing hairs, sebaceous glands, and External/outer ceruminous (earwax) glands ear Transmits sound waves to eardrum Tympanic membrane (eardrum) Boundary between external and middle ears Thin, translucent connective tissue membrane Vibrates in response to sound Transfers sound energy to bones of middle ear A small, air-filled, mucosa-lined cavity in temporal bone Flanked laterally by eardrum and medially by bony wall containing oval and round membranous windows Pharyngotympanic (auditory) tube: connects middle ear to Middle ear nasopharynx Formerly called eustachian (tympanic tube Usually flattened tube, but can cavity) be opened by yawning or swallowing to equalize pressure in middle ear cavity with external air pressure Tympanic membrane cannot vibrate efficiently if pressures on both sides are not equal, Pharyngotympanic (auditory) tube causing distorted sounds Often the site of inflammation for ear infections (common in children) Auditory ossicles: three small bones in tympanic cavity, named for their shape: Malleus: the ”hammer” is secured to eardrum Incus: the “anvil” Middle ear Stapes: the “stirrup” base fits into oval window (tympanic Synovial joints allow malleus to articulate with incus, which cavity) articulates with stapes Suspended by ligaments; transmit vibratory motion of eardrum to oval window Tensor tympani and stapedius muscles contract reflexively in response to loud sounds to prevent damage to hearing receptors Auditory ossicles: three small bones in tympanic cavity, named for their shape: Malleus: the ”hammer” is secured to eardrum Incus: the “anvil” Middle ear Stapes: the “stirrup” base fits into oval window (tympanic Synovial joints allow malleus to articulate with incus, which cavity) articulates with stapes Suspended by ligaments; transmit vibratory motion of eardrum to oval window Tensor tympani and stapedius muscles contract reflexively in response to loud sounds to prevent damage to hearing receptors Located in temporal bone behind eye socket Two major divisions: Bony labyrinth: system of channels and cavities that worm through the bone Divided into three regions: vestibule, semicircular canals, and cochlea Filled with perilymph fluid; similar to CSF Membranous labyrinth; series of membranous sacs and ducts contained in bony labyrinth; filled with potassium-rich endolymph Inner ear (labyrinth) Located in temporal bone behind eye socket Two major divisions: Bony labyrinth: system of channels and cavities that worm through the bone Divided into three regions: vestibule, semicircular canals, and cochlea Filled with perilymph fluid; similar to CSF Membranous labyrinth; series of membranous sacs and ducts contained in bony labyrinth; filled with potassium-rich endolymph Inner ear (labyrinth) Semicircular canals Three canals oriented in three planes of space: anterior, lateral, and posterior Anterior and posterior are at right angles to each other, whereas the lateral canal is horizontal Membranous semicircular ducts line each canal and communicate with utricle Ampulla: enlarged area of ducts of each canal that houses equilibrium receptor region called the crista ampullaris Receptors respond to angular (rotational) movements of the head Inner ear (labyrinth) Vestibule Central egg-shaped cavity of bony labyrinth Contains two membranous sacs Saccule is continuous with cochlear duct Utricle is continuous with semicircular canals Sacs house equilibrium receptor regions (maculae) that respond to gravity and changes in position of head Inner ear (labyrinth) Cochlea A small spiral, conical, bony chamber, size of a split pea Extends from vestibule Contains cochlear duct, which houses spiral organ (organ of Corti) and ends at cochlear apex Inner ear (labyrinth) Cavity of cochlea divided into three chambers: Scala vestibuli: abuts oval window, contains perilymph Scala media (cochlear duct): contains endolymph Scala tympani: Inner ear terminates at round window; contains (labyrinth) perilymph Scalae tympani and vestibuli are continuous with each other at helicotrema (apex) Vestibular membrane: “roof” of cochlear duct that separates scala media from scala vestibuli Endocochlear potential: endolymph liquid (only found in scala media) has Inner ear an electrical potential 80 mV more positive than (labyrinth) perilymph, due to being rich in cations (K+ and Ca2+) Spiral organ contains cochlear hair cells functionally arranged in one row of inner hair cells (pitch) and three rows of outer hair cells(adjust Inner ear volume of what we hear) Hair cells are (labyrinth) sandwiched between tectorial and basilar membranes The cochlear branch of nerve VIII runs from spiral organ to brain Spiral organ contains cochlear hair cells functionally arranged in one row of inner hair cells and three rows of outer hair cells Hair cells are sandwiched between tectorial and basilar membranes The cochlear branch of nerve VIII runs from spiral organ to brain Inner ear (labyrinth) Sound is audible variations in air pressure What is Cycle: distance between successive compressed patches of air sound? Sound frequency: number of cycles per second expressed in hertz (Hz) Hearing is the reception of an air sound wave that is converted to a fluid wave that ultimately stimulates mechanosensitive cochlear hair cells that send impulses to the brain for interpretation Detection of sound Frequency Number of waves that pass given point in a given time Wavelength Distance between two consecutive crests Shorter wavelength = higher frequency of sound Sound can be Wavelength is consistent for a particular sound described by frequency and amplitude Frequency range of human hearing is 20–20,000 hertz (Hz = waves per second), but most sensitive between 1500 and 4000 Hz Pitch: perception of different frequencies Higher the frequency, higher the pitch Quality: characteristic of sounds Sound can be Most sounds are mixtures of different frequencies Tone: one frequency (ex: tuning fork) described by Sound quality provides richness and complexity of sounds (music) frequency and amplitude Amplitude Height of crests Amplitude perceived as loudness: subjective interpretation of sound intensity Measured in decibels (dB) Normal range is 0–120 decibels (dB) Normal conversation is around 50 dB Sound can be Threshold of pain is 120 dB Severe hearing loss can occur with prolonged exposure above 90 dB described by Amplified rock music is 120 dB or more frequency and amplitude 1. Sound waves 2. Tympanic membrane Auditory 3. Ossicles pathway at a 4. Oval window glace 5. Cochlear fluid 6. Sensory neuron response Pathway of sound 1. Tympanic membrane: sound waves enter external acoustic meatus and strike tympanic membrane, causing it to vibrate The higher the intensity, the more vibration Transmission of sound to the internal ear Pathway of sound 1. Tympanic membrane: sound waves enter external acoustic meatus and strike tympanic membrane, causing it to vibrate The higher the intensity, the more vibration 2. Auditory ossicles: transfer vibration of eardrum to oval window Tympanic membrane is about 20´ larger than oval window, so vibration transferred to oval window is amplified about 20´ Transmission of sound to the internal ear Pathway of sound 1. Tympanic membrane: sound waves enter external acoustic meatus and strike tympanic membrane, causing it to vibrate The higher the intensity, the more vibration 2. Auditory ossicles: transfer vibration of eardrum to oval window Tympanic membrane is about 20´ larger than oval window, so vibration transferred to oval window is amplified about 20´ 3. Scala vestibuli: stapes rocks back and forth on oval window with each vibration, causing wave motions in perilymph Transmission Wave ends at round window, causing it to bulge outward into middle ear cavity of sound to the internal ear Pathway of sound 1. Tympanic membrane: sound waves enter external acoustic meatus and strike tympanic membrane, causing it to vibrate The higher the intensity, the more vibration 2. Auditory ossicles: transfer vibration of eardrum to oval window Tympanic membrane is about 20´ larger than oval window, so vibration transferred to oval window is amplified about 20´ 3. Scala vestibuli: stapes rocks back and forth on oval window with each vibration, causing wave motions in perilymph Wave ends at round window, causing it to bulge outward into middle ear cavity Transmission 4. Basilar membrane: sounds in hearing range go through cochlear duct, vibrating basilar membrane at specific location, according to frequency of sound of sound to the internal ear Resonance: movement of different areas of basilar membrane in response to a particular frequency Basilar membrane changes along its length: Fibers near oval window are short and stiff Resonate with high-frequency waves Fibers near cochlear apex are longer, floppier Resonate with lower-frequency waves Resonance of So basilar membrane mechanically processes sound even before the basilar signals reach receptors membrane Resonance: movement of different areas of basilar membrane in response to a particular frequency Basilar membrane changes along its length: Fibers near oval window are short and stiff Resonate with high-frequency waves Fibers near cochlear apex are longer, floppier Resonate with lower-frequency waves Resonance of So basilar membrane mechanically processes sound even before the basilar signals reach receptors membrane Basilar membrane changes along its length: Fibers near oval window are short Resonance of and stiff Resonate with the basilar high-frequency waves membrane Fibers near cochlear apex are longer, floppier Resonate with lower-frequency waves Excitation of inner hair cells Bending of stereocilia toward tallest ones pull on tip links, causing K+ and Ca2+ ion channels in shorter stereocilia to open K+ and Ca2+ flow into cell, causing receptor potential that can lead to release of neurotransmitter (glutamate) Can trigger AP in afferent neurons of cochlear nerve Bending of stereocilia toward shorter ones causes tip links to relax Ion channels close, leading to repolarization (and even hyperpolarization) Sound transduction Nerve fibers coiled around hair cells of outer row are efferent neurons that convey messages from brain to ear Outer hair cells can contract and stretch, which changes stiffness of basilar membrane This ability serves two functions: Increase “fine-tuning” responsiveness of inner hair cells by amplifying motion of basilar membrane Protect inner hair cells from loud noises by decreasing motion of basilar membrane Role of outer hair cells Neural impulses from cochlear bipolar cells reach auditory cortex via following pathway: Spiral ganglion ® Cochlear nuclei (medulla) ® Transmission Superior olivary nucleus (pons-medulla) ® of auditory Lateral lemniscus (tract) ® information to Inferior colliculus (midbrain auditory reflex center ® the brain Medial geniculate nucleus (thalamus) ® Primary auditory cortex Some fibers cross over, some do not; so both auditory cortices receive input from both ears Perception of pitch: impulses from hair cells in different positions along basilar membrane are interpreted by brain as specific pitches Detection of loudness is Processing of determined by brain as an increase in the number of action auditory potentials (frequency) that result information in when hair cells experience larger deflections the brain Localization of sound depends on relative intensity and relative timing of sound waves reaching both ears If timing is increased on one side, brain interprets sound as coming from that side Characteristic frequency: frequency at Response which a neuron is most responsive—from cochlea to cortex properties of Response properties more complex and neurons in diverse beyond the brain stem auditory Binaural neurons are present in the superior olive. cortex Encoding information about stimulus intensity Firing rates of neurons Number of active neurons Encoding Membrane potential of activated sound hair cells more depolarized or hyperpolarized intensity Loudness perceived is correlated with number of active neurons. Tonotopic maps on the basilar membrane, spiral ganglion, and cochlear nucleus From the base to apex, basilar membrane resonates with increasingly lower frequencies. Tonotopy is preserved in the auditory nerve and cochlear nucleus. In cochlear nucleus, bands of cells with similar characteristic frequencies increase from anterior to posterior. Encoding sound frequency Action potentials are going to be synchronized to specific parts of the frequency wave (e.g., peak of depolarization phase of action potential locked onto peak of sound wave or something similar) Low frequencies: phase locking Phase locking on every cycle or some fraction of cycles High frequencies: not fixed (likely too fast/chaotic to reliably synchronize) Thought to be important for sound localization/detailed sound processing Localization of sound in horizontal plane Interaural time delay: difference in time for sound to reach each ear Interaural intensity difference: sound at one Mechanisms ear less intense because of head’s sound shadow of sound Duplex theory of sound localization localization: Low frequency sounds based on interaural time delay: 20–2000 Hz High frequency sounds based on interaural intensity difference: 2000–20,000 Hz Coincidence detection principle: Sound from left side, activity in left cochlear nucleus sent to Mechanisms superior olive of sound Sound delayed to right ear, activity in right cochlear localization nucleus Impulses reach olivary neuron at the same time à summation à action potential Mechanisms Vertical sound localization of sound based on reflections from the pinna localization Tonotopy: columnar organization of cells with similar binaural interaction Unilateral lesion in auditory cortex: almost normal auditory function (unlike lesion in striate cortex: complete blindness in one visual hemifield) Different frequency bands processed in parallel Neuronal response properties Frequency tuning in neurons: similar characteristic frequency Isofrequency bands running mediolaterally across A1 cortex Primary auditory cortex Remember our association areas? Auditory association area will help with further interpretation of sounds (pattern recognition, emotional association, etc.) Beyond primary auditory cortex Overview of auditory pathway https://www.youtube.com/watch?v=yza-hpIubxQ Video on sound localization Conduction deafness Blocked sound conduction to fluids of internal ear Causes include impacted earwax, perforated eardrum, otitis media, otosclerosis of the ossicles Sensorineural deafness Damage to neural structures at any point from cochlear hair cells to auditory cortical cells Typically from gradual hair cell loss Tinnitus Clinical Ringing, buzzing, or clicking sound in ears in absence of auditory stimuli Due to cochlear nerve degeneration, inflammation of middle or internal ears, side effects connection: of aspirin sense of hearing Cochlear implants can help deaf patients by substituting the electrophysiology of normal cochlear hair cells Clinical connection: sense of hearing Equilibrium is response to various movements of head that rely on input from inner ear, eyes, and stretch receptors Vestibular apparatus: equilibrium receptors in semicircular canals and vestibule Vestibular receptors monitor static equilibrium Semicircular canal receptors monitor dynamic equilibrium Sense of equilibrium (vestibular system) Equilibrium is response to various movements of head that rely on input from inner ear, eyes, and stretch receptors Vestibular apparatus: equilibrium receptors in semicircular canals and vestibule Vestibular receptors monitor static equilibrium Semicircular canal receptors monitor dynamic equilibrium Sense of equilibrium (vestibular system) Receptor for rotational acceleration is crista ampullaris (crista) Small elevation in ampulla of each semicircular canal Cristae are excited by acceleration and deceleration of head Major stimuli are rotational (angular) movements, such as twirling of the Dynamic body Semicircular canals are located in all three planes of space (pitch, roll, equilibrium yaw), so cristae can pick up on all rotational movements of head sensors (cristae ampullares in semicircular canals) Receptor for rotational acceleration is crista ampullaris (crista) Small elevation in ampulla of each semicircular canal Cristae are excited by acceleration and deceleration of head Major stimuli are rotational (angular) movements, such as twirling of the Dynamic body Semicircular canals are located in all three planes of space (pitch, roll, equilibrium yaw) so cristae can pick up on all rotational movements of head sensors (cristae ampullares in semicircular canals) Anatomy of a crista ampullaris Each crista has supporting cells and hair cells that extend into gel-like mass called ampullary cupula Dendrites of vestibular nerve fibers encircle base of hair cells Activating receptors of crista ampullaris Dynamic Cristae respond to changes in velocity of rotational movements of head Inertia in ampullary cupula causes endolymph in semicircular ducts to equilibrium move in direction opposite body’s rotation, causing hair cells to bend sensors (cristae ampullares in semicircular canals) Bending hairs in cristae causes depolarization Rapid impulses reach brain at faster rate Activation of crista ampullares Bending of hairs in opposite direction causes hyperpolarizations Fewer impulses reach brain Activation of crista ampullares Rotational acceleration is a fast-adapting sense (after a few seconds, the static equilibrium sensors are responsible for informing equilibrium state) Activation of crista ampullares Push-pull Three semicircular canals on each side Help sense all possible head rotation activation of angles semicircular Each paired on opposite side of head. canals Push–pull activation of vestibular axons There are two maculae inside the Static vestibular system: Utricle senses horizontal equilibrium movements (e.g. tilting head, accelerating in a car) sensors Hair cells point vertically Saccule senses vertical (maculae) movements (e.g. acceleration of an elevator) Hair cells point horizontally Anatomy of a macula Each is a flat epithelium patch containing hair cells with supporting cells Static Hair cells have stereocilia and special “true stereocilium” called equilibrium kinocilium sensors Located next to tallest stereocilia Stereocilia are embedded in (maculae) otolith membrane, jelly-like mass studded with otoliths (tiny CaCO3 stones) Otoliths increase membrane’s weight and increase its inertia (resistance to changes in motion) Anatomy of a macula Each is a flat epithelium patch containing hair cells with supporting cells Static Hair cells have stereocilia and special “true stereocilium” called equilibrium kinocilium sensors Located next to tallest stereocilia Stereocilia are embedded in (maculae) otolith membrane, jelly-like mass studded with otoliths (tiny CaCO3 stones) Otoliths increase membrane’s weight and increase its inertia SEM image of otoliths in ear (resistance to changes in motion) Hair cells release neurotransmitter continuously Acceleration/deceleration causes a change in amount of neurotransmitter released Leads to change in AP frequency to brain Density of otolith membrane Activation of causes it to lag behind movement of hair cells when maculae head changes positions Base of stereocilia moves at same rate as head, but tips embedded in otolith are pulled by lagging membrane, causing hair to bend Ion channels open, and depolarization occurs Bending of hairs in direction of kinocilia: Depolarizes hair cells Increases amount of neurotransmitter release More impulses travel up vestibular nerve to brain Bending of hairs away from Activation of kinocilia: Hyperpolarizes receptors maculae Less neurotransmitter released Reduces rate of impulse generation Thus brain is informed of changing position of head Extremely slow, arguably never-adapting sense Main pathway – receptors à vestibulocochlear nerve (VIII) à vestibular nuclei in medulla and pons of brainstem Central Many additional “stops” such as cerebellum, thalamus, motor centers vestibular pathway The vestibular receptors work together to inform your brain of equilibrium and rotational motion Equilibrium information goes to reflex centers in brain stem Allows fast, reflexive responses to imbalance so we don’t fall down Impulses from activated vestibular receptors travel to either vestibular nuclei in brain stem or to cerebellum Three modes of input for balance and orientation: Transmission Vestibular receptors of equilibrium Visual receptors Somatic receptors information to the brain Vestibulo- ocular reflex is Function: fixate line of sight on visual target during head a great movement example of the Mechanism: senses rotations of neural head, commands compensatory movement of eyes in opposite coordination direction between Connections from semicircular canals, to vestibular nucleus, to vestibular, cranial nerve nuclei à excite somatic, visual extraocular muscles receptors If the visual, vestibular, and proprioceptive systems all work together to convey the sense of equilibrium in the body, what might happen if any of these components aren’t in sync with each other? Clinical Motion sickness - sensory inputs are connection: mismatched Visual input differs from equilibrium input sense of Conflicting information causes motion equilibrium sickness Warning signs are excess salivation, pallor, rapid deep breathing, profuse sweating Treatment with antimotion drugs that depress vestibular input, such as meclizine and scopolamine Clinical connection: Vertigo is another sense of common vestibular issue equilibrium Note the Auditory system parallels visual system parallels Tonotopy (auditory) or retinotopy (visual) preserved from sensory cells to cortex between Convergence of inputs from lower levels à neurons at higher levels have more complex responses. visual and Higher level visual neurons binocular auditory Higher level auditory neurons binaural systems Vestibular system receptor names & orientations (i.e., if you Quiz hint! move your head / body in a certain direction or motion, which receptors in which part of the system detect it?) Questions?