Anatomy and Physiology of the Ear PDF
Document Details
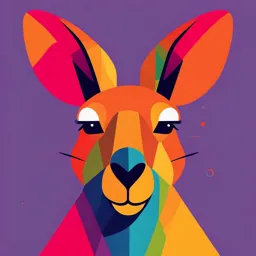
Uploaded by SprightlyTaiga5710
Tags
Summary
This document appears to be a detailed overview of the anatomy and physiology of the ear. It explores the structure and function of the outer, middle, and inner ear, covering topics such as the tympanic membrane, the cochlea, the auditory cortex, and the vestibular system. The document uses diagrams and tables to highlight key anatomical features and related physiological processes.
Full Transcript
Table of Contents The Outer Ear 5 The Middle Ear 7 The Temporal Bone 11 The Inner Ear 12 Inner Ear Physiology 17 Endocochlear Potential 19 The Acoustic Nerve 23 The Cochlear Nucleus 26 The Superior Olivary Complex 28 The Lateral Lemniscus 30 The Medial Geniculate Body and Audito...
Table of Contents The Outer Ear 5 The Middle Ear 7 The Temporal Bone 11 The Inner Ear 12 Inner Ear Physiology 17 Endocochlear Potential 19 The Acoustic Nerve 23 The Cochlear Nucleus 26 The Superior Olivary Complex 28 The Lateral Lemniscus 30 The Medial Geniculate Body and Auditory Thalamus 31 The Inferior Colliculus 32 The Auditory Cortex 33 The Corpus Collosum 36 Vascularization of the Auditory System 38 The Efferent System 40 The Vestibular Anatomy 42 The Eyes (Vestibular) 45 Meniere\'s disease: Anatomy of Inner Ear \ Standard Anatomical Terms and Planes  - Anatomy - 15-30 degree protrusion - Contains some vestigial muscles - Cartilaginous - Physiology - Collects/funnels sound - Most effective function for high frequencies (around 5000 Hz) because their wavelengths are smaller than the pinna, so the waves cannot pass around it as easily - Complex resonator for high frequencies due to ridges and depressions of the pinna---key for horizontal localization - Effect of spectral cues are also key for vertical and back/front localization. - Binaural earmolds hurt elevation localization for this reason External Auditory Meatus - Anatomy - 2.5-3 cm in length for adults - Diameter decreases as you approach isthmus - Slight downward tilt in adults so that water doesn't get trapped, but this is more horizontal in kids---thus, more susceptible to trapping foreign objects - Outer 1/3 is cartilage, inner 2/3 is bone - Bony portion is formed by three structures - 1\. Tympanic portion of temporal bone (floor, anterior wall, inferior-posterior wall) - 2\. Squamous portion of temporal bone (roof, part of posterior wall) - 3\. Condyle of the mandible (inferior-anterior wall) - At birth, no bone only the tympanic annulus (cartilaginous ring). Osseous maturation not complete until age 3 - Collapsed canals= high frequency hearing loss - Atresia: partial or complete lack of an ear canal - Epidermal lining in ear canal and lateral layer of TM - Cerumen: waxy substance secreted by epidermal glands - Moisturizes epidermal lining - Only produced in the outer 1/3 of canal - Secreted by two types of cells - 1\. Sebaceous cells---oily lubricant creator, created by passive cell breakdown - 2\. Ceruminous glands---produce wax-like substance - Combination of two leads to different Cerumen textures - Outer layers of skin migrate and push cerumen out - Can impact and harden if pushed medially (can be caused by q-tips and overproduction of wax) - Slight antibacterial and antifungal and may repel/discourage insects from entering the ear - Impaction of cerumen is most prevalent in older men because of excessive hair growth with aging - Lots of nerve innervation (Vth, VIIth, Ixth, and Xth) - Tactile stimulation may cause cough (glossopharyngeal nerve, Ixth) - Mechanical stimulation may cause heart/blood circulation which, in really sensitive people, may cause fainting (glossopharyngeal and vagus nerves, Ixth and Xth) - Physiology - Acoustic resonator---the ear canal is a tube, so it resonates frequencies with wavelength 4x greater than length. EAM is 2.5-3 cm in length and has one open end and one closed end, so it is a quarter wave resonator. Therefore, it boosts frequencies with wavelengths of 10-12 cm which is 3500-4000 Hz. - This boost is 10-15 dB in the 3-4kHz range - The closed end, the TM, is compliant which reflects some sound and transmits some sound---this modifies the effective length of the tube and has a dampening effect. Because of this, the EAM resonates over a wider frequency range than a hard-ended tube. Directional Effects - The EAM and pinna resonance/properties affects sound, as does refractions and reflections of sound by the head and body. - Head shadow effects primarily high frequencies with wavelengths too short to pass around the head (ILDs) - Low frequencies localization is more effected by ITDs. The Middle Ear The Middle Ear Space Anatomy - Narrow, elongates space in the temporal bone: 2 cubic cm volume - Two main areas - 1\. Tympanic cavity proper--- lower portion of the cavity - 2\. Attic---upper portion, houses significant portion of two ossicles - Boundaries - Lateral---TM and squamous portion of the temporal bone - Superior---tegmen tympani (thin bone plate separating the ME and cranium) - Inferior---tympanic plate of temporal bone (separates ME and jugular fossa, which is a groove in the temporal bone that the jugular vein passes through) - Anterior---carotid wall (thin bone plate with holes for ET and orifice for tensor tympani muscle) - Medial---dense portion of temporal bone that houses the inner ear - Also, on this wall is the promontory (prominence from lateral projection of the basal turn of the cochlea), the round window (opening into the basal turn of the cochlea), the oval window (opening into the vestibule of the inner ear), and the prominence of the facial nerve. - Contained, small volume of air in ME space, so acoustic impedance is governed by stiffness - Stiff objects are responsive to high frequencies and have increased resistance to low frequencies (i.e. low frequency attenuation) - Tympanometry: Low frequency (226 Hz) probe tone used while pressure is shifted in EAM. The low frequency tone has increased opposition to the flow of energy, so it best shows changes in stiffness (and, therefore, changes in compliance). This shows the pressure that is the maximum compliance of the TM and ME system. Pathologies like ME fluid change acoustic impedance, reflected in results. - Some ME pathologies - Cholesteatoma: ME cyst that often forms due to long-standing negative pressure that causes a retraction pocket in the posterior-superior quadrant of the TM. Tympanograms often have negative pressure and/or reduced compliance with a CHL. - Otosclerosis: vascular otosponiosis that becomes reabsorbed and replaced with sclerotic plaques. This "fixes" the stapes over time. Increase in ME stiffness=low frequency CHL and As tympanograms. Also, Carhartt's "SNHL' notch at 2 kHz and a reddish-blue tint to TM due to increased vascularization. Tympanic Membrane - Cone-shaped with point medially - 0.1m thick with three layers - Outer layer: extension of the EAM epidermal layer - Inner layer: continuation of membranous labyrinth (mucosal lining) of the ME space. - Middle layer: fibrous layer that provides support from the TM - Two sets of fibers: radial and circular - Denser fibers around periphery and around where the manubrium of the malleus attaches to the TM - Landmarks - Sparser fibers at upper, superior part of the TM (pars flaccida) - The rest, majority, of the TM has denser fibers (pars tensa) - Annulus: fibrocartilaginous ring around the periphery of the TM - Umbo: tip of TM cone, displaced about 2 mm - At birth, the TM is positioned almost flat on canal floor. As the EAM lengthens, the TM becomes more erect. Infant TMs are very thin and flat. Ossicular Chain - Malleus - 3 main segments - 1\. Manubrium---attaches to the TM - 2\. Head---contains articular facet which is the point of connection to the incus - 3\. Neck---constriction in the bone between the manubrium and the head - Tensor tympani muscle attaches at the point where the manubrium meets the neck - Also has anterior process (between the head and manubrium) and lateral process (attaches to the upper TM) - Incus - 3 main segments - 1\. Body - 2\. Two processes (connects neck to footplate) - Short process---shares epitympanic recess with the head of the malleus - Long process---terminates in the lenticular process with articulates with the stapes - Stapes - 5 main segments - Head---tendon of stapedius muscles attaches here - Neck - Two processes---connect neck to footplate - Footplate---medial surface covered in hyaline cartilage that is fastened to the body wall of the oval window by the annular ligament - More held in posterior region of the footplate---leads to the movement being more of a pivoting/rocking not piston - Support for ossicular chain: supported by tendons of two muscles (tensor tympani and stapedius) and several ligaments Eustachian Tube - Runs from anterior wall of the ME cavity to the posterior wall of the nasopharynx - First 1/3 has a bony foundation, last 2/3 has a cartilage foundation - Isthmus: where bone and cartilage meet. The narrowest point of the ET. - At rest, the cartilaginous portion is closed but is opened by the levator veli palatini and tensor veli palatini muscles - Bony portion remains open - In young kids, the ET is wider and more horizontal Middle Ear Muscles - 1\. Tensor Tympani - Inserts through anterior wall to the malleus - Innervated by 5^th^ cranial nerve (trigeminal) - 2\. Stapedius - Inserts through posterior wall (pyramidal eminence) to the head of the stapes - Pulls stapes posterior on contraction - Innervated by 7^th^ nerve (facial) Effect of OE and ME on Sound Transmission - ME filter effects of enclosed volume of air = dampens low frequency sounds - Pinna resonance (small effect) and ear canal resonance (larger effect) = complex pattern of emphasis and de-emphasis of high frequency sounds - Overall---low frequencies are dampened, mid frequencies are enhanced by up to 15 dB, and high frequencies are dampened a little - dB HL scale is useful because of variability, sets "normal hearing" to 0 dB HL - ME Transformer - Impedance mismatch from air to IE fluid needs to be overcome - 3 ways ME overcomes: - 1\. Area differential between TM surface and oval window - Largest contribution - 17:1 - 2\. Lever action---malleus and incus form a lever. Manubrium of malleus is longer than the long process of the incus - 1.3:1 - 3\. Buckling of the curved TM causes energy to be funneled by curve down to the manubrium attachment - Area effect + lever effect + buckle effect = 46:1 (31-33 dB boost) The Temporal Bone - Bony Labyrinth: purpose is to provide support and protection for the delicate structures of hearing and balance - Membranous Labyrinth: lines the channels of the bony labyrinth to form the inner ear; includes fluids and hearing and balance end organs. - 4 main parts of temporal bone - 1\. Squamous: smooth lateral surface, attachment for temporal muscle. Has zygomatic process and the masseter muscle attached to the zygomatic arch. - 2\. Mastoid: attachment for occipital and postauricular muscles - PAM response can muddy MLR (occurs at 13-20 msec) - 3\. Petrous: most complex, houses peripheral hearing mechanisms - Most susceptible to fracture - Longitudinal (70%): extend from medial aspect of the temporal bone to the superior-posterior EAM. Usually causes by a blow to the temporal or parietal regions. Can result in SNHL, CHL, balance issues, and facial nerve damage. Hemotympanum and blood in EAM are signs. - Transverse (20-30%): extend across petrous pyramid through otic capsule and/or IAM. Profound SNHL and severe balance issues. Causes facial paralysis 50% of the time. - Contains IAM, vestibular aqueduct, jugular fossa, and jugular bulb - 4\. Tympanic The Inner Ear The Osseous Cochlea - In petrous portion of the temporal bone - Apex points toward the cheekbone - 1 cm wide, 5 mm tall - Modiolus: central perforated bony core that accommodates nerve fibers from hair cells and blood vessels - Medial end is continuous with the IAM - Osseous spiral lamina: shelflike structure that winds around the modiolus from base to apex - Lower shelf: support point and connector for the inner aspect of the basilar membrane and scala media (cochlear duct) - Upper shelf: continuous with spiral limbus and serves as attachment point and support point for the tectorial membrane - Habenula perforate: narrow holes in the spiral lamina that serves as a passage way for spiral ganglion neurons - Has two windows: - Oval window: at basal turn (superior of two windows), interacts with stapes and opens in scala tympani - Round window: inferior to oval window, opens in scala tympani - Insertion point for a cochlear implant - Premonitory: bony eminence between the two windows. It protects the windows because foreign bodies entering the ear strike this first - The site of needle electrode for transtympanic electrocochleography The Membranous Cochlea - Three ducts - 1\. Scala vestibuli (superior) \*oval window here - 2\. Scala media (a.k.a. the cochlear duct) - 3\. Scala tympani (inferior) \*round window here - Two dividing membranes - 1\. Basilar membrane: connected to the spiral ligament on outer wall and osseous spiral lamina on inner wall) - 2\. Reisner's membrane - Helicotrema: apex of cochlea, where scala vestibuli and scala tympani communicate - Ductus reuniens of Hensen: where the basal end of the scala media communicates with the saccule - Narrow, may be obliterated in some adults - May play a communicative role between cochlear and vestibular anatomy in endolymphatic disorders (ex. If blocked, cochlear hydrops may not spread to the vestibular system) - Perilymph - In the scala tympani and scala vestibuli - Same composition as cerebral spinal fluid - Low K+ and high Na+ - Corilymph - In the scala media, along with endolymph - Similar to perilymph---low K+ and high Na+ - Bathes the hair cells - Fills spaces of Nuel, external, and main tunnel of corti - Segregated from the endolymph by the reticular lamina - Endolymph - High K+, low Na+ (Composition maintained by the active process in the stria vascularis) - High K+ is key for cochlear function because K+ ions carry most of the hair cell transduction current - HCs have K+ selectivity ion channels - Low K+ in perilymph contributes to ion action  - The two aqueducts - 2\. Vestibular aqueduct - Endolymphatic - Encompasses the endolymphatic duct - Runs from wall of vestibule to the petrous pyramid of the temporal bone - Endolymphatic duct is meant to regulate endolymphatic pressure---implicated in endolymphatic hydrops - 2\. Cochlear duct - Perilymphatic - From basal turn of the scala tympani to the subarachnoid space near the jugular fossa - Allows for the transfer of the CSF - Closes later in life - Endolymphatic hydrops - Increased endolymph disrupts cochlear mechanics and distends (or ruptures) Reissner's membrane - Low frequency SNHL, tinnitus, vertigo, aural fullness - EcochG: SP amplitude approaching 50% of the AP amplitude indicates Meniere's Disease (with high variability) - Greater effect on apex because of the less stiff BM - Increased pressure could also make the apical BM stiffer, leading to increased TW velocity and earlier low-frequency ABR responses - Perilymph fistula - When membranes of the oval or round window are torn, it leaks perilymph into the ME, decreasing pressure in the scala vestibuli and tympani relative to the scala media - Progressive hearing loss and vestibular symptoms - Noise induced hearing loss - Often first effects OHCs and their stereocilia - Noise can damage three areas - 1\. Disruption of stereocilia through decoupling from the tectorial membrane - 2\. Loss of HC bodies - 3\. Damage to supporting cells - Possible pathogenesis - Reduced blood flow during exposure leading to hypoxia - Metabolic exhaustion of HCs - Excessive neurotransmitter release leading to nerve fiber damage - Reticular lamina damage leading to fluid mixing - Free radical damage - Synaptic ribbon damage (damage to IHC firing) - Both ototoxicity and NIHL can be attributed to free radical generation leading to HC death (with noise, high noise levels tax mitochondrial respiration processes, causing free radical formation - The Membranes - Basilar membrane - 25-35mm long in adults - Base: thicker, less wide, stiffer - Apex: thinner, wider, floppier - From base to apex, the stiffness decreases and mass increases - Attachment points are the osseous spiral lamina and the spiral ligament - Reissner's membrane - Upper boundary of scala media - Epithelial cells toward the endolymph side and mesothelial cells toward the perilymph side - Tectorial membrane - Above the organ of corti and covers its entire length - Articulates with the sterocilia of the OHC (tallest OHC cilia are embedded in notches) - Hensen's strip: bulge on the underside of the tectorial membrane above the IHC (restricts area between the IHC cilia and the tectorial membrane, leveraging Bernoulli's principle) - Attached between the spiral limbus and Hensen supporting cells - Reticular Lamina: the ceiling of the sensory and supporting cells of the organ of corti - Separates endolymph from internal structures of organ of corti which are bathed in cortilymph - Supports upper portion of the hair cells - Cilia penetrate reticular lamina (are bathed in endolymph) Lateral wall of the cochlear duct - Spiral ligament: between the otic capsule wall and stria vascularis---covers the lateral wall of scala media up to the upper scala tympani - Connective tissues that support BM and Reissner's membrane - Capillary bed for blood drainage - Regulates tension on BM - Helps maintain ionic balance - Stria vascularis - Secretory and absorptive functions - Three types of cells - Marginal cells: epithelial cells associated with ion channels and pumps - Intermediate: contains melanin/melanocytes and helps generate endocochlear potential - Basal cells: forms tight junction with intermediate cells and intermingles spiral ligament cells, supporting other cell layer functions - Overall: supplies blood and nutrients to cochlea and probably creates endolymph (with 80+ mV resting potential) - Sometimes implicated in endolymphatic hydrops because of over production or under absorption Hair Cells - Inner Hair Cells - Single row, about 3500 total - Stronger than OHC, flask shaped - More at base than apex (50:100) - About 20 type I spiral ganglion neurons make 1:1 contact with the base of each IHC - Lateral olivocochlear (LOC) efferent fibers contact type I fibers beneath the IHC - Outer Hair Cells - 3-5 rows, about 12000 total - May be more at the apex than the base - -60mV charge with K+ channels - Contractive proteins like prestin, actin, myosin, and tubulin for rapid expansion and contraction - More stereocilia at base than apex - Type II spiral ganglion neurons contact the base of many OHCs - Medial olivocochlear (MOC) efferent neurons also synapse with base - Kinocilium: tallest stereocilia - Stereocilia - Have pores that open on excitation (K+ ion entry) - Cilia are bathes in endolymph (with high K+ concentration and +80 mV charge) - Tip-links: small filaments that connect to mechanotransducer channels atop stereocilia and between the stereocilia to open K+ pores upon movement - Cross links: like tip links but on sides of cilia and help cilia move in concert Frequency Tuning - Base= high pitch - Apex= low pitch - Characteristic frequency: frequency to which the HC best respond - HCs are most sensitive to their CF but can respond to adjacent frequencies (physiologic tuning curves) Supporting Cells - Pharyngeal cells, Deiter cells, pillar cells Hensen cells, Claudian cells, Bottcher cells, and inner and outer boarder cells Regeneration of Hair Cells from Endogenous Otic Progenitors in the Adult Mammalian Cochlea: Understanding Its Origins and Future Directions Inner Ear Physiology Traveling Wave - Vibratory input from stapes in oval window displaces the noncompressible cochlear fluids, terminating in compensatory bulge of the round window - Originates in the scala vestibuli - Compression= the BM moves down - Rarefaction= the BM moves up - As stiffness decreases from base to apex, do does the spread of the TW on the BM - The wave is 2x faster at the base - Greater speed and less dispersive nature of the base (high frequencies) =more synchronous neural discharge=better high frequency ABR response Frequency and Intensity Representation - Stapes input is linear (i.e. 500 Hz, 75 dB sound = 500 vibrations a second with displacement relative to a 75 dB sound) - Upward spread of masking: higher intensity low frequency mask simultaneous high frequency sounds because sounds travel from base to apex - With hearing aids, low frequency gain being too high can cause this - Place of max displacement of the BM is more important that the oscillations of the BM for representing frequency (oscillation rate plays some role in low frequency coding, but refractory period of HC makes it less usable for high frequencies) - TM has a whip-like movement traveling from the area of minimal compliance to maximal compliance (CF)---growing in amplitude as it moves apically, max at the CF, with a rapid decay following (leading to an asymmetrically shaped wave) - Resonance happens when stiffness limitations and mass limitations are equal in magnitude but opposite in phase - Cochlear amplifier: OHC mediated, sharpens frequency tuning, especially at low intensities - OHCs contract on upward BM movement, expanding the downward deflection and therefore increasing amplitude. This happens in a restricted portion of the BM (the area of peak deflections) - Only works for low to mid intensities - Relies on functional metabolic activity - Overall: the BM functions as a mechanical frequency analyzer, distributing energy along its length---the spatial sorting of maximal deflections along the BM lead to frequency selectivity and pitch discrimination. Cochlear non-linearity - If intensity was coded linearly on the BM, damage would occur (ex. BM deflection would have to be 10,000x greater for an 83 dB sound than a 3 dB sound) - So, the BM has a compressor effect for high intensities - Less compression at lower frequencies - Amplification and compression effects precede the coding at the auditory nerve because the number of nerve fibers couldn't code for the range of intensities - At high intensities---non-linear compression, larger area of max deflection, and reduced frequency sensitivity (also, the cochlear amplifier isn't active) - If cochlear amplifier and compression malfunctioned---low intensity sounds may not be heard, frequency discrimination would worsen, and hyperacusis would result Overall: compression=eardrum in=stapes in=BM down=inhibitory response - BM pivot point: foot of the inner pillar cell (near the tip of the bony spiral lamina) - On the ABR: rarefaction clicks results in slightly earlier latencies than condensation because rarefaction is where cells fire (.1-.2msec difference) Endocochlear Potential Stria Vascularis - Role: maintains endolymph ionic composition - Marginal cells: secrete K+ into endolymph - Basal cells: form 2^nd^ electro chemical barrier - Communicate via gap junctions with intermediate cells on the intrastrial side and with fibrocytes (cells that form collagen fibers) on the outer spiral ligament side - Gap junction: electrical, not chemical, synapses are found in collections of fused specialized cells serving the same function; since cells are fused, depolarizing currents flow directly from cell to cell through channel proteins called connexons. Gap junctions are commonly found in smooth muscles like cardiac muscles where rapid and synchronous depolarization is needed  - Four ion transport mechanisms in the stria vascularis - 1\. Selective K+ channel on apical membrane of the marginal cells that releases K+ into endolymph - Membrane potential: 0-10 mV - Connexin 26 deficiency: Mutations in the GJB2 gene causing disruption of potassium recycling in cochlear lymph due to protein deficiency causing loss of endocochlear potential and hearing loss - 2\. Electrogenic Na+/K+/ATPase pump in basolateral membrane of marginal cells. Each cycle, the marginal cells take up to 2 K+ ions and extrude 3 Na+ ions into the intrastrial fluid - 3\. Na+/2Cl-/K+ cotransporter contributes to uptake of 3 K+ ions from the intrastrial space and a Na+ and Cl- - 4\. Cl- channel in basolateral membrane of marginal cells contributes to the flow of Cl- from the marginal cells into the intrastrial space - Because of mechanisms: K+ concentration is low in intrastrial space but high in the intermediate cells - Marginal cells move K+ from the intrastrial space to the endolymph BUT it is the large potential difference between intrastrial fluid and intermediate cells responsible for +80 mV endocochlear potential Hair cell receptor potential - Mechanical vibration is converted to electrical energy through transduction - Transduction channels are near tips of stereocilia - Radial HC shearing opens gated ion channels so endolymph flows into the HCs (depolarization) (high K+ ions) Davis Battery Theory - Stria vascularis produces +80 mV direct current into endocochlear space - At rest: - IHC potential= -45 mV - OHC potential= -70 mV - Total voltage drop: 125-150 mV Excitation/Inhibition - Deflection towards shortest sterocilia= more channels closed = hyperpolarization = decreased neural firing rate - Deflections towards kinocilium = channels open = depolarization = release of neurotransmitter and activation/excitation of nerve fibers De/repolarization - All cells are negatively charged (IHC= -45, OHC= -70) - Depolarization: temporarily make cells' charge more positive - With HCs this is primarily from K+ cations - Repolarization: return cell to its resting potential - With HCs this is primarily from: - 1\. HCs sheared back to resting position, closing K+ channels - 2\. K+ influx will be directed out (efflux) through basolateral HC membrane - Hyperpolarization: make cell slightly more negative - Most cells, the anion Cl-, enters cell to cause inhibition - Overall: influx of K+ depolarizes the HC and opens Ca2+ channels. Influx of Ca2+ activates the Ca2+ activated K+ channels, allowing the outward flow of K+ (and cellular repolarization) Neurotransmitters - IHC are the main sensory cells, and OHC are modulators of transduction through mechanical processes - Afferent IHC transmitter: glutamate - Glutamate transport proteins surround the bases of IHC near type I neural synapses to protect against excess excitotoxic levels of glutamate during over exposure to loud sounds - Efferent IHC transmitters: acetylcholine (primary), dopamine, and GABA - Acoustic overstimulation is associated with neural swelling/inflammation often due to overaccumulation and excitotoxic glutamate around type I nerves---leads to ANSD, high frequency tinnitus, and reduced hearing sensitivity Evoked Cochlear Potentials - Cochlear microphonic - Alternating current with frequency that mimics the stimulus - Vector summation of the individual responses form a large number of hair cells distributed along the basilar membrane - Reflection of receptor currents flowing through the HCs - Predominantly generated by the OHCs - Summating potential - Direct current shift in extracellular response that follows the envelope of the stimulus - Polarity and magnitude effected by stimulus and recording location - Provides better estimate of frequency tuning of the BM than the broad frequency response curve of the CM - Dominant contribution of the IHC (at low to moderate intensities) - Compound action potential - Originates from spiral ganglion neurons that give rise to the auditory nerve response - Onset response that arises from the synchronous response of a group of auditory nerve fibers - Amplitude increases with signal amplitude and latency decreases - CAMP threshold is usually 10-20 dB above the behavioral responses - EcochG - Earliest and smallest electrical response recorded - Main clinical use is diagnosing Meniere's disease (can also be used to distinguish cochlear vs retrocochlear pathology, estimating hearing threshold, and enhancing wave I - SP/AP ratio of 0.4-0.5 is potentially Meniere's - In ANSD---CM should be present and AP absent ECochg Flashcards \| Quizlet Efferent Stimulation - Efferent set of fibers is called the olivocochlear bundle and consists of two systems: MOC and LOC - MOC (crossed): descends from brainstem nuclei of origin to the OHCs - LOC (uncrossed): unmyelinated, descends from brainstem nuclei and innervates the dendrites of type I auditory neurons at the base of IHCs OHC function - 3x more OHC than IHC - 90-95% of type I nerve fibers synapse with IHC, 5-10% synapse with OHC - Type I fibers produce AP response to sounds but type II fibers don't discharge to sounds The Acoustic Nerve Introduction to the AN - Part of the 8^th^ cranial nerve - Courses from the cochlea through the internal auditory meatus - Partially myelinated (proximal to the habenula erforate - Distal IAM is schwann myelin, proximal is olygodendrocite myelin (AKA central myelin) - Auditory bottleneck (slight damage= big consequences) Anatomy of the AN - Start: where cochlear nerve fibers converse in the modiolus (or you could consider the start the HC terminal buttons; note: the terminal buttons are a weak point for damage) - Then, the fibers go through the tunnel of corti and connect with the OHC - Then go thought the habenula perforate (bony structure of the osseus lamina) - Then form the trunk of the modiolus in Rosenthal's canal - Then, go through the IAM (myelinated here) - Then, nerve course through the cerebellopontine angle to the dorsal and posterior ventral CN - End: when AN connects to the cochlear nucleus in the pons - Both efferent and afferent fibers (most afferent) - Two types of AN fibers: - 1\. Type 1 (radial)---90-95%\-- mostly IHC (many fibers to one HC)---myelinated---most fibers in the 2 kHz region - 2. Type II (spiral/longitudinal)---5-10%\-- mostly OHC (many HC share one fiber)---slim to no myelin - High frequencies are on the outside of the AN AN Fibers - Increase from spontaneous firing rate is affected by AN characteristics and dependent on: - 1\. Degree of BM displacement - 2\. Velocity Conditions affecting the AN - Acoustic neuroma - 90% of time, it is a VS - High frequency HL (grow from outside) and tinnitus (encroaches on blood supply) are common symptoms. As are poor speech recognition and elevated/absent ARTs - Can erode the bone of the IAM or grow medially to the CPA - ABR is best for detection: - Increase in interpeak latency between waves I-III or I-V - Since HL is present, wave I may not be visible, so comparing interaural wave V latency could be helpful (wave I is often visible if tumor is medial to its generator. - Auditory neuropathy - Poor ABR, present CM, abnormal ARTs, abnormal MLDs, poor speech recognition, normal OAEs, variable thresholds - Can have similar findings with IHC disorders and low brainstem disorders, so rule those out (sone believe these still fall in the category of ANSD, though) - Hyperbilirubinemia affects CNS nuclei and leads to auditory dyssynchrony AN frequency coding - Characteristic frequency: frequency where AN fibers respond best ie. Have the lowest threshold - Tuning curve: plotted thresholds for various frequencies for one AN - Low frequencies: broader TC - High frequencies: asymmetric TC with steep high frequency side and less steep low frequency side - Damage to OHC: raises threshold of TC and rounds its tip - Frequency is coded two ways: - 1\. Location along the BM of max displacement - 2\. Temporal coding related to firing rate of AN and phase locking ability - Neural discharges phase lock to acoustic stimuli - AN fibers have higher firing rates than rostral structures, so phase locking is most prominent here - Phase locking quickly deteriorates in AN above 1000 Hz - Volley principle: when one fiber reaches its frequency limit, another fiber is recruited so they can alternate, when the second reaches its limit, it recruits another---codes up to 4-5 kHz this way - Two-tone suppression - When two tones are presented, interactions take place; response from the AN fiber to one tone inhibits the response to another tone if it is within the range of frequencies around the AN fiber's response - Intensity and frequency relationship - Low and mid frequencies: sharp tuning - High intensity: broader tuning (less OHC response) - Behaviorally, frequency selectivity is worse at higher intensities - OHC damage or AN fiber damage= worse discrimination (although, speech discrimination effects are variable with acoustic neuroma) AN intensity coding - Spontaneous firing rates vary for AN fibers - Low (0-.5 spikes/second), medium (.5-18), high (\>18) - Higher spontaneous rate fibers have lower thresholds - Lack of auditory input leads to atrophy of nerve fibers and can decrease spontaneous firing rate - High SR fibers have thresholds around 0 dB SPL at the CF and saturate at moderate intensities (around 40 dB SPL) - Low SR fibers have higher thresholds but respond up to 100 dB SPL - Temporary threshold shifts can show a decrease in suprathreshold measures (like ABR wave I) permanently, showing possible damage to low SR nerve fibers aka "hidden hearing loss"---side of lesion is likely at CN terminals (synaptic ribbons) and AN synaptic region - Firing pattern and adaptation - AN have initial spike with adaptation and plateau - Adaptation: decrease in AN action potential over time without change in stimulus - Greater adaptation for high frequency sounds - Greater adaptation for those with \>50 dB HL hearing loss - Adaptation is neural activity not a sensory response (hence why CM doesn't change) AN evoked potentials - Near-field responses are used for AN monitoring in surgery - AP (wave I) and ABR wave II are both from the AN - Wave I latency decreases by 0.3-0.4 msec at higher intensities due to traveling wave mechanics The Cochlear Nucleus Introduction to the CN - 3 major subdivisions: dorsal (DCN), posterior ventral (PVCN), and anterior ventral (AVCN) - Located in the pontomedullary junction - The CN modifies and repackages information (AN fibers have one firing patter, but the CN has multiple firing patterns due to diverse cell types) - The CN contributes to the ABR wave III - Hydrocephalus in the fourth ventricle can compress the CN and can affect hearing function and ABR results Inputs - AN enters at the root entry zone then bifurcates - Sends to the AVCN and PVCN (then arrives at the DCN through the PVCN) CN intensity coding - Most fibers have a DR for intensity of 30-40 dB (some have 80-90 dB DR) - CN alters firing rates in a stepwise manner by small amounts in response to a continuous tone that decreased or increases in intensity---it has great sensitivity for coding intensity - Very sensitive to AM tones - Better pediatric sound field responses to warble tones in partially due to standing wave avoidance and partially because the CANS neurons respond better to modulated tones - CN fibers follow the envelope of modulation with fluctuating firing rates up to 60-80 dB range (with only a 20-30 dB intensity DR for steady-state stimuli - AM frequency that CN responds to vary, but best at 300 Hz (optimal modulation rate decreases as you move up the CANS) - ASSR uses modulation rates below 100 HZ CN frequency coding - TC of the CN very from the AN because of inhibitory influences that narrow the cochlear nucleus TCs to increase frequency specificity to affect the representation of complex signals. Temporal functions of CN - In animal studies, phase locking could happen for inputs up to 3-4 kHz - Bushy and octopus cells have the best synchronicity because of abrupt on responses---these cells, therefore, play a key role in tests like ABR and gap detection because of the requirement of quick analysis Outputs - CN projects to the contralateral and ipsilateral SOC, ventral nucleus of the LL, and the IC Pathologies associated with the CN - Intrinsic lesions to the lower pons, midline: bilateral ABR and behavioral deficits - CN lesions: ipsilateral effect behavioral and electrophysiological - Hyperbilirubinemia/kernicterus: damage to nuclei of the brain, especially to the CN (and other brainstem nuclei) - High frequency SNHL (often with normal OAEs) - May see I-III interpeak latency increase - Often, ANSD associations are made due to similar profiles, but jaundice primarily effects brain tissue (may have some secondary association with the auditory nerve) - Noise exposure: may result in DCN hyperactivity - Tinnitus: may be result from DCN hyperactivity Additional CN associations - The DCN is the site of auditory brainstem implant placement - The acoustic startle response travels to the CN before evoking a motor response - ME---cochlea---AN---CN---pons and pontine reticular formation---spinal cord neurons---muscles of the upper torso The Superior Olivary Complex Anatomy - Main nuclei: lateral superior olive (LSO), medial superior olive (MSO), and trapezoid body - SOC contributes to ABR wave IV - Narrow tuning curves with good sensitivity. Strong phase-locking ability (up to 2-3 kHz) - Inputs: most input comes from the contralateral CN - Outputs: - LSO: - lateral LSO (low frequencies) to ipsilateral IC (inhibitory) - medial LSO (high frequencies) to contralateral IC (excitatory) - MSO: ipsilateral IC Physiology - First site of binaural hearing---fuses information for it to later be processed and made meaningful - Analyzes differences in neural impulse timing and intensity for recreation of the auditory spatial field - Sensitive to ILDs and ITDs for localization - Localization/lateralization improves hearing speech and noise (cocktail party effect) by helping listener focus on the signal, employing auditory filtering and suppression ability because of the spatial origin of the signal and noise Neuron responses - EE, EI, IE, EO, or OE (contralateral, ipsilateral) - MSO: 65% EE, 25% EI, 10% EO or OE - Highly sensitive to ITDs (better at low frequencies) - LSO: mostly IE - Highly sensitive to ILDs (better for high frequencies) SOC pathologies - Fourth ventricle damage: secondary effect on SOC - Interaxial (low pons) lesions: affect SOC and can be seen through ABR, MLD, and ART abnormalities Masking level differences - Looks at ability to process ITDs (MSO function) - Protocol: - 1\. Present signal and noise to both ears (N0S0) - 2\. Create phase difference in signal between ears (N0SÏ€) - Improvement in thresholds (up to 15 dB better for low frequencies and as low as a 3 dB improvement for high frequencies) - Why? High frequency phase difference is small in reference to time and too brief for neurons to respond accurately - 3\. Many combinations exist (ex. NuS0---uncorrelated noise conditions---is up to a 13 dB improvement) - MLDs are reduced for those with pontomedullary lesions (but not for those with higher CANS lesions) Binaural interaction components (BIC) of ABR - Wave (IV on) amplitudes: monaural\