A Technology Enabler for Green Machining: Minimum Quantity Lubrication (MQL) PDF
Document Details
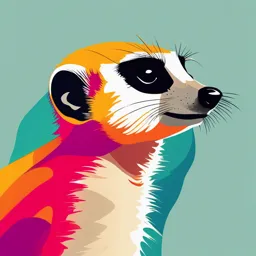
Uploaded by FriendlyOrphism
McMaster University
Nourredine Boubekri, Vasim Shaikh and Phillip R. Foster
Tags
Related
- Minimum Staffing Levels PDF - Chesapeake Fire Department Policy
- OMB Chapter 8 Ed 3 Rev 33 - Operations Manual PDF
- Li et al. - 2024 - Minimum Quantity Lubrication (MQL) Review PDF
- Evaluation of Cryogenic Machining of Inconel 718 (PDF)
- The Effects of Minimum Quantity Lubrication (MQL) on Machining Force, Temperature, and Residual Stress PDF
- Government Regulations
Summary
This paper reviews the literature on minimum quantity lubrication (MQL), focusing on its economic, environmental, and technical advantages compared to conventional flood-cooling techniques. It also highlights areas for future research in this machining technology. The paper is a valuable resource for stakeholders interested in using or designing machining systems using microlubrication.
Full Transcript
The current issue and full text archive of this journal is available at www.emeraldinsight.com/1741-038X.htm JMTM 21,5 A technology enabler for green machining: minimum qua...
The current issue and full text archive of this journal is available at www.emeraldinsight.com/1741-038X.htm JMTM 21,5 A technology enabler for green machining: minimum quantity lubrication (MQL) 556 Nourredine Boubekri, Vasim Shaikh and Phillip R. Foster Department of Engineering Technology, University of North Texas, Received December 2008 Revised November 2009 Denton, Texas, USA Accepted January 2010 Abstract Purpose – The purpose of this paper is twofold. First review the relevant literature in machining using minimum quantity lubrication (MQL), contrast the economical, environmental, and technical attributes of this technology to conventional flood-cooling techniques. Second highlight areas of relevant future research. Design/methodology/approach – The approach consists of describing the essential elements of MQL as a technology, reviewing the relevant research by focusing on the most frequently used machining processes in this industry, highlighting the findings as they compare to flood cooling, and pointing to directions of required research in this technology. Findings – The application of MQL in machining has shown better results in some processes; including in drilling, a cleaner environment, and a more cost-effective machining technology. Further research is required however to better understand the underlying cause an effect phenomena in machining using microlubrication technology including environmental and health effects of this technology. Practical implications – The paper provides a body of knowledge required for all stakeholders to better use or design machining systems using microlubrications. Originality/value – This paper focuses on the state of the art of MQL and how it contrasts with conventional methods of machining. Keywords Lubrication, Lubricating systems, Cooling systems Paper type Technical paper Introduction Shortly after the Industrial Revolution in America, Frederick Winslow Taylor flooded the tool-work interface with a heavy stream of water and discovered that the cutting speed could be increased by a factor of 2 or 3. This initial application of a “cutting fluid” inspired today’s impressive variety of extremely effective fluids. These fluids provide numerous advantages which include: mechanical and chemical lubrication thereby reducing friction; cooling of the work and tool; enhancing dimensional stability; inhibiting chip welding which further stabilizes dimensions; and, flushing away chips which improves surface finish, tool efficiency and makes automated material handling practical. Unfortunately, these fluids also share several negative characteristics. Many are costly to purchase; all are applied in volume; all must be periodically replaced; and, when improperly used and disposed off can lead to health and environmental issues. It is these negative characteristics that have prompted researchers to investigate alternative solutions to Journal of Manufacturing Technology traditional cutting fluids and their method of application (flood cooling). Management Vol. 21 No. 5, 2010 pp. 556-566 Background information q Emerald Group Publishing Limited 1741-038X The current trend in the metal-cutting industry is to find ways to reduce cutting fluid DOI 10.1108/17410381011046968 use; the use of coolants in machining is thought to be undesirable for economical, health, and environmental reasons. Heins (1997) reported that coolant and coolant management Minimum costs are between 7.5 and 17 percent of the total manufacturing cost compared to only quantity 4 percent for cutting tools. Ngoi and Sreejith (2000) stated that lubrication represents 16-20 percent of the product cost. Quaile (2000) reported that the coolant cost represents lubrication about 15 percent of the life-cycle operational cost of a machining process. According to a survey conducted by the European Automobile Industry, the cost incurred on lubricants comprises nearly 20 percent of the total manufacturing cost contrasted with the cost of 557 the cutting tool which is only 7.5 percent of the total cost (Brockhoff and Walter, 1998). Chalmers (1999) reported that more than 100 million gallons of metalworking fluids are used in the USA each year and that 1.2 million employees are exposed to them and to their potential health hazards. The US Occupational Safety and Health Administration (Aronson, 1995) and the US National Institute for Occupational Safety and Health (NIOSH) reported that the permissible exposure level for metal working fluid (MWF) aerosol concentration is 5 and 0.5 mg/m3, respectively, (US Department of Health and Human Services, 1998). However, the oil mist level in the US automotive parts manufacturing facilities has been estimated to be 20-90 mg/m3 with the use of conventional lubrication by flood coolant (Bennett and Bennett, 1985). The exposure to such amounts of MWF may contribute to adverse health effects and safety issues, including toxicity, dermatitis, respiratory disorders, and cancer. The mechanical infrastructure that sustains a flood coolant system is of such complexity that it hinders the rapid reconfiguration of equipment. In the conventional application of flood coolant, the chips produced are wet. They have to be dried before recycling, which incurs additional cost. Minimum quantity lubrication (MQL), on the other hand, produces essentially dry chips, so the cost of drying them is reduced (Filipovic and Stephenson, 2006). The savings in cutting fluid and related costs could be significant if MQL was adopted. Minimum quantity lubrication The concept of MQL was suggested a decade ago as a mean for addressing the issues of environmental intrusiveness and occupational hazard associated with airborne cutting fluid particles. The minimization of cutting fluid leads to economical benefits by saving lubricant costs. Workpiece, tool, and machine-cleaning time are reduced. The MQL technique consists of misting or atomizing a very small quantity of lubricant, typically of a flow rate of 50 to 500 ml/h, in an air flow directed towards the cutting zone (Autret and Liang, 2003). The lubricant is sprayed by means of an external supply system consisting of one or more nozzles. The amount of coolant used in MQL is about 3-4 orders of magnitude lower than the amount commonly used in flood-cooling condition. MQL, also known as “Microlubrication” (MaClure et al., 2007) and “Near-Dry Machining” (Klocke and Eisenblatter, 1997), is the latest technique of delivering metal cutting fluid to the tool/work interface. Using this technology, a little fluid, when properly selected and applied, can make a substantial difference in how effectively a tool performs. In conventional operations utilizing flood coolant, cutting fluids are selected mainly on the basis of their contributions to cutting performance. In MQL, however, secondary characteristics are important. These include their safety properties (environment pollution and human contact), biodegradability, oxidation, and storage stability. This is important because the lubricant must be compatible with the environment and resistant to long-term usage caused by low consumption (Wakabayashi et al., 2006). In MQL, lubrication is obtained via the lubricant, while a minimum cooling action is achieved by JMTM the pressurized air that reaches the tool/work interface. Further, MQL reduces induced 21,5 thermal shock and helps to increase the workpiece surface integrity in situations of high tool pressure (Attanasio et al., 2006). Types of MQL systems There are two basic types of MQL delivery systems: external spray and through-tool. 558 The external spray system consists of a coolant tank or reservoir which is connected with tubes fitted with one or more nozzles. The system can be assembled near or on the machine and has independently adjustable air and coolant flow for balancing coolant delivery. It is inexpensive, portable, and suited for almost all machining operations. Through-tool MQL systems are available in two configurations; based on the method of creating the air-oil mist. The first is the external mixing or one-channel system. Here, the oil and air are mixed externally, and piped through the spindle and tool to the cutting zone. The advantages of such systems are simplicity and low cost; they are suited to be retrofitted to existing machines with high pressure, through the tool-coolant capability. They are easy to service; no critical parts are located inside the spindle. The disadvantage is that the oil-mist is subjected to dispersion and separation during its travel from the nozzle. To minimize oil drop outs, a mist of relatively fine particles is used, which often limits the amount of lubrication that can be supplied to the cutting zone and consequently affects the performance of the cutting process. The second configuration is the internal mixing or two-channel systems. Most commonly in a two channel system, two parallel tubes are routed through the spindle to bring oil and air to an external mixing device near the tool holder where the mist is created. This approach requires a specially designed spindle. Such systems have less dispersion and dropouts and can deliver mist with larger droplet sizes than external mixing devices. They also have less lag time when changing tools between cuts or oil delivery rate during a cut. However, the systems are more difficult to maintain; critical parts are located inside the spindle (Filipovic and Stephenson, 2006). MQL lubricant characteristics Lubricant concentration in MQL varies between 0.2 and 500 ml/h. Since very good lubrication properties are required in MQL, vegetable oil or synthetic ester oil are used instead of mineral oil. Air pressure is roughly five bars (Filipovic and Stephenson, 2006). MQL is consumption lubrication, that is, the bulk of the lubricant applied is evaporated at the point of application. This evaporation, in concert with the compressed air stream, cools the workpiece. The remaining heat is dissipated through the tool and the chips (SCHUNK, 2007). The chips, workpiece and tool remain nearly dry in an ideally adjusted MQL system. Wakabayashi et al. (2006) introduced synthetic polyol esters and described their capabilities as MQL fluids. These represent a potential replacement for vegetable-based MQL oils, particularly with regard to their optimal secondary performance characteristics. All vegetable oils display high biodegradability. Synthetic esters, however, provide a wide range of biodegradability depending on their combined molecular structures of acids and alcohols. This characteristic, in conjunction with their suitable viscosities, prompted (Wakabayashi et al., 2006) to identify these lubricants for further examination. Physical properties and biodegradability of polycol esters were compared with a vegetable oil. The viscosity, total acid number (TAN), pour point and biodegradability for polycol ester oil were 19.1 mm2/s, 0.02 mgKOH/g, 458C and 100 percent, respectively. Minimum These characteristics for vegetable oil were 35.6 mm2/s, 0.04 mgKOH/g, 208C and quantity 98 percent, respectively. The molecular weights of polycol ester oil and vegetable oil were also compared. The molecular weight of the oil film increased by more than lubrication 10 percent. The molecular weight of vegetable oil increased by 65 percent. In contrast, there was no significant change in the molecular weights of polyol esters. Most vegetable oils consist of a number of ester compounds mainly derived from a combination of 559 glycerin and fatty acids. Vegetable oils are usually liquids at room temperature, due to their unsaturated bonds. Unfortunately, unsaturated bonds are chemically unstable and may cause vegetable oils molecular weight to increase. A detailed investigation of this behavior was carried out using gel permeation chromatography analysis. The results indicated that some of the molecules in vegetable oil had changed into compounds having higher molecular weights. Results of the UV analysis, which can selectively detect changes in unsaturated double bonds, indicate the unsaturated structure decreased significantly. This result supports the hypothesis that: H1. The unsaturated bond structure of vegetable oil molecules is the main cause of their easy degradation by oxidation polymerization. The polyol esters chosen as preferable biodegradable lubricants in this investigation are synthesized from a specific polyhydric alcohol rather than glycerin. Their molecules can greatly improve oxidation stability; they are free from unsaturated bonds. Regardless, they can be liquid at room temperature. Compared with vegetable oils, the synthetic polyol esters studied were optimal lubricants for MQL machining from the standpoint of maintaining a clean working environment. Another characteristic studied concerned the long-term storage potential of polyol esters and vegetable oils. Lubricant containers are often stored outside, and the temperature in the containers can rise as high as 708C. Since an MQL system consumes very little lubricant, the lubricant must remain stable under such conditions. In order to simulate this storage situation, an oxidation test was conducted at 708C for four weeks. Changes in viscosity and TAN were measured. The change in viscosity for polyol ester oil and vegetable oil after the storage stability test were 0.01 and 1.5 percent and the change in TAN were 0.01 and 0.18 percent, respectively. While the viscosity and TAN of polyol ester were almost constant, the values for vegetable oil increased considerably. These results confirm the stability of the molecular structure of the synthetic esters regarding oxidative degradation, thus promoting their stability in storage. Selected recent research on MQL Recent MQL researches tend to emphasize drilling operations. Other process reported include: turning, milling, and grinding. Work piece materials reported include medium carbon steels, 4100 and 4300 series alloy steels, aluminum, nodular cast iron, and titanium. Various cutting tool materials, ranging from the common to the exotic, have been studied. Included are: coated and uncoated high-speed steel (HSS) and cobalt HSS; coated and uncoated carbide; aluminum oxide (Al2O3); cubic boron nitrite (CBN); and, polycrystalline diamond. The following studies are representative of research conducted in the area. Each highlights one or more contributions of this emerging technology to the field of manufacturing technology. JMTM MQL applied to drilling operations 21,5 MQL performance using coated and uncoated HSS and cobalt HSS drills, in a high-aspect ratio operation, was examined by Heinemann et al. (2006). The workpiece material was AISI 1045. The workpiece was mounted using a two component dynamometer which measured thrust force and torque. The twist drills tested had a diameter of 1.5 mm and an included angle of 1308. Drills were of uncoated HSS, uncoated cobalt HSS, and cobalt HSS with 560 various coatings. A cutting speed of 26 m/min and a feed rate of 0.26 mm/rev were used. Three series of tests were performed. MQL-supply in the first series of tests was stopped once the drill reached a depth of 5 mm. In the second series of tests, two other lubricants were used; one with the same chemical composition as the lubricant used in the first series but without alcohol, and one composed of an oil-free synthetic lubricant with a water content of 40 percent. In the third test series, drilling was carried out under dry conditions. In the first series of tests, it was observed that interruption of the MQL-supply caused a dramatic drop of 98 percent in tool life for the uncoated cobalt HSS drills. In the case of the TiN and TiAlN-coated twist drills, the tool life also decreased, but by 42 and 27 percent, respectively. The second series of tests, carried out with three different types of MQL, had the lubricant supplied continuously at a rate of 18 ml/h. All tests were performed with uncoated HSS drills. The alcohol-free lubricant resulted in an increase of 23 percent in tool life over that achieved with the alcohol-blended lubricant. When using the oil-free synthetic lubricant plus 40 percent water, the tool life increased by a 100 percent. The study reported that a continuous MQL supply is beneficial in terms of tool life, whereas interrupting the MQL supply leads to a significant drop in tool life, especially in the case of heat-sensitive drills. With respect to the type of MQL lubricant, a low viscous type with high-cooling capability gives rise to a notably prolonged tool life. A similar study (Dosbaeva et al., 2008) was conducted to compare MQL and wet drilling using tooling prepared with thin perfluoropolyether (PFPE) lubricant films. The main findings indicate that the PFPE surface treatment reduced the cutting torque, increased tool life and improved the surface finish of the machined part. Also, drilling with pecking showed some improvement with the number of holes produced being more consistent. Zeilman and Weingaertner (2006) also reported on MQL and drilling operations. The workpiece material investigated was titanium alloy Ti6Al4V (300 BHN). They analyzed the temperature during drilling while using class K10 carbide drills with and without hard coating (TiAlN, CrCN or TiCN). Cutting fluids were applied with a pressure of 3.5 bar. Two types of drills (Types 125 and 105) were used in these experiments. All were carbide containing 9.5 percent cobalt, had a diameter of 8.5 mm and had three edges. The Type 125 drill had an internal cooling channel and the Type 105 drill utilized an external nozzle. To verify workpiece temperature at particular depths, special plates were made for insertion of a type K thermocouple. Cutting speeds of 10-50 m/min and feeds of 0.1-0.2 mm were used. It was concluded that the measured temperature with application of MQL internally through the tool was 50 percent lower than that obtained with MQL applied with an external nozzle. Braga et al. (2002) conducted MQL experiments using diamond-coated and uncoated carbide drills. Drills used in the experiments were made of uncoated ISO K10 carbide and diamond-coated carbide. The K10 carbide drills had an average diameter of 9.986 mm Minimum and the diamond drills had an average diameter of 9.992 mm. The workpieces were quantity aluminum-silicon alloy with 7 percent silicon (SAE 323). In this experiment, two cooling systems were used. The first was a mixture of air and oil (MQL). Of mineral oil, 10 ml/h lubrication was introduced into the air flow of 72 m3/h and 4.5 bar of pressure. The second system was a flood of soluble oil (one part oil to 25 parts water) with a flow rate of 2.4 m3/h. For both systems, a consistent cutting speed of 300 m/min and feed of 0.1 mm/rev was used. 561 The study reported that MQL performance, in terms of forces, tool wear and quality of holes, was similar to that obtained when using large amounts of soluble oil, with both, coated and uncoated K10 drills. It was observed that the value of flank wear was similar when using coated or uncoated K10 drills. Power consumption for the two drill materials, when using MQL was similar at 0.81 Kw (coated) and 0.79 Kw (uncoated) at 20-m feed lengths. Feed force displayed nearly the same rate of increase with feed length for all experiments regardless of the cutting condition and tool material. The uncoated K10 drill presented the best results related to the average diameter of the hole. For the diamond-coated drill, results are better when MQL was used. For uncoated drills, the results were similar for both cooling systems. These conclusions support the potential for using MQL in drilling aluminum-silicon alloys. A similar study (Tasdelen et al., 2008a, b) was conducted to compare MQL at different flow rates with emulsion and compressed air cooling in the drilling of gear wheel steel. The main findings indicate that the highest wear was observed for emulsion, followed by air and MQL assisted machining. In terms of surface finish, MQL (15 ml/h) and emulsion drilling gave the best result followed by air, MQL (5 ml/h) and MQL (23 ml/h). MQL applied to drilling and milling operations In May 2007, an article was published by Tech Solve, based on a comparison between flood and MQL (MaClure et al., 2007). The lubricant used was experimental vegetable oil-based soluble oil (10 percent). The flow rates used for flood and mist conditions were 1.7 and 0.0029 gpm, respectively. Experiments were conducted for drilling and milling operations. The drilling operation used AISI 4340 Steel (32-34 HRC). The speed and feed rate levels were 55 sfpm and 0.007 ipr, respectively. The drill used was 0.5-in. oxide-coated HSS with a 1358 split point. Using flood coolant, 60 holes were drilled and utilizing MQL 61 were drilled. Analysis showed no significant differences in tool life (number of holes to reach end of life criteria) between MQL and flood cooling. Average thrust forces were 570 and 447 lbs for flood and MQL cooling, respectively. The milling operation used AISI 4140 Steel (24-26 HRC). The speed, feed rate and depth of cut levels were 400 sfpm, 0.005 ipr and 0.5 in., respectively. The cutter insert was grade SM-30 uncoated carbide. The analysis showed little differences in tool life between flood and MQL cooling. For the flood tests, 66 passes were milled and 80 were milled for the MQL tests. The average resultant forces observed were 46 lbs for flood and 36 lbs for MQL cooling (MaClure et al., 2007). A similar study (Furness et al., 2006) was conducted to show the technological developments and implementation of MQL at the Ford Motor Company. The main findings indicate that a ten year per machine life cycle comparison with flood cooling showed a 15 percent reduction of operating cost with the implementation of MQL. It was also observed that for deep hole drilling, the feed rate could be increased from JMTM 125 mm/min for wet application to over 660 mm/min with MQL. MQL had a positive 21,5 effect on tool life, and reduced cycle time. Operator exposure to MWFs for skin conditions, inhalation, and slip and fall have all been reduced due to MQL. MQL applied to turning operations A study involving the intermittent turning of aluminum alloy on a CNC lathe was 562 undertaken by (Itoigawa et al., 2006). There were two test conditions. The first had a cutting speed of 200 m/min, feed rate of 0.05 mm/rev and axial travelling length of 3 mm. The second condition had a cutting speed of 800 m/min, feed rate of 0.2 mm/rev and axial travelling length of 10 mm. In both, the MQL oil supply rate was fixed at 30 ml/h and air flow rate at 70 l/min. For MQL with water droplets, tap water was used at a rate of 3,000 ml/h. Rapeseed oil and synthetic esters (mono carboxylic acid with polyalcohol) were employed as lubricants. Cutting tests using emulsion type coolant and dry machining were performed in the same conditions. Two tools were used; a sintered diamond tool with 08 rake angle and a K10 grade carbide tool with 58 rake angle. MQL with rapeseed oil had a small lubricating effect in light loaded machining conditions. Results showed MQL with water droplets, specifically an oil film on a water droplet, provided good lubrication performance if an appropriate lubricant, such as synthetic ester, was used. When MQL with synthetic ester but without water was used, it showed a lubrication effect. However, tool damage was evident as was chip welding (Itoigawa et al., 2006). A similar study (Li and Liang, 2007) was conducted to develop the understanding of mechanical and environmental effects of MQL in machining and characterize MQL performance as a function of machining and fluid application parameters. The main findings indicate that MQL reduced the tangential cutting force, at low cutting speeds. MQL showed a significant reduction in the cutting temperature over a wide range of speeds, and resulted in lower cutting tool wear rate as compared to dry machining. Khan and Dhar (2006) conducted an experiment to evaluate the performance of MQL by vegetable oil in terms of cutting force, cutting zone temperature, tool wear, job dimension, and surface finish in turning AISI-1060 steel. The main findings indicate that the cutting force, cutting zone temperature, tool wear, and surface roughness were reduced by the application of MQL as compared to dry cutting. Application of MQL also improved the dimensional accuracy as compared to dry cutting. A similar study (Dhar et al., 2006) was conducted to experimentally investigate the role of MQL on tool wear and surface roughness in turning AISI-4340 steel by uncoated carbide inserts. The results were compared to those of dry and wet machining. The main findings indicate that the tool wear and surface roughness were reduced by the application of MQL as compared to dry and wet cutting. The study indicated that MQL cutting was better than dry and flood cutting because MQL provided lower cutting temperatures, which improved the chip-tool interaction and maintained sharpness of the cutting edges. Autret and Liang (2003) conducted a study where they compared the mechanical performance of MQL with completely dry lubrication when turning hardened, bearing-grade steel using CBN cutters. The process attributes analyzed included: surface roughness, cutting temperature, cutting forces, and tool life. A range of feeds from 0.002 to 0.014 ipr were employed. The cutting speed was 450 sfpm and the depth of cut was 0.012 in. The fluid tested was a triglyceride and propylene glycol ester solution vegetable-based cutting fluid at a flow rate of 50 ml/h at a nozzle pressure of 20 psi. The findings indicated that regarding surface roughness, no noticeable difference Minimum was found with the use of MQL over dry turning. An improvement of surface finish was quantity however indicated by MQL machining under higher depths of cut (0.012 in.) and feeds (0.006 ipr). In the context of a steady-state cutting temperature, a 10-30 percent reduction lubrication was consistently observed when MQL was applied as opposed to dry turning. The study reported that this result was due to an increase in the evaporative heat transfer at the cutting zone. Regarding cutting forces, there were no significant differences observed 563 with the use of MQL or dry cutting. The cutting force was approximately 250 N at a feed of 0.012 in./rev. The study on the other hand showed a significant increase in tool life of 35-50 percent when using MQL as compared to dry turning over a wide range of cutting conditions. Similarly, Dhar et al. (2007) conducted a study to investigate the role of MQL on cutting temperature, chip formation mode, cutting forces, and tool wear as compared to dry machining. The material used was AISI 1040 steel. The main findings indicate that MQL reduced the cutting temperature, cutting force, flank wear, and surface roughness more than dry cutting. MQL also increased the tool life and provided greater dimensional accuracy. A similar study (Tasdelen et al., 2008a, b) was conducted to investigate the influence of MQL, compressed air and emulsion assisted cutting on tool-chip contact length. The main findings indicate that the contact length was the same for MQL and compressed air assisted cutting but emulsion-assisted cutting gave the shortest contact length. MQL applied to milling operations Recently, in June 2009, a study (Heisel et al., 2009) was conducted to investigate the influence of burr formation using MQL in up-, down- and face-milling. The main findings indicate that variation in cutting speed showed no influence on burr formation. But, varying feed per tooth increased the burr value in dry machining and MQL. The supply of the fluid through an external nozzle proved to be disadvantageous. A similar study (Kang et al., 2008) was conducted to investigate the effect of the MQL in high-speed end-milling of AISI D2 cold worked die steel. The tool performances of Ti0.75Al0.25N and Ti0.69Al0.23Si0.08N-coated carbides end-mills were compared using wet, dry and MQL conditions. The main findings indicate that MQL conditions showed maximum cutting length with minimum flank wear followed by dry cutting and wet cutting. Ti0.69Al0.23Si0.08N coating was better than Ti0.75Al0.25N coating. Liao and Lin (2007) conducted an experiment to investigate the mechanism of MQL in high-speed milling of hardened steel and compared with dry cutting. The main findings indicate that cutting force and surface roughness under MQL was less than dry cutting. Tool performance under MQL was enhanced under all cutting speeds. MQL applied to grinding operations Da Silva et al. (2007) studied the effectiveness of MQL in grinding operations. The workpiece material was tempered and annealed ABNT 4340 steel (HRC 60). Tests utilized Al2O3 grinding wheels (FE 38A60KV). The main parameters included a grinding wheel speed of 30 m/s, an in-feed rate of 1 mm/min, a workpiece speed of 20 m/min, a depth of cut of 0.1 mm and a spark-out time of 10 s. These were held constant throughout the tests. A synthetic emulsion in a 5 percent concentration was applied for both conventional flood cooling and MQL. JMTM No significant clogging of the grinding wheel pores was found with MQL. The use of 21,5 MQL did not negatively affect surface integrity; roughness values were decreased due to the excellent properties of lubricity using MQL. No significant subsurface alterations in the microstructure were detected under conventional cooling or with MQL. A similar study (Li et al., 2008) was conducted to analyze the feasibility of using MQL as an environmentally corrective alternative to the grinding fluid utilized in 564 surface grinding compared with conventional cooling. The main findings indicate that MQL leads to lower surface roughness and heat affected zone and higher micro hardness as compared to flood cooling. MQL was found to be a better alternative to flood cooling because it combined the functionality of cooling with an extremely low consumption of lubricant. Shen et al. (2008) conducted a study to investigate the grinding wheel wear and tribological characteristics in wet, dry and MQL grinding of cast iron. The main findings indicate that MQL grinding reduced the grinding temperature more as compared to dry grinding. It was also observed that increase in MQL flow decreased the grinding temperature. Conclusions and future research Findings from the forgoing research can be summarized under the categories of: “Air quality”; “Capability and robustness” and “Cost”. Air quality MQL applications generate mist. This later must be effectively controlled to realize the benefits of MQL. Mist collection or filtering equipments are generally required to manage this fine mist particularly in ferrous machining, where sparking and smoking is often observed. Capability and robustness MQL has been shown to work well in short-term tests over a range of processes. Long-term capability and robustness remain still unanswered. These issues may be sorted out when more extensive MQL experience is accumulated from large-scale production applications. More material specific issues may require additional testing. For example, aluminum machining includes sensitivity to surface finish due to a tendency of the material to create a built-up edge on the tooling. Many high-tensile materials, e.g. medium carbon and alloy steels, are also subject to this condition. Variations in tool geometry and/or coating conditions may provide answers to these issues. The processes of lubrication and cooling in MQL are yet to be well understood. The process of MWFs mist particles generation and their physical characteristics are yet to be determined for a whole class of machining processes and machining conditions. Cost MQL production experience to date has indicated favorable cost-reduction applications due to the reduced cost of managing the cutting fluids. This observation is expected to be more relevant as MQL is used in mass production. References Aronson, R.B. (1995), “Why dry machining”, Manufacturing Engineering, Vol. 114 No. 1, pp. 33-6. Attanasio, A., Gelfi, M., Giardini, C. and Remino, C. (2006), “Minimum quantity lubrication in turning”, Wear, Vol. 260, pp. 333-8. Autret, R. and Liang, S.Y. (2003), “Minimum quantity lubrication in finish hard turning”, Minimum Proceedings of International Conference on Humanoid, Nanotechnology, Information Technology, Communication and Control, Environment, and Management, Manila [CD]. quantity Bennett, E.O. and Bennett, D.L. et al., (1985), “Occupational airways diseases in the metal lubrication working industries”, Tribology International, Vol. 18 No. 3, pp. 169-76. Braga, D.U., Diniz, A.E., Miranda, G.W.A. and Coppini, N.L. (2002), “Using a minimum quantity of lubricant (MQL) and a diamond coated tool in the drilling of aluminum-silicon alloys”, 565 Journal of Materials Processing and Technology, Vol. 122, pp. 127-38. Brockhoff, T. and Walter, A. (1998), “Fluid minimization in cutting and grinding”, Abrasives, October, pp. 38-92. Chalmers, R.E. (1999), “Global flavor highlights NAMRC XXVII”, Manufacturing Engineering, Vol. 123 No. 1, pp. 80-8. Da Silva, L.R., Bianchi, E.C., Fusse, R.Y., Catai, R.E., Franca, T.V. and Aguiar, P.R. (2007), “Analysis of surface integrity for minimum quantity lubricant-MQL in grinding”, International Journal of Machine Tool and Manufacture, Vol. 47, pp. 412-8. Dhar, N.R., Ahmed, M. and Islam, S. (2007), “An experimental investigation on effect of minimum quantity lubrication in machining AISI 1040 steel”, International Journal of Machine Tools & Manufacture, Vol. 47 No. 5, pp. 748-53. Dhar, N.R., Kamruzzaman, M. and Ahmed, M. (2006), “Effect of minimum quantity lubrication (MQL) on tool wear and surface roughness in turning AISI-4340 steel”, Journal of Materials Processing Technology, Vol. 172 No. 2, pp. 299-304. Dosbaeva, J., Fox-Rabinovich, G., Dasch, J. and Veldhuis, S. (2008), “Enhancement of wet- and MQL-based machining of automotive alloys using cutting tools with DLC/polymer surface treatments”, Journal of Materials Engineering and Performance, Vol. 17 No. 3, pp. 346-51. Filipovic, A. and Stephenson, D.A. (2006), “Minimum quantity lubrication (MQL): applications in automotive power-train machining”, Machining Science and Technology, Vol. 10, pp. 3-22. Furness, R., Stoll, A., Nordstrom, G., Martini, G., Johnson, J., Loch, T. and Klosinski, R. (2006), “Minimum quantity lubrication (MQL) machining for complex powertrain components”, Proceedings of the International Conference on Manufacturing Science and Engineering, Psilanti, MI. Heinemann, R., Hinduja, S., Barrow, G. and Petuelli, G. (2006), “Effect of MQL on the tool life of small twist drills in deep-hole drilling”, International Journal of Machine Tool and Manufacture, Vol. 46, pp. 1-6. Heins, H.J. (1997), “Dry machining – a promising option”, American Machinist, Vol. 126 No. 8, pp. 92-3. Heisel, U., Schaal, M. and Wolf, G. (2009), “Burr formation in short hole drilling with minimum quantity lubrication”, Production Engineering, Vol. 3 No. 2, pp. 157-63. Itoigawa, F., Childs, T.H.C., Nakamura, T. and Belluco, W. (2006), “Effects and mechanisms in minimal quantity lubrication machining of an aluminum alloy”, Wear, Vol. 260, pp. 339-44. Kang, M., Kim, K., Shin, S., Jang, S., Park, J. and Kim, C. (2008), “Effect of the minimum quantity lubrication in high-speed end-milling of AISI D2 cold-worked die steel (62 HRC) by coated carbide tools”, Surface and Coatings Technology, Vol. 202 Nos 22/23, pp. 5621-4. Khan, M.M.A. and Dhar, N.R. (2006), “Performance evaluation of minimum quantity lubrication by vegetable oil in terms of cutting force, cutting zone temperature, tool wear, job dimension and surface finish in turning AISI-1060 steel”, Journal of Zhejiang University (Science), Vol. 7 No. 11, pp. 1790-9. Klocke, F. and Eisenblatter, G. (1997), “Dry cutting”, Annals of the CIRP, Vol. 46 No. 2, pp. 519-26. JMTM Li, C.H., Hou, Y.L., Xiu, S.C. and Cai, G.Q. (2008), “Application of lubrication theory to near-dry green grinding – feasibility analysis”, Advanced Materials Research, Vol. 44-46, pp. 135-42. 21,5 Li, K. and Liang, S.Y. (2007), “Performance profiling of minimum quantity lubrication in machining”, International Journal of Advanced Manufacturing Technology, Vol. 35 Nos 3/4, pp. 226-33. Liao, Y.S. and Lin, H.M. (2007), “Mechanism of minimum quantity lubrication in high-speed milling of hardened steel”, International Journal of Machine Tools & Manufacture, Vol. 47 566 No. 11, pp. 1660-6. MaClure, T.F., Adams, R., Gugger, M.D. and Gressel, M.G. (2007), “Comparison of flood vs. microlubrication on machining performance”, available at: www.unist.com/pdfs/articles/ AR_flood_v_micro.pdf (accessed January 30, 2009). Ngoi, B.K.A. and Sreejith, P.S. (2000), “Dry machining: machining of the future”, Journal of Materials Processing Technology, Vol. 101, pp. 287-91. Quaile, R. (2000), “Understanding MQL: machining with minimum quantity lubricant can save money and improve both tool life and part finish”, Modern Machine Shop, January, available at: http://findarticles.com/p/articles/mi_m3101/is_8_77/ai_n8695313 (accessed January 30, 2009). SCHUNK (2007), “For minimum costs and maximum protection of the environment”, Topic of the Month, available at: www.schunk-usa.com (accessed January 30, 2009). Shen, B., Shih, A. and Tung, S. (2008), “Application of nanofluids in minimum quantity lubrication grinding”, Tribology Transactions, Vol. 51 No. 6, pp. 730-7. Tasdelen, B., Thordenberg, H. and Olofsson, D. (2008a), “An experimental investigation on contact length during minimum quantity lubrication (MQL) machining”, Journal of Materials Processing Technology, Vol. 203 Nos 1-3, pp. 221-31. Tasdelen, B., Wikblom, T. and Ekered, S. (2008b), “Studies on minimum quantity lubrication (MQL) and air cooling at drilling”, Journal of Materials Processing Technology, Vol. 200 Nos 1-3, pp. 339-46. US Department of Health and Human Services (1998), “Occupational exposure to metal working fluid”, NIOSH Publication No. 98-102. Wakabayashi, T., Inasaki, I. and Suda, S. (2006), “Tribological action and optimal performance: research activities regarding MQL machining fluids”, Machining Science and Technology, Vol. 10, pp. 59-85. Zeilman, R.P. and Weingaertner, W.L. (2006), “Analysis of temperature during drilling of Ti6Al4V with minimal quantity of lubricant”, Journal of Materials Processing and Technology, Vol. 179, pp. 124-7. Further reading Wakabayashi, T., Inasaki, I., Suda, S. and Yokota, H. (2003), “Tribological characteristics and cutting performance of lubricant esters for semi-dry machining”, CIRP Annals, Vol. 51 No. 1, pp. 61-4. Corresponding author Nourredine Boubekri can be contacted at: [email protected] To purchase reprints of this article please e-mail: [email protected] Or visit our web site for further details: www.emeraldinsight.com/reprints