Preparation and Properties of Chitosan-Poly(N-isopropylacrylamide) Full-IPN Hydrogels PDF
Document Details
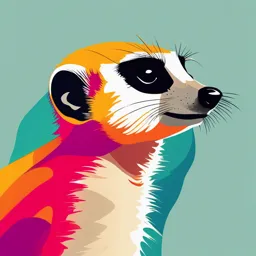
Uploaded by pepinos
Brock University
2001
Mingzhen Wang, Yu Fang, Daodao Hu
Tags
Related
- Chitosan Nanoparticle Delivery Systems for Sustainable Agriculture PDF
- Chitosan cross-linked poly(acrylic acid) hydrogels: Drug release control and mechanism PDF
- Chitosan: Structure, Properties, and Importance in Environmental Cleanup PDF
- Chitosan Hydrogels & Glutaraldehyde-Crosslinked Counterparts (PDF)
- Temperature-Sensitive Poly(N-isopropylacrylamide)-Chitosan Hydrogel for Fluorescence Sensors (PDF)
- Temperature-sensitive Poly(N-isopropylacrylamide)-chitosan Hydrogel for Fluorescence Sensors in Living Cells and Its Antibacterial Application PDF
Summary
This scientific paper describes the preparation and properties of a new type of hydrogel, a chitosan-poly(N-isopropylacrylamide) hydrogel. The synthesis and characterization of full-IPN hydrogels are reported in the paper. The properties of the hydrogels are studied and different aspects are considered, such as swelling behaviors in various solvents.
Full Transcript
Reactive & Functional Polymers 48 (2001) 215–221 www.elsevier.com / locate / react Preparation and properties of chitosan-poly(N-isopropylacrylamide) full-IPN hydrogels...
Reactive & Functional Polymers 48 (2001) 215–221 www.elsevier.com / locate / react Preparation and properties of chitosan-poly(N-isopropylacrylamide) full-IPN hydrogels Mingzhen Wang, Yu Fang*, Daodao Hu Department of Chemistry, Shaanxi Normal University, Xi’ an 710062, PR China Received 1 September 2000; accepted 8 January 2001 Abstract A new kind of full-IPN chitosan / poly(N-isopropylacrylamide) (CS / PNIPAM) hydrogels was prepared by chemical combination of methylene bis-acrylamide (MBAM) cross-linked PNIPAM network with formaldehyde (HCHO) cross-linked CS network. It was demonstrated that the properties of the gels, including the extractability of PNIPAM within it, the phase transition behavior, the swelling dynamics in aqueous phase, the swelling behavior in ethanol / water mixtures and even the microstructure are quite different from those of the semi-IPN CS / PNIPAM hydrogels, in which PNIPAM was simply embedded. Like the semi-IPN CS / PNIPAM hydrogels, however, the new gel is also temperature sensitive, that is the gel is transparent below 308C, whereas opaque above the temperature. It is expected that the finding of the new smart hydrogels may find some uses in separation science and in the design and preparation of new soft machines. 2001 Elsevier Science B.V. All rights reserved. Keywords: Chitosan; N-Isopropylacrylamide; Thermoresponsive gel; Hydrogel 1. Introduction volume transition [6–9]. Obviously, this may limit their applications in the areas of optics and Poly(N-isopropylacrylamide) (PNIPAM) is a material science. To overcome this shortcoming, well-known member of the thermo-responsive a thermo-stable hydrogel may be taken as a polymer family. The abrupt conformational matrix, in which PNIPAM or its modified forms transition of it upon changes at temperatures may be embedded. It is expected that the change around 328C has stirred up explorations for in temperature may induce the conformational technological applications [1,2]. Based upon its transition of the embedded polymer, and thereby conformational changes, various PNIPAM hy- make the hydrogels change between transparent drogels have been studied as controlled optical and opaque. Based upon these considerations, switches, limiters and modulators [3–5]. How- chitosan (CS) (mainly (1,4)-2-amino-2-deoxy- ever, the change is always accompanied by a b-D-glucan) was chosen as matrix gel forming material and CS / PNIPAM semi-IPN hydrogels were prepared in our previous work. It was *Corresponding author. Tel.: 186-29-5307534; fax: 186-29- 5307025 or 186-29-5307607. demonstrated that introduction of PNIPAM into E-mail address: [email protected] (Y. Fang). the CS gel yielded a new smart hydrogel. The 1381-5148 / 01 / $ – see front matter 2001 Elsevier Science B.V. All rights reserved. PII: S1381-5148( 01 )00057-8 216 M. Wang et al. / Reactive & Functional Polymers 48 (2001) 215 – 221 responsive property of it is not the traditional shaking. The mixture was agitated for another 5 volume phase transition, but the transparencey min and kept at room temperature for more than of it. However, the PNIPAM embedded within 24 h. The CS hydrogel without PNIPAM was the semi-IPN hydrogel is extractable when the prepared in a similar way. gel is exposed in water. To further the study The full-IPN CS / PNIPAM hydrogels were described above, a full-IPN hydrogel with tem- prepared in a different way. The details are as perature response was synthesized from CS and follows. CS (500 mg) and NIPAM (315 mg) N-isopropylacrylamide (NIPAM) in the pres- were suspended in 25 ml of water. To the ence of a suitable crosslinker and an initiator. suspension 5 ml of acetic acid solution (3 mol ? The composition and microstructure of the gel, cm 23 ) were added with stirring. The mixture the extractability of PNIPAM within the gel, the was stirred for 15 min to make sure that CS was phase transition and the swelling behaviors of dissolved completely. The solution was degas- the gel both in water and in ethanol / water sed for 5 min at room temperature. MBAM mixtures were studied. solution (30 mg / ml, 0.5 ml), KPS solution (25 mg / ml, 0.5 ml), SBS solution (20 mg / ml, 0.5 ml), TEMED solution (50 mg / ml, 0.5 ml) and 2. Experimental HCHO (2 ml, 37%) were added consecutively to the degassed solution with stirring. The 2.1. Chemicals solution was kept at room temperature for more than 24 h. NIPAM and CS were prepared and purified, respectively, by the methods described before 2.3. Composition and microstructures of the [10,11]. Methylene bis-acrylamide (MBAM) gels was purified by recrystallization from methanol. PNIPAM was synthesized and purified in the The xerogels used for IR and SEM measure- way reported earlier. Other chemicals such ments were prepared by rapidly freezing a cube 3 as formaldehyde (HCHO), potassium persulfate (approx. 2 cm ) of the gels at 2 608C (liquid (KPS), sodium bisulfite (SBS), tetramethylene- nitrogen) and lyophilized. FTIR spectra of all diamine (TEMED), acetic acid, methanol and samples pelletized with KBr were recorded ethanol were of at least analytical grade and using an Equinox 55 Fourier transform infrared used without further purification. Deionized and spectrometer, and the SEM measurement was doubly distilled water was used throughout this performed using a Hitachi S-570 electron work. microscope. 2.2. Preparation of the hydrogels 2.4. Extraction of PNIPAM from the gels The CS / PNIPAM semi-IPN hydrogels were A cube of the prepared hydrogels (1.0 g) was prepared in the following procedures: CS (500 immersed in 20 ml of pure water. The variation mg) and PNIPAM (315 mg) were suspended in of the transmittance (480 nm) of the extraction 25 ml of deionized water. To the suspension 5 with time was monitored using an UV-260 ml of acetic acid solution (3 mol dm 23 ) were spectrometer. The extraction experiment was added with stirring. The mixture was vigorously conducted at 15 and 408C, respectively. stirred for more than 15 min to make sure that CS was dissolved completely. The solution was 2.5. Phase transition temperature of the gels thoroughly degassed via vacuum extraction. After degassing, HCHO solution (37%, 2 ml) A cuvette containing CS, semi-IPN CS / was added slowly to the polymer solution with PNIPAM or full-IPN CS / PNIPAM hydrogels M. Wang et al. / Reactive & Functional Polymers 48 (2001) 215 – 221 217 was put into the sample holder with an online temperature controller system of the UV spec- trometer. The transmittance of the sample at various temperatures were recorded using 480 nm as an absorption wavelength. 2.6. Swelling dynamics of the gels The gels obtained were removed from the vessel, sliced into disks (about 3 mm thick), and washed with water for several times. Swelling experiment was conducted in the following way. Immersing the gel disks (m 0 , triplicates) in a given solvent. The approach to equilibrium was monitored by periodically withdrawing of the Fig. 1. FTIR spectra of various xerogels: (a) PNIPAM; (b) CS; (c) disks from the solvent and weighing (m s ) after full-IPN CS / PNIPAM; (d) semi-IPN CS / PNIPAM. removal of the excess surface water by light blotting with a filter paper. Each disk weight the semi-IPN xerogels, the profile of the full- was individually monitored in the way de- IPN xerogels resembles, however, to that of CS, scribed until it reaches a constant value. The except that the absorption within the range of relative swelling ratio (R) was expressed as 21 1750–1250 cm is apparently enhanced. Inter- R 5 m s /m 0. estingly, the microstructure of the full-IPN xerogel is also very similar to that of the CS 2.7. Swelling behaviors in ethanol /water xerogel (Fig. 2a,b). Clearly, the similarities in mixtures FTIR spectra of the full-IPN CS / PNIPAM hydrogel and CS hydrogel may show that the Eleven disks of the gels were weighted, networks of the CS gel was fully encompassed respectively (m i , i51–11), and immersed into with the networks of PNIPAM. The differences various composition of ethanol / water mixtures. between the semi-IPN and CS hydrogels (Fig. Each disk was withdrawn and weighted (m si , 2a,b), however, show that the two CS / PNIPAM i51–11) 24 h later. The equilibrated swelling hydrogels are different structurally. ratio for each gel at a given composition of The differences between the two CS / ethanol / water mixture was calculated in the PNIPAM hydrogels are further confirmed by way described in the previous section. water extraction experiment. The main results are shown in Fig. 3. As expected, at tempera- 3. Results and discussion tures below 308C, the quantity of PNIPAM remained within the semi-IPN hydrogels de- 3.1. Composition and microstructures of the creases dramatically with time. With continuous gels extraction, the PNIPAM within the gel can be extracted completely. This observation may be a Fig. 1 shows the FTIR spectra of the purified result of weak interaction between CS and the PNIPAM (Fig. 1a), the xerogels of CS (Fig. 1b), embedded PNIPAM. However, for the full-IPN full-IPN CS / PNIPAM (Fig. 1c) and the semi- CS / PNIPAM hydrogels the extraction result is IPN CS / PNIPAM (Fig. 1d). The profiles of the fully different. With reference to Fig. 3, it can semi-IPN xerogels and the full-IPN xerogels are be seen that the quantity of PNIPAM within the characterized by both the absorption of gel decreases little with time. This is a strong PNIPAM and that of CS. Compared to that of evidence in support of the tentative conclusion 218 M. Wang et al. / Reactive & Functional Polymers 48 (2001) 215 – 221 Fig. 2. General surface view of various xerogels: (a) CS; (b) semi-IPN CS / PNIPAM; (c) full-IPN CS / PNIPAM. PNIPAM may physically encompass on the CS network, and thereby PNIPAM cannot be effec- tively extracted. 3.2. Phase transition temperature of the gels The temperature dependence of the phase transition of the various gels is depicted in Fig. 4. Reference to this figure reveals that compared to the CS gels, both the semi-IPN and the full-IPN CS / PNIPAM hydrogels shows clear phase transition within the temperature range Fig. 3. Transparency of the two CS / PNIPAM hydrogels as functions of extraction time ( l 5480 nm). that PNIPAM within the full-IPN hydrogel is cross-linked and encompassed with the CS network. It may be interesting to look at the extractability of PNIPAM within the two gels at temperatures above the LCST of PNIPAM. The results showed in the small Fig. inserted in Fig. 3 demonstrated clearly that at the experimental temperature PNIPAM within any of the two gels cannot be significantly extracted. This is not a surprising result as the solubility of PNIPAM is Fig. 4. Transparency of the two CS / PNIPAM and CS hydrogels so low at this temperature that the precipitated as functions of temperature ( l 5480 nm). M. Wang et al. / Reactive & Functional Polymers 48 (2001) 215 – 221 219 tested. Furthermore, the phase transition tem- perature of the full-IPN hydrogels is at least 4–58C greater than that of the corresponding semi-IPN hydrogels. The difference of the two gels in the phase transition behavior may be attributed to the difference in their microstruc- tures. The fact that the phase transition tempera- ture of the full-IPN hydrogels shifts to higher temperature indicates that polymerization of NIPAM in the presence of divinyl crosslinker and CS may result in the formation of PNIPAM network and multi-point grafting of it onto CS network. Clearly, the chemical combination of CS network to PNIPAM is something like Fig. 5. Plots of swelling ratio against the swelling time for the hydrophilic modification of PNIPAM. It is two CS / PNIPAM gels at two different temperatures. reported by a number of groups [12–15] that hydrophilic modification of PNIPAM results in peratures as a function of time. Inspection of the shifts of its LCST to higher temperature. There- figure reveals that the semi-IPN hydrogels swell fore, it is not surprising that the phase transition faster than the corresponding full-IPN hydro- temperature of the full-IPN hydrogels is greater gels, and the swelling ratio data for the semi- than that of the semi-IPN hydrogels. It is also IPN hydrogels are almost independent of tem- reported that the phase transition of PNIPAM perature at which the experiment was con- becomes more diffusion with hydrophilic modi- ducted. For the semi-IPN hydrogel, at tempera- fication. For the present study, however, ture (158C) below the LCST of PNIPAM the the phase transition of the full-IPN hydrogels is swelling ratio increases from 1 to 15 with time. also very sharp, indicating that the conforma- The two gels behaved similarly at temperature tional transition (the cause for the phase transi- (388C) greater than the LCST of the polymer. tion) of PNIPAM within the gel is relatively This is not surprising because PNIPAM, as independent of CS. described earlier, was simply trapped in the CS It is a little bit surprising that the LCST of network and change in temperature has little PNIPAM in the semi-IPN hydrogels is about 258C, a result significantly lower than that of effect upon the swelling behavior of the CS PNIPAM in water. This may be attributed to the network. This argument was further sup- high ionic strength of the medium in which ported by the observation of swelling behavior PNIPAM was dissolved. A similar result has of the full-IPN hydrogels at different tempera- been reported by Park and Hoffman. In tures (Fig. 5). It can be seen from Fig. 5 that at fact, studies in our laboratory also demonstrated temperature 158C, the swelling ratio of the full- that introduction of a salt into PNIPAM solution IPN hydrogels increases gradually with time, will alter the phase transition temperature of the and reaches equilibrium within 200 min. At polymer. The degree of the alteration depends temperature (388C) greater than the LCST of upon both the salt nature and the concentration PNIPAM, the swelling ratio increases linearly of it. with time. It was also found that the mechanical strength of the full-IPN hydrogel at swelling 3.3. Swelling properties of the gels ratio greater than 6 is too weak to handle, indicating the possible break down of the net- Fig. 5 depicts the swelling ratios of the two works. Far from that expected that the full-IPN CS / PNIPAM hydrogels at two different tem- hydrogels swell much faster at 388C than that at 220 M. Wang et al. / Reactive & Functional Polymers 48 (2001) 215 – 221 158C. This observation can only be attributed to tion in gel volume and weight may be useful for the break down or partially break down of the separation purposes. gel network. It is known that the swelling ratio of a polymer network is determined by a balance of attractive forces and repulsive forces. 4. Conclusions For the full-IPN CS / PNIPAM hydrogels, at temperature greater than the LCST, the CS It has been demonstrated that chemical network has a strong tendency to swell in combination of CS and PNIPAM networks aqueous phase, but the PNIPAM network tends yielded a new kind of full-IPN hydrogels. to contract due to collapse of the polymer coil. Unlike the semi-IPN CS / PNIPAM hydrogels, Clearly, a strong inner-stress may exist within chemical combination of PNIPAM into the CS the gel. Based upon discussion above, it can be networks has a great effect upon the properties concluded that the abnormal swelling behavior of the gel, including the swelling dynamics in of the full-IPN hydrogel at high temperature aqueous phase, swelling ability in ethanol / water could result from the stress cracking of the gels. mixtures, the extractability of PNIPAM within The swelling behavior of the full-IPN hydro- the gel and even the microstructure of it. The gels in ethanol / water mixtures is also different new gel is also temperature sensitive. However, from that of the semi-IPN hydrogels. With the responsive property of the new gel is not the reference to Fig. 6, it can be noted that the traditional volume phase transition of PNIPAM swelling ratios of the full-IPN hydrogels kept gels, but the transparencey of it. It is expected almost constant as long as the concentration of that the finding of the new smart hydrogel may ethanol was lower than 30% (v / v). Above that find some uses in separation science and in the concentration, the swelling ratio starts to de- design and preparation of soft machines. crease along with increasing ethanol concen- tration. When ethanol concentration is beyond Acknowledgements 70% (v / v), the swelling ratio kept constant again. In other words, there is a sharp transition Funding from the National Natural Science in the swelling ratio as the concentration of Foundation of China (29973024), Ministry of ethanol increases from 30 to 70% in volume Education of the PRC, and the Natural Science (the small figure inserted in Fig. 6). The transi- Foundation of Shaanxi Province are greatly appreciated. References K. Dusek, in: Advances in Polymer Science, Responsive Gels, Volume Transitions II, Springer, Berlin, 1993. A.S. Hoffman, P.S. Stayton, T. Shimoboji, Macromol. Symp. 118 (1997) 553–563. A. Suzuki, T. Tanaka, Nature 346 (1990) 345–347. J.M. Weissman, H.B. Sunkara, A.S. Tse, S.A. Asher, Science 274 (1996) 959–960. S.A. Jenekhe, X.L. Chen, Science 283 (1999) 372–375. S. Fujishige, K. Kubota, I. Ando, J. Phys. Chem. 93 (1989) 3311–3313. K. Kubota, S. Fujishige, I. Ando, J. Phys. Chem. 94 (1990) 5154–5158. Fig. 6. Plots of equilibrium swelling ratio against the composition X.Y. Shi, S.K. Wu, C.M. Sun, Acta Polym. Sininca 2 (1999) of the ethanol / water mixtures for the two CS / PNIPAM hydrogels. 210–216, (in Chinese). M. Wang et al. / Reactive & Functional Polymers 48 (2001) 215 – 221 221 T. Okano, in: Biorelated Polymers and Gels, Academic M.J. Snowden, B.Z. Chowdhry, B. Vincent, G.E. Morris, J. Press, Boston, MA, 1998. Chem. Soc. Faraday Trans. 92 (1996) 5013–5016. M.Z. Wang, J.C. Qiang, Y. Fang, D.D. Hu, Y.L. Cui, X.G. Fu, S.Q. Zhou, B. Chu, J. Phys. Chem. B 102 (1998) 1364– J. Polym. Sci. Part A: Polym. Chem. 38 (2000) 474–481. 1371. Y. Fang, S.X. Liu, D.D. Hu, Y.L. Cui, M. Xue, Polym. Bull. T.G. Park, A.S. Hoffman, Macromolecules 26 (1993) 5045– 43 (1999) 387–394. 5048. H. Ringsdorf, J. Simon, F.M. Winnik, Macromolecules 25 M.Z. Wang, L.N. Gao, Y. Fang, D.D. Hu, J. Shaanxi Normal (1992) 5353–5361. Univ. (Nat. Sci. Edn.) 28 (2000) 41–44, (in Chinese). Y. Fang, J.C. Qiang, D.D. Hu, M.Z. Wang, Y.L. Cui, Colloids Y. Fang, D.D. Hu, Y.L. Cui, High Tech. Commun. 11 (3) Polym. Sci. 279 (2001) 47–52. (2001) 56–62.