Temperature-sensitive Poly(N-isopropylacrylamide)-chitosan Hydrogel for Fluorescence Sensors in Living Cells and Its Antibacterial Application PDF
Document Details
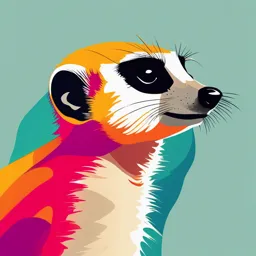
Uploaded by pepinos
Jilin University
2021
Lifeng Xu, Xiao Liang, Liru You, Yongyan Yang, Gangying Fen, Yan Gao, Xuejun Cui
Tags
Related
- W10 Temperature Regulation (Connolly) PDF
- Lecture Notes on Bacterial Specimen Transport PDF
- Lecture 5 BBT317 Molecular Genetics Mutation and Repair Part V PDF
- Temperature-Sensitive Poly(N-isopropylacrylamide)-Chitosan Hydrogel for Fluorescence Sensors (PDF)
- Food Safety Procedures PDF
- Chapter 15: Low-Temperature Sterilization PDF
Summary
This article details the synthesis of a temperature-sensitive hydrogel containing organic fluorescent materials. The work focuses on creating a biocompatible hydrogel with aggregation-induced emission properties for cellular temperature sensing. The hydrogel is designed for use in biomedicine, potentially as a drug delivery carrier.
Full Transcript
International Journal of Biological Macromolecules 189 (2021) 316–323 Contents lists available at ScienceDirect International Journal of Biological Macromolecules...
International Journal of Biological Macromolecules 189 (2021) 316–323 Contents lists available at ScienceDirect International Journal of Biological Macromolecules journal homepage: www.elsevier.com/locate/ijbiomac Temperature-sensitive poly(N-isopropylacrylamide)-chitosan hydrogel for fluorescence sensors in living cells and its antibacterial application Lifeng Xu a, Xiao Liang b, Liru You a, Yongyan Yang a, Gangying Fen a, Yan Gao a, c, Xuejun Cui a, c, * a College of Chemistry, Jilin University, Changchun 130012, PR China b Key Laboratory for Molecular Enzymology and Engineering of Ministry of Education, School of Life Sciences, Jilin University, Changchun 130012, China c Weihai Institute for Bionics-Jilin University, Weihai 264400, PR China A R T I C L E I N F O A B S T R A C T Keywords: It is meaningful and challenging to design and develop a fluorescent probe for living cell temperature sensors Temperature stimulus since it should have good cell compatibility and high-resolution features. In this work, the temperature-sensitive Aggregation-induced emission enhancement polymer of PA-loaded cysteine (Cys) modified chitosan (Cs) grafted PNIPAM (Cs-Cys-PN/PA) with aggregation- Antibacterial drugs carrier induced emission enhancement (AIEE) properties that reversible hydrogel in an aqueous solution is synthesized. Here, we interpret the temperature stimulus as a monochromatic signal through the AIEE active reversible hydrogel of Cs-Cys-PN. In addition, the cytotoxicity test shown that Cs-Cys-PN has good biocompatibility. Cs-Cys- PN can be used to build antibacterial drugs carrier, thereby providing a new platform of self-released drugs for the treatment of bacterial infections. 1. Introduction temperature of cell [16,17]. Especially, thermosensitivity polymers with amphiphilic blocks have attracted great attention from investigators Fluorescent organic materials have been paid close attention from. In most polymers, due to poly(N-isopropylacrylamide) (PNIPAM) people due to the facile operation [1,2], higher efficiency , and has the adjustable of phase transition temperature, it has become a hot excellent selectivity. However, majority traditional organic mate research in thermosensitive materials by adjusting the composition of rials have aggregation-caused quenching (ACQ) effect, which heavily copolymer [19,20]. Moreover, the thermosensitive polymer, PNIPAM, limiting their application in bioimaging [5,6]. The concept of AIE was has a lower critical solution temperature (LCST) in water, which has first proposed from tang and colleagues, which is to say, molecules in the been confirmed by different researchers in concentrated and dilute so dilute solution do not emit light due to the active movement between the lutions. PNIPAM has the sensitivity temperature sensitivity and molecules, but they can emit strong light when in concentrated solution good biocompatibility, it has been broadly used in the fields of or aggregation state [7,8]. Since most traditional AIE or AIEE materials biomedicine, particularly in the system of drug delivery. were manufactured by the way of organic synthesis [9,10], thus, some Chitosan (Cs) is a derivative obtained by partial deacetylation of natural AIE or AIEE materials are urgently needed with the low cost chitin. Chitin is the second largest natural biopolymer that exten , easy operation. Thus, palmatine (PA), as previously reported, sively distributed on the earth. It is composed of D-glucosamine and it is a natural AIE material which come from the Fibraurea recisa Pierre of N-acetyl-D-glucosamine. It is connected by glycosidic bond at the stems and roots. PA has good water solubility and has the unique β-(1,4) position and is widely used in biomedical field. Chitosan is a structure of donor and acceptor, moreover, it is broadly used in the fields kind of polysaccharide which is rich in nature resource. It has the on bacterial diarrhea. bioactive property due to the antibacterial activity, non-toxic, easy to In the parameters of the human body, temperature is an important modify, good biodegradable. physical index of the cell, since it can adjust many biochemical reaction Here, a polymer fluorescent probe with temperature-responsive of processes in the cell [14,15]. In the past decades, some polymers of AIEE property based on chitosan was designed and synthesized. Due to fluorescent probes have played an important role of explaining the the competition between the isopropyl group-linked amide and the * Corresponding author at: College of Chemistry, Jilin University, Changchun 130012, PR China. E-mail address: [email protected] (X. Cui). https://doi.org/10.1016/j.ijbiomac.2021.08.057 Received 21 June 2021; Received in revised form 28 July 2021; Accepted 6 August 2021 Available online 12 August 2021 0141-8130/© 2021 Elsevier B.V. All rights reserved. L. Xu et al. International Journal of Biological Macromolecules 189 (2021) 316–323 hydrogen bonds of water, the PNIPAM on PA-loaded Cys-modified- Transient Fluorescence Spectrometer (FLS 920 Edinburgh Instrument). Chitosan grafted PNIPAM (Cs-Cys-PN/PA) hydrogel will shrink or Cell viability was studied by GF-M 3000 microplate reader (Shandong, extend with temperature changes. The reversible change of space re China). Cell imaging was researched by confocal laser scanning micro alizes thermochromism by changing the intramolecular motion of PA. scope (CLSM 710, Carl Zeiss Microscopy LLC, Jena, Germany). Finally, this biocompatible thermally responsive material was proved to be effective in terms of intracellular temperature. Moreover, due to Cs- Cys-PN/PA hydrogel can be released stably and slowly at 37 ◦ C, thus, the 2.4. In vitro stability test of CS-Cys-PN/PA hydrogel Cs-Cys-PN/PA hydrogel has better antibacterial properties. In this study, 100 mg of PA was added into 2 mL Cs-Cys-PN/PA 2. Experimental section hydrogel, and put it into a dialysis tube (MWCO 3.5 KDa), soaked in 20 mL PBS solution, and incubating at 37 ◦ C and 25 ◦ C, shaking at 150 2.1. Materials rpm in shaking incubator. Taking out 500 μL solution at different time intervals (2, 4, 6, 8, 10, 12, 24 h), meanwhile, replaced with 500 μL of N-isopropylacrylamide (NIPAM 98%, purity) was acquired to fresh PBS solution. And the released amount of PA was analyzed by Shanghai Macklin Biochemical Co., Ltd. Chitosan (Cs ≥ 95% deacety UV–Vis. The drug release percentage (%) was determined by comparing lated, 150–275 kDa, viscosity 100–200 mpa.s dissolved in 1% acetic the cumulative amount of PA recovered in the release medium at each acid) was purchased from Shanghai Macklin Biochemical Co., Ltd. certain time point with the total amount of PA. The experiment was Acetic acid was purchased from Tianjin Tiantai Fine Chemical Co., Ltd. repeated three times to ensure accuracy. L-Cysteine (Cys, 98%, purity) was acquired from Sinopharm Chemical Reagent Co., Ltd. Palmatine (PA, 98%, purity) was obtained from 2.5. Cellular cytotoxicity study Shanghai Macklin Biochemical Co., Ltd. N-hydroxysuccinimide (NHS 98%, purity) was obtained from Shanghai Macklin Biochemical Co., Ltd. Hela cells were seen in 96-well plates at a quantity of 8000 cells/well N-(3-Dimethylaminopropy)-N-(ethylcarbodiimide hydrochloride) (EDC in 100 μL DMEM containing 10% FBS and incubated at 37 ◦ C for 12 h. 99%, purity) was acquired from Shanghai Macklin Biochemical Co., Ltd. After removing the medium, the cells were washed with PBS and treated Ammonium persulfate (APS 98%, purity) was obtained from Shanghai with Cs-Cys-PN at a different concentration from 20 to 100 μg/mL. The Macklin Biochemical Co., Ltd. Fetal bovine serum (FBS 99%, purity) was cells were then cultured for another 24 h, and treated with 15 μL of MTT obtained from Gibco (Grand Island, USA). 3-(4,5-Dimethylthiazol-2-yl)- solution (5 mg/mL) for 4 h. Afterwards, the medium was removed, and 2,5-diphenyltetrazolium bromide (MTT) was obtained from Amersco 200 μL DMSO was used to dissolve the blue purple formazan crystals. (Solon, USA). S. aureus (BNCC186335) and Nutrient-Agar-Medium was The plates were subsequently shaked for 2 min and subjected to GF-M obtained from Henan Putian Tongchuang Biotechnology Co. Ltd. 3000 microplate reader (Shandong, China) for measuring the absor bance at 492 nm. The cell viability (%) was detected by the ratio of the 2.2. Synthesis of PN-Cs-Cys/PA hydrogel absorbance values of the treated group and the blank group. Briefly, 300 mg Chitosan (Cs) was dissolved in 30 mL 1% acetic acid aqueous solution, 100 mg N-hydroxysuccinimide (NHS) and 150 mg N- 2.6. Cellular uptake and imaging (3-Dimethylaminopropy)-N-(ethylcarbodiimide hydrochloride) (EDC) were add into the Cs solution, after they were completely dissolved, 250 Hela cells were respectively seeded in plates and cultured with mg L-Cysteine (Cys)was added into the Cs solution, and the reaction was sterilized coverslips at 37 ◦ C for 12 h, then incubated of Cs-Cys-PN/PA allowed to proceed overnight under constant stirring (500 rpm). The solution with 100 μg/mL for 4 h. Next, it was washed with PBS, fixed reaction is under N2 atmosphere to prevent -SH from being oxidized. with 75% ethanol and treated with 4,6-diamidino-2-phenylindole After reacting completely, the product of Cs-Cys was purified via dialysis (DAPI). Finally, the confocal laser scanning microscope (CLSM 710 (MWCO 8–14 kDa) used ultrapure water for two days. The dialyzed Carl Zeiss Microscope LLC, Jena, Germany) was used to analyze the product was lyophilized and stored for further use. coverslips of Cs-Cys-PN/PA fluorescent intensity under 37 ◦ C and cool 300 mg lyophilized Cs-Cys was fully dissolved in 30 mL aqueous ing at 25 ◦ C. solution, then 1.0 g N-isopropylacrylamide, purified and recrystallized with n-hexane, the mixtures were introduced to nitrogen for 20 min under continuous stirring until mixtures were dissolved. Then 200 μL 2.7. Antibacterial activity assay 0.1 w/v% ammonium persulfate (APS) as initiator was added into the mixtures. The reaction process was maintained for 4 h at 70 ◦ C under N2 Sterilize the control agar medium and (0.0125–0.2) μmol/mL PA, Cs- atmosphere to synthesize the Cs-Cys-PN. After reacting completely, the Cys-PN/PA in 250 mL Erlenmeyer flasks, related equipments are ster mixtures were dialysis (MWCO 3.5 kDa) by using ultrapure water for ilized in an autoclave at 121 ◦ C for 20 min. The subsequent operation is two days. The product was frozen for further use. carried out in the vertical ultra-clean workbench (Shanghai Sujing In dustrial Co., Ltd. SW-CJ-2D). After the medium was solidified, filling the 2.3. Characterization middle of each petri dish with a 5 mm fungal inoculum (the mycelial growth side is facing down). Last, the inoculum was cultured at 37 ◦ C for The Cs-Cys-PN/PA was analyzed by Fourier Transform Infrared 5 days. Each treatment was repeated three times. The diameter of S Spectroscopy (FT-IR IRAffinity-1, SHIMADZU), and CHNS elemental aureus is determined by measuring two intersecting lines passing analyzer (Vario EL III). 1H NMR of Cs-Cys-PN was dissolved in D2O through the center of a 5 mm disc. The diameter is recorded when the deuterium oxide (D2O, 99.9% D, abcr GmbH, Germany) which hypha is near the edge of the control disc. The formula for the inhibition measured by using a Bruker Avance 400 MHz spectrometer (Bruker rate is as follows. Corp., Karlsruhe, Germany). The gelation time of the Cs-Cys-PN/PA was (C − d) − (T − d) studied by the “Visual Tube Inversion Method” as previous research. To I% = × 100% (C − d) investigate the morphology of Cs-Cys-PN/PA, scanning electron micro scope (SEM SU8020 HITACHI) was used to study the morphology of Cs- The C is the diameter for the control group, d is the diameter for the Cys-PN/PA and the morphology of S. aureus. The emission spectra and original group (5 mm), T is the diameter of which was treated with fluorescent lifetimes of Cs-Cys-PN/PA was studied by Steady State sample, and I is the inhibition rate (%). 317 L. Xu et al. International Journal of Biological Macromolecules 189 (2021) 316–323 Scheme 1. Graphical representation of synthesis of Cs-Cys-PN polymer. Fig. 1. (A) FT-IR spectra of (a) Cs-Cys, (b) Cs (c) NIPAM (d) Cs-Cys-PN. (B) Single Cs-Cys, (C) The magnified infrared characteristic peak of sulfhydryl group of Cs-Cys.(D) 1H NMR spectra of Cs-Cys-PN. Table 1 Table 2 CHNS element of Cs-Cys. CHNS element of Cs-Cys-PN. Element C H N S Element C H N S Mean value 41.44 7.60 5.37 1.37 Mean value 40.50 7.44 6.98 1.15 Deviation, abs 0.29 0.30 0.03 0.01 Deviation, abs 0.05 0.45 0.02 0.06 2.8. Morphology observation of bacteria acquired by centrifugating (8000 rpm, 5 min) and were washed several times with PBS. The samples were fixed with 2.5% glutaraldehyde and The morphological of S. aureus were studied by SEM. S aureus was co- washed by PBS buffer. The sample is dehydrated by using the concen cultured with 0.05 μmol/mL Cs-Cys-PN/PA for 12 h. S aureus were tration of ethanol 75% at three times. S aureus growing in blank culture 318 L. Xu et al. International Journal of Biological Macromolecules 189 (2021) 316–323 Fig. 2. (A), (B) SEM images of Cs-Cys-PN/PA. (C) Phase diagram of Cs-Cys-PN/PA, (D) Gelation time of Cs-Cys-PN/PA. (E) The storage (G′ ) and loss (G′′ ) moduli of Cs-Cys-PN/PA. medium was used as control group. After drying in air and coating by the stretching vibrations of the amide I and amide II bands of NIPAM, gold sputtering, the sample was observe by SEM. the absorption peak of 2907 cm− 1 was owed to the stretching vibrations for C–H of NIPAM. The absorption peak of 1650 cm− 1 was attributed to 3. Results and discussion the stretching vibrations for C–– O of NIPAM, the absorption peak of 3350 cm− 1 was owed to the stretching vibrations for N–H of Cs-Cys-PN, The synthetic route of Cs-Cys-PN hydrogel is shown from Scheme 1. which indicated that NIPAM had been coupled on Cs-Cys successfully. The lyophilized powder of Cs-Cys-PN was analyzed by FT-IR, as shown As shown from Fig. 1B, 1.00 ppm (a, d), which are dominant in NIPAM. from Fig. 1A, the characteristic absorption peak of 1620 cm− 1 was The overlap of -NH (b) and the –H of the ring for the Cs (2, 3, 4), at 3.56 attributed to the amino of stretching vibration of Cs, the absorption peak ppm of –H of ring of (1) for Cs , in addition, the peaks of 1.78 ppm of 2876 cm− 1 was owed to the stretching vibration of the C–H of Cs, the and 2.12 ppm were attributed to the methyne -CH) (c) and methyne absorption peak of 3450 cm− 1 was corresponded to the stretching vi (-CH) (f), 2.42 ppm was owed to the CH-SH (e) which indicated that the bration for hydroxyl of Cs, the characteristic absorption peak at 2559 successful coupling from Cs-Cys. The CHNS of Table 2 also indicted cm− 1 was owed to the stretching vibration for the sulfhydryl group of that Cs-Cys-PN was synthesized successfully. The grafting rate of NIPAM Cys, which indicated that the Cys had been coupled on Cs successfully to Cs-Cys was 45.6%. Moreover, the yield for the Cs-Cys-PN was 76.8%. [28,29]. Moreover, since the characteristic absorption peaks of thiol In order to get more information about Cs-Cys-PN/PA, SEM was used groups were very weak, the CHNS elemental analysis (Table 1) was to distinguish the morphology of Cs-Cys-PN/PA. As shown from Fig. 2A tested to prove that the Cys was successfully grafted to Cs. The charac and B, Cs-Cys-PN/PA has high porosity, good interconnectivity and teristic absorption peaks at 1600 cm− 1 and 1500 cm− 1 were attributed to uniform pore size distribution, moreover, due to the introduced of thiols 319 L. Xu et al. International Journal of Biological Macromolecules 189 (2021) 316–323 Fig. 3. Images of the Cs-Cys-PN/PA under (A1) natural light and (A2) under UV light. (B) Fluorescent intensity of Cs-Cys-PN/PA with different temperature under pH = 7.4. (C) Peak intensities for different temperature of Cs-Cys-PN/PA. (D) Lifetimes of fluorescent intensity of Cs-Cys-PN/PA with different temperature under pH = 7.4. (E) Fluorescent intensity of Cs-Cys-PN/PA at several cycles by adjusting temperature between 24 ◦ C and 40 ◦ C. which get a better crosslink density.nd the pore size from about 1 μm to 2 μm. To study the lower critical solution temperature (LCST) of Cs-Cys- PN/PA hydrogel, as shown from Fig. 2C, when the temperature reaches the LCST of Cs-Cys-PN/PA, due to the isopropyl hydrophobic interaction is dominant, and the thermosensitive PNIPAM chain is removed from the hydrate disk, the PNIPAM volume shrinks into dehydrated block hydrophobic hydrogel, and the water is squeezed out without forming hydrogen bond. As shown from Fig. 2D, with the temperature increased, the gelation time decreased significantly. As described from Fig. 2E, rheological properties of Cs-Cys-PN/PA hydrogel was measured, as the temperature increased, the turning point of which the storage and loss modulus (G′ and G′′ data) were significantly increased was 33 ◦ C. It also indicated that phase transition temperature of Cs-Cys-PN/PA was 33 ◦ C. When it reaches the maximum value, the viscosity data remains almost unchanged, which indicated that the formed Cs-Cys-PN/PA hydrogel was stable on increasing the temperature. These results all indicted that the Cs-Cys-PN/PA hydrogel with excellent gelling properties. To further study the fluorescent properties of Cs-Cys-PN/PA, the PL spectra was analyzed, as shown from Fig. S1, enhanced emission was found as the fraction of THF (fT) increasing from 0% to 90%, the results of enhanced Cs-Cys-PN/PA emission in THF/water (90/10) was that the Fig. 4. In vitro PA release from Cs-Cys-PN hydrogel under different temperature aggregation of Cs-Cys-PN/PA in poor solvent, THF. These results all at pH = 7.4. Error bars indicate ± s.d. (n = 3). proved that the AIE or AIEE characteristic of Cs-Cys-PN/PA. Due to the thermosensitivity property of Cs-Cys-PN/PA, which is crucial in the fluorescent function. As shown from Fig. 3A1 and A2, the phase 320 L. Xu et al. International Journal of Biological Macromolecules 189 (2021) 316–323 Fig. 5. CLSM imaging of hela cells incubated with Cs-Cys-PN/PA measured under 25 ◦ C and 37 ◦ C at pH = 7.4. Concentration: 100 μg/mL, λex = 405 nm. The images were acquired of magnification in 80. polymer chain of PNIPAM does not restrict the free motion of PA mol ecules, thus, Cs-Cys-PN/PA exhibits weak green fluorescence. However, once the temperature of the Cs-Cys-PN/PA reaching the LCST, the PNIPAM block begins to become hydrophobic and it collapse to form micelles, wrapping more PA in the core of the micelles, restricting the free intramolecular molecular motion of the PA, enhanced green emis sion of Cs-Cys-PN/PA [16,22]. These results all indicated that the increasing of temperature will lead to the enhancement of the fluores cent intensity of PA. As shown from Fig. 3D, when the temperature is higher than the LCST (32 ◦ C) of Cs-Cys-PN/PA, as the temperature in creases, the emission lifetime of Cs-Cys-PN/PA gradually becomes longer. For the reversible cycle experiment, the result was shown in Fig. 3E, since the phase transformation from hydrophilic to hydrophobic of PNIPAM in Cs-Cys-PN at 25 ◦ C and 40 ◦ C was reversible, the PL in tensity of Cs-Cys-PN/PA was reversible, namely, it could be “turn-on” and “turn-off” repeatedly. These results all shown that the fluorescent intensity is controllable. To better understand the drug released carrier of Cs-Cys-PN/PA. The loading efficiency of PA was 96.8%. The experiment of in vitro PA release from Cs-Cys-PN hydrogel was conducted under 25 ◦ C and 37 ◦ C at pH = 7.4, respectively. As shown from Fig. 4, PA can quickly reach stability within 4 h, under the condition of 37 ◦ C, only 54.74% ± 3.5% of PA was released from the Cs-Cys-PN hydrogel at 24 h, however, under the condition of 25 ◦ C, Cs-Cys-PN/PA hydrogel released about 90.46 ± 2.97% of PA. This indicated that Cs-Cys-PN/PA hydrogel can maintain a stable and slow release under the condition of phosphate buffered saline (PBS) at 37 ◦ C. Thus, it can be used as a stable sustained drug delivery carrier. After evaluating of the fluorescent property of Cs-Cys-PN/PA, the Fig. 6. (A) PA, Cs-Cys-PN/PA inhibition ratio against S. aureus. (B) The number biocompatibility of Cs-Cys-PN was studied since it is crucial for the of viable S aureus treated with blank culture medium and 0.05 μmol/mL of PA, application of bioimaging. MTT experiments indicated that Cs-Cys-PN 0.05 μmol/mL of Cs-Cys-PN/PA in the temperature of 37 ◦ C. *, P < 0.05. had good biocompatibility (Fig. S2). In order to study the effect of temperature on the bioimaging of Cs-Cys-PN/PA, hela cells were transition state of Cs-Cys-PN/PA under natural light and UV light, cultured with Cs-Cys-PN/PA (100 μg/mL) for 4 h and used to study the respectively. When reaching the LCST of Cs-Cys-PN/PA, PNIPAM arm effect of temperature on Cs-Cys-PN/PA in bioimaging and cell imaging shrinks and collapses to form micelles, due to the hydrophobic at 25 ◦ C and 37 ◦ C, respectively. As shown from Fig. 5, the green fluo contraction of isopropyl, thus, PA is wrapped in the core of Cs-Cys-PN rescence was very weak and hardly observed at 25 ◦ C, however, with the micelles, which restricting of intramolecular molecular (RIM) motion temperature increased, enhanced emission of green fluorescence of PA, thus, the enhanced emission of Cs-Cys-PN/PA is found. Moreover, increased significantly. The reason of the enhanced emission of green as shown from Fig. 3B and C, which reveals the change of the fluorescent fluorescence was that the cellular activity of cellular uptake PA higher at intensity of Cs-Cys-PN/PA hydrogel with temperature. In a solution at 37 ◦ C than at 25 ◦ C, the enhanced cellular uptake of PA at 37 ◦ C. In 24 ◦ C, pH = 7.4, PNIPAM shown the block with hydrophilic. Since the addition, when the temperature was higher than the LCST of Cs-Cys-PN/ PA, the PNIPAM chains gradually converted hydrophilic to 321 L. Xu et al. International Journal of Biological Macromolecules 189 (2021) 316–323 Fig. 7. SEM images of S. aureus bacterial morphology with (A) culture medium and (B) 0.05 μmol/mL PA, (C) 0.05 μmol/mL of Cs-Cys-PN/PA. hydrophobic, which wrapping more PA in the core of Cs-Cys-PN, not exhibits a single-color AIEE active reversible response function. Due to only providing a non-polar microenvironment for PA but also restricting PA molecule is encapsulated in Cs-Cys-PN, as the temperature increases, of intramolecular molecular motion (RIM) of PA, resulting in AIEE it not only restricts of intramolecular molecular (RIM) motion of PA but behavior of fluorescence. also provides PA with a non-polar microenvironment, achieving the In order to study the antibacterial properties of Cs-Cys-PN/PA, in effect of fluorescence enhancement. Due to its responsive fluorescence vitro antibacterial experiment of S. aureus was investigated by the enhancement and low cytotoxicity, the Cs-Cys-PN/PA can be coopera method of standard plate counting. As shown from Fig. 6A, it is worth tively applied to cell imaging and can distinguish the change in tem mentioning that under low concentration of (0.0125–0.025 μmol/mL), perature. Moreover, Cs-Cys-PN/PA has a more ideal effect in slowing the PA, Cs-Cys-PN/PA shown low antibacterial effect than the concentration release of PA and inhibiting the growth of S. aureus. Therefore, Cs-Cys- of 0.05 μmol/mL of PA, Cs-Cys-PN/PA. Cs-Cys-PN/PA has distinctly PN/PA has potential applications in temperature sensors and antibac bacteriostatic properties with S. aureus contrasted to PA (P < 0.05), terial field. particularly, when the concentration was 0.05 μmol/mL, the inhibition ratio of PA against S. aureus was 61.20 ± 2.00%, and the inhibition rate CRediT authorship contribution statement of Cs-Cys-PN/PA was 86.97 ± 6.00%, Cs-Cys-PN/PA shown higher antibacterial rate. However, under (0.1–0.2 μmol/mL), due to the Lifeng Xu: Data curation, Writing- Original draft preparation. S. aureus has certain resistance, thus, PA, Cs-Cys-PN/PA shown low Xiao Liang: Data curation, Visualization, Investigation. antibacterial rate than the concentration of 0.05 μmol/mL of PA, Cs-Cys- Liru You: Visualization, Investigation. PN/PA. Therefore, 0.05 μmol/mL is the best inhibitory concentration of Yongyan Yang: Supervision. Cs-Cys-PN/PA. Fig. 6B shown that the photos of S. aureus under 0.05 Gangying Feng: Conceptualization, Methodology, Software. μmol/mL, it is obvious that Cs-Cys-PN/PA has better inhibition effect Yan Gao: Supervision. than PA. Xuejun Cui: Writing- Reviewing and Editing. To further study the antibacterial properties of Cs-Cys-PN/PA, SEM was used to investigate the morphology of S. aureus. As shown from Acknowledgments Fig. 7A, standard S. aureus is round and the smooth which the diameter is probably 800 nm. In the Fig. 7B, comparing with normal S aureus, the This work was supported by the Natural Science Foundation of the S aureus of PA is no longer standard spherical. We observed wilting and Department of Science and Technology of Jilin Province, PR China (No. irregularly broken bacteria in rough surfaces. As shown from Fig. 7C, 20180101072JC), the Key Program of the Science and Technology compared with PA, the S aureus of Cs-Cys-PN/PA has more severe Department of Jilin Province, PR China (No. 20180201082SF) and the damages of shrink and rupture irregularly, and the surface of the bac scientific research Program of the Education Department of Jilin Prov teria obviously become rough and collapsed. Moreover, the morphology ince (P. R. China) during the 13th Five-Year Plan Period (No. of the S aureus became elliptical. As we all know, the protonated JJKH20180119KJ),Interdisciplinary Research Funding Program for ammonium on chitosan has an antibacterial effect, it can adsorb and Doctoral Students of Jilin University (No. 101832020DJX026). The accumulate bacteria, penetrate the cell wall, and interfere with meta authors are thanks for the funding. bolism. Therefore, Cs-Cys-PN has the inhibitory effect on S aureus. Since the PNIPAM block in Cs-Cys-PN/PA starts to be hydrophobic and col Appendix A. Supplementary data lapses to form micelles, which can wrap more PA in the core of micelles, and show a stable sustained release of PA for more effective inhibition Supplementary data to this article can be found online at https://doi. for the growth of S. aureus. Therefore, Cs-Cys-PN/PA can be aggregation org/10.1016/j.ijbiomac.2021.08.057. and attachment of S. aureus which causes blurred cytoplasmic mem brane boundaries, lysis of bacterial cells and loss of internal structure. References Thus, Cs-Cys-PN/PA has dual antibacterial properties in inhibiting the growth of S. aureus. L. Xu, S. Zhang, X. Liang, S. Zhong, B. Wang, Z. Li, X. Cui, Novel biocompatible AIEgen from natural resources: palmatine and its bioimaging application, Dyes Pigments 184 (2021), 108860. 4. Conclusion A.K. Sharma, B.S.Kaith Priya, A.Singh Isha, K. Chandel, Vipula, riboflavin functionalized dextrin-sodium alginate based fluorescent sensor: detoxification of In short, a temperature-sensitive copolymer containing AIEE fluo Cu2 and Ni2 ions, ACS Appl. Polym. Mater. (2019) 3084–3094. J. Qi, C. Sun, D. Li, H. Zhang, W. Yu, A. Zebibula, J.W.Y. Lam, W. Xi, L. Zhu, F. Cai, rophore was prepared by the way of RAFT polymerization of PNIPAM P. Wei, C. Zhu, R.T.K. Kwok, L.L. Streich, R. Prevedel, J. Qian, B.Z. Tang, and chitosan. When the external temperature changes, the polymer Aggregation-induced emission luminogen with near-infrared-II excitation and 322 L. Xu et al. International Journal of Biological Macromolecules 189 (2021) 316–323 near-infrared-I emission for ultradeep intravital two-photon microscopy, ACS Nano S. Ekici, P. Ilgin, S. Yilmaz, N. Aktas, N. Sahiner, Temperature and magnetic field 12 (2018) 7936–7945. responsive hyaluronic acid particles with tunable physical and chemical properties, S. Wang, F. Hu, Y. Pan, L.G. Ng, B. Liu, Bright AIEgen–Protein hybrid Appl. Surf. Sci. 257 (2011) 2669–2676. nanocomposite for deep and high-resolution in vivo two-photon brain imaging, Q.X. Hua, B. Xin, J.X. Liu, L.X. Zhao, Z.J. Xiong, T. Chen, Z.Q. Chen, C. Li, W. Adv. Funct. Mater. 29 (2019) 1902717. L. Gong, Z.L. Huang, M.Q. Zhu, Bulky 4,6-disubstituted tetraphenylethene- R. Long, C. Tang, J. Xu, T. Li, C. Tong, Y. Guo, S. Shi, D. Wang, Novel natural naphthalimide dyad: synthesis, copolymerization, stimuli-responsive fluorescence myricetin with AIE and ESIPT characteristics for selective detection and imaging of and cellular imaging, Faraday Discuss. 196 (2017) 439–454. superoxide anions in vitro and in vivo, Chem. Commun. (Camb.) 55 (2019) A.S. Montaser, M. Rehan, M.E. El-Naggar, pH-thermosensitive hydrogel based on 10912–10915. polyvinyl alcohol/sodium alginate/N-isopropyl acrylamide composite for treating L. Xu, J. Cao, S. Zhong, J. Wang, Y. Yang, Y. Gao, X. Cui, Photoluminescence of re-infected wounds, Int. J. Biol. Macromol. 124 (2019) 1016–1024. tilapia skin collagen: aggregation-induced emission with clustering triggered Z. Zhang, N. Hadjichristidis, Temperature and pH-dual responsive AIE-active Core emission mechanism and its multiple applications, Int. J. Biol. Macromol. 182 crosslinked Polyethylene–Poly(methacrylic acid) multimiktoarm star copolymers, (2021) 1437–1444. ACS Macro Lett. 7 (2018) 886–891. J. Luo, Z. Xie, J.W. Lam, L. Cheng, H. Chen, C. Qiu, H.S. Kwok, X. Zhan, Y. Liu, H. Ma, C. Qi, C. Cheng, Z. Yang, H. Cao, Z. Yang, J. Tong, X. Yao, Z. Lei, AIE-active D. Zhu, B.Z. Tang, Aggregation-induced emission of 1-methyl-1,2,3,4,5- tetraphenylethylene cross-linked N-isopropylacrylamide polymer: a long-term pentaphenylsilole, Chem. Commun. (Camb.) 1740–1741 (2001). fluorescent cellular tracker, ACS Appl. Mater. Interfaces 8 (2016) 8341–8348. L. Xu, X. Liang, S. Zhang, B. Wang, S. Zhong, M. Wang, X. Cui, Riboflavin: a natural Y. Zhao, Y. Wu, S. Chen, H. Deng, X. Zhu, Building single-color AIE-active aggregation-induced emission luminogen (AIEgen) with excited-state proton reversible micelles to interpret temperature and pH stimuli in both solutions and transfer process for bioimaging, Dyes Pigments 182 (2020), 108642. cells, Macromolecules 51 (2018) 5234–5244. L. Shi, Y.H. Liu, K. Li, A. Sharma, K.K. Yu, M.S. Ji, L.L. Li, Q. Zhou, H. Zhang, J. Z. Dong, H. Cui, Y. Wang, C. Wang, Y. Li, C. Wang, Biocompatible AIE material S. Kim, X.Q. Yu, An AIE-based probe for rapid and ultrasensitive imaging of plasma from natural resources: chitosan and its multifunctional applications, Carbohydr. membranes in biosystems, Angew. Chem. Int. Ed. 59 (2020) 9962–9966. Polym. 227 (2020), 115338. C. Hu, Y. Ru, Z. Guo, Z. Liu, J. Song, W. Song, X. Zhang, J. Qiao, New multicolored M.T. Pelegrino, J.C. Pieretti, G. Nakazato, M.C. Goncalves, J.C. Moreira, A. AIE photoluminescent polymers prepared by controlling the pH value, J. Mater. B. Seabra, Chitosan chemically modified to deliver nitric oxide with high Chem. C 7 (2019) 387–393. antibacterial activity, Nitric Oxide 106 (2021) 24–34. M.M.S. Lee, L. Zheng, B. Yu, W. Xu, R.T.K. Kwok, J.W.Y. Lam, F. Xu, D. Wang, B. X. Wang, C. Zheng, Z. Wu, D. Teng, X. Zhang, Z. Wang, C. Li, Chitosan-NAC Z. Tang, A highly efficient and AIE-active theranostic agent from natural herbs, nanoparticles as a vehicle for nasal absorption enhancement of insulin, J Biomed Mater. Chem. Front. 3 (2019) 1454–1461. Mater Res B Appl Biomater 88 (2009) 150–161. Y. Gu, Z. Zhao, H. Su, P. Zhang, J. Liu, G. Niu, S. Li, Z. Wang, R.T.K. Kwok, X.L. Ni, J. Huang, Y.L. Wang, X.D. Yu, Y.N. Zhou, L.Q. Chu, Enhanced fluorescence of J. Sun, A. Qin, J.W.Y. Lam, B.Z. Tang, Exploration of biocompatible AIEgens from carboxymethyl chitosan via metal ion complexation in both solution and hydrogel natural resources, Chem. Sci. 9 (2018) 6497–6502. states, Int. J. Biol. Macromol. 152 (2020) 50–56. K.G. Lyamzaev, A.V. Pustovidko, R.A. Simonyan, T.I. Rokitskaya, L.V. Domnina, O. S. Zhao, Z. Li, Z. Zhou, L. Xu, S. He, Y. Dou, X. Cui, S. Kang, Y. Gao, Y. Wang, Y. Ivanova, N.V. Severina II, G.A. Sumbatyan, V.N. Korshunova, V.A. Tashlitsky, Y. Antifungal activity of water-soluble products obtained following the liquefaction of N. Roginsky, M.V. Antonenko, B.V. Skulachev, V.P.Skulachev Chernyak, Novel cornstalk with sub-critical water, Pestic. Biochem. Physiol. 163 (2020) 263–270. mitochondria-targeted antioxidants: plastoquinone conjugated with cationic plant L. Xu, S. Zhong, C. Shi, Y. Sun, S. Zhao, Y. Gao, X. Cui, Sonochemical fabrication of alkaloids berberine and palmatine, Pharm. Res. 28 (2011) 2883–2895. reduction-responsive magnetic starch-based microcapsules, Ultrason. Sonochem. L. Xu, Y. Zhang, S. Wang, H. Hu, S. Zhong, S. He, Y. Dou, Z. Li, X. Cui, 49 (2018) 169–174. Thermoresponsive gel for sustained release of BMP4 to inhibit corneal S. He, S. Zhong, L. Xu, Y. Dou, Z. Li, F. Qiao, Y. Gao, X. Cui, Sonochemical neovascularization, Colloids Surf. B: Biointerfaces 194 (2020), 111167. fabrication of magnetic reduction-responsive alginate-based microcapsules for T. Li, S. He, J. Qu, H. Wu, S. Wu, Z. Zhao, A. Qin, R. Hu, B.Z. Tang, drug delivery, Int. J. Biol. Macromol. 155 (2020) 42–49. Thermoresponsive AIE polymers with fine-tuned response temperature, J. Mater. W.L.Shujun Sun, Nan Cheng, Bingqi Zhang, Zhiqiang Cao, Kangde Yao, A.Z. Chem. C 4 (2016) 2964–2970. Dongchun Liang, Gang Guo, Jingyu Zhang, A thermoresponsive chitosan-NIPAAm/ Z. Wang, T.Y. Yong, J. Wan, Z.H. Li, H. Zhao, Y. Zhao, L. Gan, X.L. Yang, H.B. Xu, vinyl laurate copolymer vector for gene transfection, Bioconjug. Chem. 16 (2005) C. Zhang, Temperature-sensitive fluorescent organic nanoparticles with 972–980. aggregation-induced emission for long-term cellular tracing, ACS Appl. Mater. T. Li, P. Wang, W. Guo, X. Huang, X. Tian, G. Wu, B. Xu, F. Li, C. Yan, X.J. Liang, Interfaces 7 (2015) 3420–3425. H. Lei, Natural berberine-based chinese herb medicine assembled nanostructures with modified antibacterial application, ACS Nano 13 (2019) 6770–6781. 323