1. Introduction to Aircraft Structures.pdf
Document Details
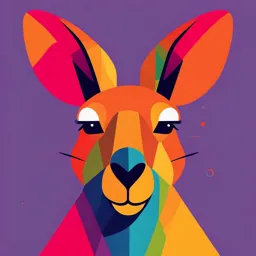
Uploaded by QuickerKelpie3864
Tags
Full Transcript
INTRODUCTION TO AIRCRAFT STRUCTURES AIRCRAFT STRUCTURES 1 PREPARED BY: ENGR. AMOS MATTHEW S. PORTUGAL AIRCRAFT STRUCTURAL DESIGNS AND SYSTEMS The full understanding of both the terminology in statics and the fundamental principles of mechanics is an essential prerequisite to the analysis and d...
INTRODUCTION TO AIRCRAFT STRUCTURES AIRCRAFT STRUCTURES 1 PREPARED BY: ENGR. AMOS MATTHEW S. PORTUGAL AIRCRAFT STRUCTURAL DESIGNS AND SYSTEMS The full understanding of both the terminology in statics and the fundamental principles of mechanics is an essential prerequisite to the analysis and design of structures. Therefore, this lesson is devoted to the presentation and the application of these fundamentals. Any deformable solid body which is capable of carrying loads and transmitting these loads to other parts of the body is referred to as a structural system. The constituents of such systems are beams, plates, shells, or a combination of the three. BAR ELEMENTS Bar elements, such as shown in the figure, are one dimensional structural members which are capable of carrying and transmitting bending, shearing, torsional, and axial loads or a combination of all four. Bars which are capable of carrying only axial General bar loads are referred to as axial rods or two force members. Structural systems constructed entirely out of axial rods are called trusses and frequently are Axial rod used in many atmospheric, sea, and land-based structures, since simple tension or compression members are usually the lightest for transmitting forces. Torsion member PLATE ELEMENTS Plate elements, such as shown in the figure, are two- dimensional extensions of bar elements. Plates made to carry only in-plane axial loads are called General plate membranes. Those which are capable of carrying only in-plane shearing loads are referred to as shear panels; frequently these are found in missile fins, aircraft wings, and tail surfaces. Membrane element Shear panel SHELL ELEMENTS Shells are curved plate elements which occupy a space. Fuselages, building domes, pressure vessels, etc., are typical examples of shells. BENDING MEMBER A structural member that can carry bending moments is called a beam. A beam can also act as an axial member carrying longitudinal tension and compression. TORSION MEMBER Torque is an important form of load to aircraft structures. In a structural member, torque is formed by shear stresses acting in the plane of the cross-section. A member subjected to torsional moments would twist about a longitudinal axis through the shear center of the cross section. MAJOR STRUCTURAL STRESSES Aircraft structural members are designed to carry a load or to resist stress. In designing an aircraft, every square inch of wing and fuselage, every rib, spar, and even each metal fitting must be considered in relation to the physical characteristics of the material of which it is made. Every part of the aircraft must be planned to carry the load to be imposed upon it. The term stress is often used interchangeably with the word strain. While related, they are not the same thing. External loads or forces cause stress. Stress is a material’s internal resistance, or counterforce, that opposes deformation. The degree of deformation of a material is strain. When a material is subjected to a load or force, that material is deformed, regardless of how strong the material is or how light the load is. MAJOR STRUCTURAL STRESSES There are five major stresses (shown in the figure) to which all aircraft are subjected: Tension Compression Torsion Shear Bending MAJOR STRUCTURAL STRESSES Tension is the stress that resists a force that tends to pull something apart. It is the stress of stretching an object or pulling at its ends. Tension is the resistance to pulling apart or stretching produced by two forces pulling in opposite directions along the same straight line. For example, an elevator control cable is in additional tension when the pilot moves the control column. MAJOR STRUCTURAL STRESSES Compression is the resistance to crushing produced by two forces pushing toward each other in the same straight line. It tends to shorten or squeeze aircraft parts. For example, when an airplane is on the ground, the landing gear struts are under a constant compression stress. MAJOR STRUCTURAL STRESSES Torsion is the stress that produces twisting. While moving the aircraft forward, the engine also tends to twist it to one side, but other aircraft components hold it on course. Thus, torsion is created. The torsion strength of a material is its resistance to twisting or torque. MAJOR STRUCTURAL STRESSES Shear is the stress that resists the force tending to cause one layer of a material to slide over an adjacent layer. Two riveted plates in tension subject the rivets to a shearing force. Usually, the shearing strength of a material is either equal to or less than its tensile or compressive strength. Aircraft parts, especially screws, bolts, and rivets, are often subject to a shearing force. MAJOR STRUCTURAL STRESSES Bending stress is a combination of compression and tension. The rod in has been shortened (compressed) on the inside of the bend and stretched on the outside of the bend. A single member of the structure may be subjected to a combination of stresses. In most cases, the structural members are designed to carry end loads rather than side loads. They are designed to be subjected to tension or compression rather than bending. GENERAL LOAD CLASSIFICATION Loads which act on a structural system may be generally classified in accordance with their causes. Those which are produced by surface contact are called surface loads. Dynamic and/or static pressures are examples of surface loads. If the area of contact is very small, then the load is said to be concentrated; otherwise, it is called a distributed load. GENERAL LOAD CLASSIFICATION Loads which depend on body volume are called body loads. Inertial, magnetic, and gravitational forces are typical examples. Generally, these loads are assumed to be distributed over the entire volume of the body. Most of the loads resisted by structural elements are due to aircraft weight (actually the aircraft’s mass) and the fact that the airplane accelerates during flight. Inertia loads are a primary source of loads for aircraft and arise because of accelerations created by engine thrust, maneuvers or gust conditions. The concept of the load factor or g-force is used to describe inertia loads created by acceleration and resisted by the structural components to keep it acting as a unit. GENERAL LOAD CLASSIFICATION Dynamic loads are time dependent, whereas static loads are independent of time. GENERAL LOAD CLASSIFICATION Thermal loads are created on a restrained structure by a uniform and/or non-uniform temperature change. Regardless of the classification of the externally imposed loads, a structural member, in general resists these loads internally in the form of bending, axial, shear, and torsional actions or a combination of the four. WING STRUCTURE The main function of the wing is to pick up the air loads and transmit them to the fuselage. The wing cross-section takes the shape of an airfoil, which is designed based on aerodynamic considerations. The wing as a whole performs the combined function of a beam and a torsion member. It consists of axial members in stringers, bending members in spars and shear panels in the cover skin and webs of spars. WING SPAR The spar is a heavy beam running spanwise to take transverse shear loads and spanwise bending. They run parallel to the lateral axis of the aircraft, from the fuselage toward the tip of the wing, and are usually attached to the fuselage by wing fittings, plain beams, or a truss. As a rule, a wing has two spars: one spar is usually located near the front of the wing, and the other about two-thirds of the distance toward the wing’s trailing edge. When other structural members of the wing are placed under load, most of the resulting stress is passed on to the wing spar. WING SPAR In an I–beam spar, the top and bottom of the I– beam are called the caps and the vertical section is called the web. The web forms the principal depth portion of the spar and the cap strips (extrusions, formed angles, or milled sections) are attached to it. Together, these members carry the loads caused by wing bending, with the caps providing a foundation for attaching the skin. WING SPAR Additionally, fail-safe spar web design exists. Fail-safe means that should one member of a complex structure fail, some other part of the structure assumes the load of the failed member and permits continued operation. The spar (in figure) is made in two sections. The top section consists of a cap riveted to the upper web plate. The lower section is a single extrusion consisting of the lower cap and web plate. These two sections are spliced together to form the spar. If either section of this type of spar breaks, the other section can still carry the load. This is the fail-safe feature. WING RIB Wing ribs are planar structures capable of carrying in-plane loads. They are placed chordwise along the wing span. Besides serving as load redistributors, ribs also hold the skin stringer to the designed contour shape. Ribs reduce the effective buckling length of the stringers (or the stringer-skin system) and thus increase their compressive load capability. Note that the rib is supported by spanwise spars. WING SKIN Often, the skin on a wing is designed to carry part of the flight and ground loads in combination with the spars and ribs. This is known as a stressed-skin design. Fuel is often carried inside the wings of a stressed-skin aircraft. The joints in the wing can be sealed with a special fuel resistant sealant enabling fuel to be stored directly inside the structure. This is known as wet wing design. WING SKIN On aircraft with stressed-skin wing design, honeycomb structured wing panels are often used as skin. A honeycomb structure is built up from a core material resembling a bee hive’s honeycomb which is laminated or sandwiched between thin outer skin sheets. Panels formed like honeycomb are lightweight and very strong. WING CONSTRUCTION In general, wing construction is based on one of three fundamental designs: monospar, multispar, and box-beam. The monospar wing incorporates only one main spanwise or longitudinal member in its construction. Ribs or bulkheads supply the necessary contour or shape to the airfoil. Although the strict monospar wing is not common, this type of design modified by the addition of false spars or light shear webs along the trailing edge for support of control surfaces is sometimes used. WING CONSTRUCTION The multispar wing incorporates more than one main longitudinal member in its construction. To give the wing contour, ribs or bulkheads are often included. WING CONSTRUCTION The box beam type of wing construction uses two main longitudinal members with connecting bulkheads to furnish additional strength and to give contour to the wing. A corrugated sheet may be placed between the bulkheads and the smooth outer skin so that the wing can better carry tension and compression loads. Air transport category aircraft often utilize box beam wing construction. WING ATTACHMENT WING ATTACHMENT Often wings are of full cantilever design. This means they are built so that no external bracing is needed. They are supported internally by structural members assisted by the skin of the aircraft. The semi-cantilever usually has one, or perhaps two, supporting wires or struts attached to each wing and the fuselage. Other aircraft wings use external struts or wires to assist in supporting the wing and carrying the aerodynamic and landing loads. Wing support cables and struts are generally made from steel. Many struts and their attach fittings have fairings to reduce drag. Short, nearly vertical supports called jury struts are found on struts that attach to the wings a great distance from the fuselage. This serves to subdue strut movement and oscillation caused by the air flowing around the strut in flight. FUSELAGE STRUCTURE The fuselage is the main structure or body of the fixed-wing aircraft. It provides space for cargo, controls, accessories, passengers, and other equipment. In single-engine aircraft, the fuselage houses the powerplant. In multiengine aircraft, the engines may be either in the fuselage, attached to the fuselage, or suspended from the wing structure. There are two general types of fuselage construction: truss and monocoque. FUSELAGE STRUCTURE A truss is a rigid framework made up of members, such as beams, struts, and bars to resist deformation by applied loads. The truss-framed fuselage is generally covered with fabric, and is usually constructed of steel tubing welded together in such a manner that all members of the truss can carry both tension and compression loads. In some aircraft, principally the light, single engine models, truss fuselage frames may be constructed of aluminum alloy and may be riveted or bolted into one piece, with cross-bracing achieved by using solid rods or tubes. FUSELAGE STRUCTURE The monocoque (single shell) fuselage relies largely on the strength of the skin or covering to carry the primary loads. The true monocoque construction uses formers, frame assemblies, and bulkheads to give shape to the fuselage. The heaviest of these structural members are located at intervals to carry concentrated loads and at points where fittings are used to attach other units such as wings, powerplants, and stabilizers. Since no other bracing members are present, the skin must carry the primary stresses and keep the fuselage rigid. Thus, the biggest problem involved in monocoque construction is maintaining enough strength while keeping the weight within allowable limits. FUSELAGE STRUCTURE To overcome the strength/weight problem of monocoque construction, a modification called semi- monocoque construction was developed. It also consists of frame assemblies, bulkheads, and formers as used in the monocoque design but, additionally, the skin is reinforced by longitudinal members called longerons, which usually extend across several frame members and help the skin support primary bending loads. FUSELAGE STRUCTURE Stringers are also used in the semi-monocoque fuselage. These longitudinal members are typically more numerous and lighter in weight than the longerons. Stringers have some rigidity but are chiefly used for giving shape and for attachment of the skin. Stringers and longerons together prevent tension and compression from bending the fuselage. FUSELAGE STRUCTURE The advantages of the semi-monocoque fuselage are many. The bulkheads, frames, stringers, and longerons facilitate the design and construction of a streamlined fuselage that is both rigid and strong. Spreading loads among these structures and the skin means no single piece is failure critical. This means that a semi-monocoque fuselage, because of its stressed-skin construction, may withstand considerable damage and still be strong enough to hold together. FUSELAGE STRUCTURE The fuselage will see a combination of loads from multiple sources during a typical flight. Large bending loads are introduced from the wing and tail sections, as well as torsional loads from the pitching moment of the wing. The fuselage generates its own aerodynamic loads during flight which must be reacted by the structure. These external pressure loads combine with internal pressure loads if the aircraft is pressurized. Landing loads introduced to the fuselage can be particularly severe if the landing is executed poorly. NACELLES Nacelles (sometimes called pods) are streamlined enclosures used primarily to house the engine and its components. They usually present a round or elliptical profile to the wind thus reducing aerodynamic drag. A nacelle contains the engine and accessories, engine mounts, structural members, a firewall, and skin and cowling on the exterior to fare the nacelle to the wind. NACELLES Cowling refers to the detachable panels covering those areas into which access must be gained regularly, such as the engine and its accessories. It is designed to provide a smooth airflow over the nacelle and to protect the engine from damage. Cowl flaps are moveable parts of the nacelle cowling that open and close to regulate engine temperature. EMPENNAGE The empennage of an aircraft is also known as the tail section. Most empennage designs consist of a tail cone, fixed aerodynamic surfaces or stabilizers, and movable aerodynamic surfaces. The tail cone serves to close and streamline the aft end of most fuselages. The cone is made up of structural members like those of the fuselage; however, cones are usually of lighter construction since they receive less stress than the fuselage. EMPENNAGE The structure of the stabilizers is very similar to that which is used in wing construction. Notice the use of spars, ribs, stringers, and skin like those found in a wing. They perform the same functions shaping and supporting the stabilizer and transferring stresses. Bending, torsion, and shear created by air loads in flight pass from one structural member to another. Each member absorbs some of the stress and passes the remainder on to the others. Ultimately, the spar transmits any overloads to the fuselage. LANDING GEAR The purpose of the landing gear in an aircraft is to provide a suspension system during taxi, take-off, and landing. It is designed to absorb and dissipate the force of a landing impact which in turn reduces the stress on the airframe. The landing gear also facilitates the braking system of the aircraft and provides directional control via a steering system. The main structural components of a landing gear system are the shock absorber, axle, torque links, side braces, retraction actuators, wheels, and tires. GENERAL LOAD CONDITIONS To ensure safety, structural integrity, and reliability of flight vehicles along with the optimality of design, government agencies, both civil and military, have established definite specifications and requirements in regard to the magnitude of the loads to be used in structural design of various flight vehicles. Terms are defined in the following slides which are generally used in the specification of loads on flight vehicles. GENERAL LOAD CONDITIONS The limit loads used by civil agencies or applied loads used by military agencies are the maximum anticipated loads in the entire service lifespan of the vehicle. The ultimate loads, commonly referred to as design loads, are the limit loads multiplied by a factor of safety (FS): ultimate load FS = limit load Generally, a factor of safety which varies from 1.25 for missile structures to 1.5 for aircraft structures is used in practically every design because of the uncertainties involving: The simplifying assumption used in theoretical analyses The variations in material properties and in the standards of quality control The emergency actions which might have to be taken by the pilot, resulting in loads on the vehicle larger than the specified limit loads GENERAL LOAD CONDITIONS The limit loads and ultimate loads quite often are prescribed by specifying certain load factors. The limit load factor is a factor by which basic loads on a vehicle are multiplied to obtain the limit loads. The ultimate load factor by which basic loads on a vehicle are multiplied to obtain the ultimate loads. In other words, the ultimate load factor is the product of the limit load factor and the factor of safety. STRESS-STRAIN DIAGRAM Suppose that a metal specimen be placed in tension-compression-testing machine. As the axial load is gradually increased in increments, the total elongation over the gauge length is measured at each increment of the load and this is continued until failure of the specimen takes place. Knowing the original cross-sectional area and length of the specimen, the normal stress σ and the strain ε can be obtained. The graph of these quantities with the stress 𝜎 along the 𝑦-axis and the strain 𝜀 along the 𝑥-axis is called the stress-strain diagram. STRESS-STRAIN DIAGRAM From the origin to the point called proportional limit, the stress-strain curve is a straight line. This linear relation between elongation and the axial force causing is called Hooke's Law that within the proportional limit, the stress is directly proportional to strain. The elastic limit is the limit beyond which the material will no longer go back to its original shape when the load is removed, or it is the maximum stress that may be developed such that there is no permanent or residual deformation when the load is entirely removed. STRESS-STRAIN DIAGRAM The region in stress-strain diagram from the origin to the elastic limit is called the elastic range. The region to the right of the elastic range is called the plastic range. A slight increase in stress above the elastic limit will result in a breakdown of the material and cause it to deform permanently. This behavior is called yielding, and it is indicated by the rectangular dark orange region. STRESS-STRAIN DIAGRAM The stress that causes yielding is called the yield stress or yield point, and the deformation that occurs is called plastic deformation. Once the yield point is reached, the specimen will continue to elongate (strain) without any increase in load. When the material behaves in this manner, it is often referred to as being perfectly plastic. STRESS-STRAIN DIAGRAM When yielding has ended, any load causing an increase in stress will be supported by the specimen, resulting in a curve that rises continuously but becomes flatter until it reaches a maximum stress referred to as the ultimate stress. The rise in the curve in this manner is called strain hardening, and it is identified as the region in light green. STRESS-STRAIN DIAGRAM Up to the ultimate stress, as the specimen elongates, its cross-sectional area will decrease in a fairly uniform manner over the specimen’s entire gage length. However, just after reaching the ultimate stress, the cross-sectional area will then begin to decrease in a localized region of the specimen, and so it is here where the stress begins to increase. As a result, a constriction or neck tends to form with further elongation. This region of the curve due to necking is indicated in dark green. STRESS-STRAIN DIAGRAM Here the stress–strain diagram tends to curve downward until the specimen breaks at the fracture stress. Instead of always using the original cross- sectional area 𝐴0 and specimen length 𝐿0 to calculate the stress and strain, we could have used the actual cross-sectional area 𝐴 and specimen length 𝐿 at the instant the load is measured. The values of stress and strain found from these measurements are called true stress and true strain, and a plot of their values is called the true stress– strain diagram. STRESS-STRAIN DIAGRAM When this diagram is plotted, it has a form shown by the upper blue curve. From the conventional stress-strain diagram, the specimen appears to support a decreasing stress (or load), since 𝐴0 is constant, 𝜎 = 𝑁/𝐴0. In fact, the true stress-strain diagram shows the area 𝐴 within the necking region is always decreasing until fracture, and so the material actually sustains increasing stress, since 𝜎 = 𝑁/𝐴. ALLOWABLE STRESS Working stress is defined as the actual stress of a material under a given loading. The maximum safe stress that a material can carry is termed as the allowable stress. The allowable stress should be limited to values not exceeding the proportional limit. However, since proportional limit is difficult to determine accurately, the allowable stress is taken as either the yield point or ultimate strength divided by a factor of safety. The ratio of this strength (ultimate or yield strength) to allowable strength is called the factor of safety.