Reprogramming Human Fibroblasts to Pluripotent Stem Cells (2010) PDF
Document Details
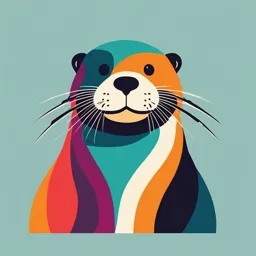
Uploaded by MeaningfulVampire5959
Arizona State University
2010
Eduard Yakubov, Gidi Rechavi, Shmuel Rozenblatt, David Givol
Tags
Summary
This research article details a method for reprogramming human fibroblasts into pluripotent stem cells using mRNA encoding four transcription factors. This approach avoids DNA integration typically associated with other methods. Using RNA transfection results in protein expression, and the development of iPS cells, demonstrating successful reprogramming.
Full Transcript
Biochemical and Biophysical Research Communications 394 (2010) 189–193 Contents lists available at ScienceDirect Biochemical and Biophysical Research Communications...
Biochemical and Biophysical Research Communications 394 (2010) 189–193 Contents lists available at ScienceDirect Biochemical and Biophysical Research Communications journal homepage: www.elsevier.com/locate/ybbrc Reprogramming of human fibroblasts to pluripotent stem cells using mRNA of four transcription factors Eduard Yakubov a, Gidi Rechavi b, Shmuel Rozenblatt c, David Givol a,* a Department of Molecular Cell Biology, Weizmann Institute of Science, 76100 Rehovot, Israel b Cancer Research Center, Chaim Sheba Medical Center, Tel-Hashomer and Sackler School of Medicine, Tel-Aviv University, Tel-Aviv, Israel c Department of Molecular Microbiology and Biotechnology, Tel-Aviv University, Tel-Aviv, Israel a r t i c l e i n f o a b s t r a c t Article history: Reprogramming of differentiated cells into induced pluripotent cells (iPS) was accomplished in 2006 by Received 21 February 2010 expressing four, or less, embryonic stem cell (ESC)-specific transcription factors. Due to the possible dan- Available online 25 February 2010 ger of DNA damage and the potential tumorigenicity associated with such DNA damage, attempts were made to minimize DNA integration by the vectors involved in this process without complete success. Keywords: Here we present a method of using RNA transfection as a tool for reprogramming human fibroblasts to Reprogramming iPS. We used RNA synthesized in vitro from cDNA of the same reprogramming four transcription factors. iPS After transfection of the RNA, we show intracellular expression and nuclear localization of the respective DNA integration Malignancy proteins in at least 70% of the cells. We used five consecutive transfections to support continuous protein RNA transfection expression resulting in the formation of iPS colonies that express alkaline phosphatase and several ESC Transcription factors markers and that can be expanded. This method completely avoids DNA integration and may be devel- oped to replace the use of DNA vectors in the formation of iPS. Ó 2010 Elsevier Inc. All rights reserved. 1. Introduction quences remained at the integration site as a potential damaging ele- ment. Hence the danger of DNA damage was not eliminated Induced pluripotent stem cells (iPS) are produced by reprogram- completely from the procedures using DNA vectors. ming of somatic cells and are very similar to natural embryonic stem We believe that avoiding DNA in the reprogramming process cells (ESC), showing the capacity to differentiate into any cell type might be a necessary solution to the problem of genome integra- and the ability to self renew. Potentially they hold great hope for per- tion, providing that it does not prevent the production of iPS. Re- sonal regenerative medicine, cell therapy and disease models in cently the use of recombinant proteins of the same four factors, addition to their value in the study of the process of cell fate determi- produced in bacteria, was reported with successful generation of nation. Since the pioneering work of S. Yamanka in 2006, iPS cells PiPS (Protein-produced iPS) cells from mouse fibroblasts. have been generated from a variety of somatic cell types by the However proper post translation modification of the proteins is transfection of DNA encoding four, or fewer, transcription factors better processed inside the cell and not in bacteria. (TF), Oct4, Sox2, Klf4, and c-Myc. In human iPS cells, Lin28 and Here we report the use of in vitro-produced mRNA encoding for Nanog replaced Klf4 and Myc. DNA was transduced into somatic Oct4, Lin28, Sox2, and Nanog for reprogramming of human fore- cells by retroviral or lentiviral infection, by plasmids, or by other vec- skin fibroblasts (hFF) to generate RiPS (RNA-produced iPS) cells. tors [3–5]. iPS cells were obtained by various alternative methods of This procedure avoids any DNA integration events and the associ- DNA insertions into cells, all of which resulted in multiple DNA inte- ated genomic damage. RNA transfection resulted in protein expres- gration sites in the genome of the generated iPS cells. Based on pre- sion level in less than eight hours and at least five consecutive vious experience, particularly from gene therapy, it became obvious transfections were needed to maintain protein expression levels that genome integration by DNA is unpredictable and may lead to that could switch-on the expression of endogenous stem cell plu- malignancy and other undesired results. Therefore attempts were ripotent genes that drive the human fibroblasts to iPS. made to minimize genome integration by combining the DNA of all four factors into one polycistronic vector [6,7] and more recently 2. Materials and methods by Cre-loxP excision of the inserted genes after the reprogramming process was switched-on [5,8]. However some vector arms se- 2.1. Cell culture, cells transfection * Corresponding author. Fax: +972 89344125. Human foreskin fibroblasts (hFF, Sigma) were cultured on gela- E-mail address: [email protected] (D. Givol). tin-coated dishes (100,000 cells per well of six-well plate) with hFF 0006-291X/$ - see front matter Ó 2010 Elsevier Inc. All rights reserved. doi:10.1016/j.bbrc.2010.02.150 190 E. Yakubov et al. / Biochemical and Biophysical Research Communications 394 (2010) 189–193 growth media containing DMEM (Invitrogen), 10% FBS (Beit- ward 50 -GTGGACCTGACCTGCCGTCT; Reverse 50 - GGAGGAGTGGG Haemek, Israel), 4 mM L-glutamine, 10 mM HEPES and 1:100 pen- TGTCGCTGT). PCRs consisted of 21–27 cycles of 95 °C for 1 min, icillin–streptomycin (Invitrogen). Transfection was in 1 ml of 55 °C for 45 s, and 72 °C for 1 min. antibiotic-free hFF medium supplemented with 4 lg of RNA mix (1.0 lg of each of the 4 TFs) and 2 ll of Lipofectamine 2000 (Invit- 2.4. Alkaline phosphatase staining and immunocytochemistry rogen) per well of six-well plate according to the manufacturer’s instructions. After 5 h of incubation the medium was changed to Alkaline phosphatase (AP) staining was performed using the fresh hFF medium without RNA and Lipofectamine while contain- Vector Red Substrate Kit (Vector Laboratories) according to the ing antibiotic. Usually five consecutive transfections were per- manufacturer’s protocol. The AP staining was recorded as red color formed 24 h apart. After the last transfection the hFF cells were or by fluorescence. For Immunostaining cells were fixed in 4% para- transferred to irradiated murine embryonic fibroblasts (iMEF) formaldehyde in PBS and immunostained according to standard and cultured with hES medium prepared as DMEM/F12 (Invitro- protocols using the following primary antibodies: GFP (rabbit, gen) supplemented with 20% knockout serum replacement, 2 mM L-glutamin, 1:100 nonessential amino acids, 1:100 penicillin– streptomycin (Invitrogen), 2 lg/ml Heparin (Sigma) and 10 ng/ml basic FGF (Peprotech). The medium was replaced daily. Colonies of iPS were observed between 1 and 2 weeks after the last transfection. After 10 days on iMEF the hES medium was replaced with 50– 75% conditioned medium. Primary colonies were picked onto new iMEF plate 3 weeks after the start of the procedure. 2.2. Plasmids, primers, IVT with T7 RNA polymerase Plasmid pTM1 was described previously and was modified to yield pTMA, by the addition of a fragment containing polyA(82A) between the XhoI and NsiI sites after the 30 end of GFP (Supple- mentary Fig. 1). To clone each of the cDNAs of the TF (Oct4, Lin28, Sox2, or Nanog), into pTMA the GFP coding region was ex- cised from pTMA by EcoRI and either MscI or NcoI and was re- placed by the coding sequences of Oct4, Lin28, Sox2 or Nanog, respectively. The DNAs of were amplified by PCR from the respec- tive plasmids (Addgene Cat# 16579, 16580, 16577, 16578, 17219) using high fidelity DNA polymerase (Invitrogen, Cat#11304-011) and primers that include the appropriate restriction site sequences for cloning into pTMA after GFP excision. To prepare the RNA of the inserted TFs or of GFP, the pTMA was linearized by SalI after the polyA site, followed by in vitro RNA transcription (IVT) using the mMESSAGE mMACHINE T7 kit (Applied Biosystems/Ambion, TX, USA) that allows 50 cap formation. The IVT produced RNA includes also the IRES and the polyA. After DNaseI treatment, the tran- scribed RNA was recovered on RNeasy columns (Qiagen) according to the RNA Cleanup protocol of the manufacturer. RNA integrity was monitored by agarose gel electrophoresis and then with RT- PCR using dT25 and gene specific primers for each TF or GFP. 2.3. RNA isolation and RT-PCR Twenty four hour (including 5 h of transfection) after the first transfection about 100,000 hFF cells were harvested using stan- dard trypsinization procedure and frozen in liquid nitrogen. Total RNA was purified with Rneasy Mini kit (Qiagen). On-column DNase treatment (Qiagen) was performed to remove genomic DNA con- tamination. One microgram of total RNA was used for reverse tran- scription reaction with SuperScipt II (Invitrogen) and dT25 primer, according to the manufacturer’s instructions. The resulting cDNA mix was five-fold diluted and 2 ll was then used as template for PCR with ReddyMix PCR Mater Mix (Thermo Scientific). The set of primers used for the PCR was for Oct3/4 (Forward 50 -CAGT GCCCGAAACCCAC; Reverse 50 - GGAGACCCAGCAGCCTCAAA), for- Lin28 (Forward 50 -AAGCTGCCACCCCAGCCCAAGA; Reverse 50 -TTC TGTGCCTCCGGGAGCAGGGTA), for Sox2 (Forward 50 - AGCTACAGCA Fig. 1. Generation of specific mRNA used for production of iPS. (A) Structure of the TGATGCAGGA; Reverse 50 -GGTCATGGAGTTGTACTGCA), for Nanog DNA template in pTMA including the T7 promoter, IRES, cDNA, polyA, and SalI for linearization. The produced RNA is capped. (B) Determination by RT-PCR of the (Forward 50 -CAGAAGGCCTCAGCACCTAC; Reverse 50 -ATTGTTCCAG amount of each specific mRNA in hFF, following transfection with RNA mixture of GTCTGGTTGC), for GFP (Forward 50 - CACTACCAGCAGAACACCCCCA; four (OLSN) factors. (C) Schematic protocol for iPS generation by RNA transfection of Reverse 50 -ACAGCTCGTCCATGCCGAGAGT), and for GAPDH (For- hFF. T, transfection; OLSN, initials of the four TF. E. Yakubov et al. / Biochemical and Biophysical Research Communications 394 (2010) 189–193 191 Chemicon); hOCT4-3/4 (goat, Santa Cruz Biotechnology); hSOX2 19 h post transfection was determined using RT-PCR on RNA ob- (goat, Santa Cruz Biotechnology); hNanog (mouse monoclonal, Sig- tained from these cells (Fig. 1B). It was concluded that 1 lg per ma): SSEA4 (mouse monoclonal, Millipore). Appropriate Cy2 or well of six-well plate is optimal of each RNA for transfection. In Cy3-conjugated secondary antibodies (Jackson lab) were used. the case of GFP, the encoding RNA obtained was used to follow Stained cultures were photographed in Olympus microscope. the kinetics and stability of the intracellular expressed protein following the GFP fluorescence in the transfected cells. Protein 3. Results expression was detected 8 h after transfection, reached a maximal level at 24 h and declined towards 72 h with lower expression We used the pTMA plasmid (Supplemental Fig. 1) as a plat- that persisted longer than 72 h. Cells were transfected a second form to prepare the mRNAs of each of the transcription factors and third time at 24 h intervals and GFP expression persisted by in-vitro-transcription (IVT) using T7 RNA polymerase. The plas- for more than 48 h after each transfection. On the basis of this re- mid pTMA contains the T7 promoter followed by IRES sequence sult hFF cells were transfected with a mixture containing 1 lg and the GFP coding region followed by a polyA tail in the 30 re- RNA of each of the four factors according to the time-scale illus- gion (Fig. 1A). The coding sequences of human Oct4, Lin28, trated in Fig. 1C and protein expression was followed by immu- Sox2, or Nanog were amplified by high fidelity PCR from the nocytochemistry with antibodies to the various factors. The respective plasmids (Addgene, Cambridge, MA) and used to re- results showed that whereas the expressed GFP was confined to place the GFP in pTMA after appropriate restriction and ligation the cytoplasm, the transcription factors were localized predomi- with the pTMA vector (Fig. 1A). The plasmid was linearized at nantly to the nucleus indicating the authenticity of the RNA and restriction site SalI and subjected to IVT with T7 RNA polymerase its translation process (Fig. 2). The GFP fluorescence of the trans- which allowed for 50 cap formation (Ambion, Austin, TX). Supple- fected cells is shown in Fig. 2B whereas the staining with anti- mental Fig. 2 shows the resulting RNA as analyzed by gel electro- GFP clearly shows the cytoplasmic localization of GFP (Fig. 2C phoresis. To determine the amount necessary for transfection, the and E). The staining with antibody to OCT4 (Fig. 2F–H) and to RNA was transfected into hFF and the amount present in the cells SOX2 (Fig. 2I–K) depicts nuclear localization of the expressed TF Fig. 2. Protein expression in transfected hFF cells. (A) Untransfected hFF cells. (B) Fluorescence of GFP detected 19 h after transfection. (C–E) Immunocytochemistry of hFF cells transfected with mixtures of four OLSN-mRNA or GFP and separately detected with specific antibody 24 h after transfection. (C–E) GFP, (F–H) OCT4, and (I–K) SOX2. The middle square shows DAPI stain and tight one is a merge of the previous two. Transfection was with 1 lg of each RNA. Magnification was 100. Note the cytoplasmic localization of GFP and the nuclear localization of OCT4 and SOX2. 192 E. Yakubov et al. / Biochemical and Biophysical Research Communications 394 (2010) 189–193 which is clear by merging with DAPI staining. Similar results Fig. 3B and C and the expression of SSEA4 is shown in Fig. 3E were obtained also with IHC for Nanog and Lin28. and F. Note that Nanog is expressed mostly in the nucleus whereas The four factors were expressed in high proportion (70%) of SSEA4 is expressed in the cell membrane as is indicated by the the cells 24 h after first transfection. Later on the proportion of fac- merge with DAPI staining. After the fifth transfection the hFF cells tors expressing cells decreased and only 25% of the cells continue were transferred onto iMEF cells and grown with the hES medium. to express the proteins (data not shown). Nevertheless, this frac- Ten days after the last transfection we observed several small pri- tion of the cells showed stable expression since they retained the mary colonies. Fig. 4A depicts such colony in bright field and expressed transcription factor proteins even 7 days after the fifth Fig. 4B shows their dye fluorescence. The general morphology of transfection (Fig. 3). these colonies is shown in Fig. 4C and D at higher magnification. Since stable expression was observed for only 72 h after the first After further 10 days of growth a typical iPS colony after AP stain- transfection we speculate that the persistent expression of the fac- ing is shown in pink color in bright field (Fig. 4E) as well as in red tors 7 days after the fifth transfection may be due to activation of fluorescence (Fig. 4F). In addition this colony is shown after DAPI the endogenous genes of these factors rather than to residual staining (Fig. 4G) and after anti-Nanog staining whereas the MEF transfected RNA. This contention is supported by staining with background was negative (Fig. 4H) indicating strong Nanog expres- antibody to the stem cell surface marker SSEA4 which was not sion, likely from endogenous expression, since it was detected transfected at all but was very likely activated by the reprogram- 20 days post transfection. We obtained approximately 50 colonies ming process (Fig. 3). The expression of Nanog is shown in per 105 hFF cells and we are in the process of further analysis of the Fig. 3. Expression of Nanog and SSEA4 in hFF derived cells seven days after transfection as in protocol Fig 1. (A–C) Immunocytochemistry of Nanog using anti-Nanog at day 7 after the last transfection. (D–F) Immunocytochemistry of SSEA4 that was not included in the transfected RNA. In each line the first square was stained with DAPI the middle with specific antibody and the right one was a merge. Magnification 400. Fig. 4. Generation of mRNA induced iPS cells from hFF culture. (A–D) Primary (10 days from fifth transfection) iPS cells colonies visualized by AP staining. (A,C,D) bright field, (B) fluorescence. Primary (20 days from fifth transfection) RiPS cells colony. (E) AP staining, bright field, (F) AP staining, fluorescence, (G) DAPI, (H) anti-Nanog staining. Magnification (A,B) 40, (C,D) 400, (E–H) 100. E. Yakubov et al. / Biochemical and Biophysical Research Communications 394 (2010) 189–193 193 properties of these colonies and their pluripotency. Further optimi- cient. The construction of plasmids that allow the synthesis in the zation of the reprogramming process by using chromatin modifica- test tube of capped RNA containing IRES and polyA was found to be tion by small molecules (e.g. valproic acid and others) is also being superior in stability and protein expression over RNA that lacks examined. these elements (data not shown). The production of RiPS (RNA-induced pluripotent cells) cells by 4. Discussion RNA overcomes an important hurdle on the way to personalized cell therapy. It eliminates the hazard of insertion mutations and In this paper we describe an experimental approach for the genome damage that may be associated with the use of DNA vec- preparation of human iPS from foreskin fibroblasts using transfec- tors and may result in malignancy or genetic defects. It under- tion of mRNAs encoding the four TF whose DNA constructs were scores the potential of using functional RNA for regulating cell used previously to prepare iPS. We established a convenient and functions without genome integration. efficient plasmid platform to prepare in vitro relatively large amounts of translatable mRNA, using T7 RNA polymerase and the Acknowledgment linearized pTMA. The efficiency of transfection of this RNA was very high for all four factors and >70% of the cells expressed the This work was supported in part by the Kahn Family Founda- proteins encoded by the RNA, peaking at 24 h post transfection tion. We thank R. Kaufman, Drs. J. Chebath and E. Ainbinder for ad- and subsequently declining towards 72 h, probably through degra- vice and help and Dr. H. Gal for editorial assistance. dation. In the case of DNA transfection, the DNA has to enter the cell, penetrate the nucleus, integrate into the genome, where it is being transcribed to mRNA that crosses the nuclear membrane to Appendix A. Supplementary data the cytoplasm where it undergoes translation. In the case of the transfected RNA it is translated as it enters the cell and reaches Supplementary data associated with this article can be found, in the cytoplasm. This may explain the rapid expression and the high the online version, at doi:10.1016/j.bbrc.2010.02.150. yield of the protein product since the entire RNA is ready for trans- lation without dependence on the cellular transcription machinery. References Furthermore, the transient nature of the transfected RNA can be regulated and used to switch the plutipotential process on and K. Takahashi, S. Yamanaka, Induction of pluripotent stem cells from mouse embryonic and adult fibroblast cultures by defined factors, Cell 126 (2006) off. In the case of DNA transfection special precaution are neces- 663–676. sary to shut off the expression of the integrated transcription J. Yu, M.A. Vodyanik, K. Smuga-Otto, et al., Induced pluripotent stem cell lines factors. derived from human somatic cells, Science 318 (2007) 1917–1920. K. Okita, M. Nakagawa, H. Hyenjong, et al., Generation of mouse Surprisingly, almost nothing has been reported on the use of induced pluripotent stem cells without viral vectors, Science 322 (2008) functional RNA transfection as a tool to endow cells with a new 949–953. function that can be regulated. For example it was possible to M. Stadtfeld, M. Nagaya, J. Utikal, et al., Induced pluripotent stem cells generated without viral integration, Science 322 (2008) 945–949. change the phenotype of differentiated neurons into that of astro- M. Wernig, A. Meissner, R. Foreman, et al., In vitro reprogramming of cyte by transfer of the entire transcriptome of the astrocyte to neu- fibroblasts into a pluripotent ES-cell-like state, Nature 448 (2007) 318– rons. Very recently the RNA encoding the T cell receptor with 324. anti-HER2 specificity was utilized to transfect T cells that tran- B.W. Carey, S. Markoulaki, J. Hanna, et al., Reprogramming of murine and human somatic cells using a single polycistronic vector, Proc. Natl. Acad. Sci. siently became cytotoxic for cancer cells expressing HER2. This USA 106 (2009) 157–162. activity was maximal at day 2 and persisted not later than day 9 C.A. Sommer, M. Stadtfeld, G.J. Murphy, et al., Induced pluripotent stem cell. In our system five transfections were needed in order to generation using a single lentiviral stem cell cassette, Stem Cells 27 (2009) 543–549. switch-on the stem cell specific gene repertoire and later the trans- C.A. Sommer, A.G. Sommer, T.A. Longmire, et al., Excision of reprogramming fected RNA was eliminated without trace in the genome. transgenes improves the differentiation potential of iPS cells generated with a A recent report described the production of iPS without DNA single excisable vector, Stem Cells 28 (2010) 64–74. H. Zhou, S. Wu, J.Y. Joo, et al., Generation of induced pluripotent stem cells vectors, by using bacterially synthesized recombinant proteins of using recombinant proteins, Cell Stem Cell 4 (2009) 381–384. the four factors slightly modified to allow cell membrane penetra- J. McWhir, D. Wojtacha, A. Thomson, Routine culture and differentiation of tion. In this procedure the proteins were made in bacteria (a po- human embryonic stem cells, Methods Mol. Biol. 331 (2008) 77–90. B. Moss, O. Elroy-Stein, T. Mizukami, et al., New mammalian expression tential problem for therapeutic use) as inclusion bodies and then vectors, Nature 348 (1990) 91–92. were refolded and inserted into the cells. In our procedure using J.Y. Sul, C.W. Wu, F. Zeng, et al., Transcriptome transfer produces a predictable mRNA, the proteins fold in their normal cellular environment cellular phenotype, Proc. Natl. Acad. Sci. USA 106 (2009) 7624–7629. K. Birkholz, C. Krug, A. Hombach, S. Reuter, M. Kershaw, E. Kämpgen, G. and most importantly, they undergo the natural post translational Schuler, H. Abken, N. Schaft, J. Dörrie, Transfer of mRNA encoding recombinant modifications. We point out that the procedure of RNA preparation immunoreceptors reprograms CD4+ and CD8+ T cells for use in the adoptive in vitro is simple and easy to scale up and transfection is very effi- immunotherapy of cancer, Gene Ther. 16 (2009) 596–604.