Lecture 3: iPS Cells PDF
Document Details
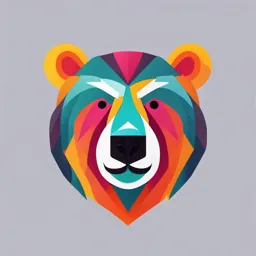
Uploaded by VeritableAmber980
Monash University
Tags
Related
- Sports Injury Psychology - UNIL - PDF
- Lecture 5 - Genetics PDF
- Induction of Pluripotent Stem Cells from Mouse Embryonic and Adult Fibroblast Cultures by Defined Factors PDF
- Stem Cells L23_F2024v2 - PDF
- Gene Regulation Lecture Outline - A01-2024 PDF
- Induction of Pluripotent Stem Cells from Mouse Embryonic and Adult Fibroblast Cultures by Defined Factors PDF
Summary
This document describes iPS cells, their potential applications, and the reprogramming process required. It also explores the epigenetic mechanisms regulating gene expression during differentiation. It covers the topic of stem cell biology and associated molecular mechanisms.
Full Transcript
🥽 Lecture 3: iPS cells LO: That differentiated adult cells can be reprogrammed back into pluripotency What’s so exciting about iPS cells? iPS cells are self-renewing and have the potential to be directed to form...
🥽 Lecture 3: iPS cells LO: That differentiated adult cells can be reprogrammed back into pluripotency What’s so exciting about iPS cells? iPS cells are self-renewing and have the potential to be directed to form any cell type of the adult body Scaleable supply of these stem cells No requirement for donor human embryos (oocytes) Relative ease in obtaining donor tissue and in generating cell lines in the laboratory Patient-donor specific Development of in vitro disease models Potential for use in drug, anti-cancer discoveries, and in clinical cell based therapies iPS bright promise Lecture 3: iPS cells 1 In vitro differentiation of iPS to look at the affected cell type, and screened for therapeutic compounds to develop disease-specific drugs for the patients Gene targeting can be used to repair iPS and they can differentiate (in vitro) into healthy cells → transplantation of the genetically matched healthy cells can occur LO: The factors commonly used for reprogramming, and how they are delivered Four Yamanaka factors that can be used to reprogram cells back into a pluripotent state There are now many more factors The four key defining factors: OCT3/4, SOX2, KLF4 and c-MYC (referred to as OSKM) Capable of reprogramming differentiated adult fibroblasts back into pluripotency (in the experiments with Yamanaka and colleagues) There are many different reprogramming methods that have been attempted Lecture 3: iPS cells 2 These are some delivery modes for iPS, as well as their efficiency relative to their safety LO: The sequence of molecular events (particularly the epigenetics) underlying reprogramming Starting point: how do cells transition from the pluripotent state to a differentiated state Note: As cells differentiate from a totipotent state (e.g., zygote) to a pluripotent state (e.g., blastocyst) to a multipotent state (e.g., trilaminar embryo) and beyond, the genetic information within the cells is not lost during development. While all cells retain the same genome, the differentiation process involves selective activation or silencing of different parts of the genome. This selective gene expression is regulated by various mechanisms, including epigenetic modifications (e.g., DNA methylation, histone Lecture 3: iPS cells 3 modification) and transcription factors, which determine the specific characteristics and functions of each cell type. “Genetics proposes, epigenetics disposes” Epigenetics - DNA methylation and histone modifications that are heritable in the short term but do not change the DNA or create mutations This is the way in which the genome is regulated so that the same genome in different cells can be switched on in different ways Epigenetic regulation of gene expression is all about transcription factor access and chromatin structural regulation DNA methylation changes the accessibility of the DNA to transcription factors Nucleosome rearrangement - making the chromatin either more open and accessible for transcription, or very close down and inaccessible to transcription Histone variant replacement Post-translational modifications of histones Chromatin packaging by HP1 or PRC1 Essentially epigenetics is all about accessibility - is the chromatin open and accessible for transcription factors to access it and switch on the genes in that region? Oct3/4 - combining different epigenetic events to control gene expression Oct3/4 POU-domain transcription factor Expressed in early embryogenesis, early germ cells, ES and EC cells Critical factor in maintenance of pluripotency When pluripotent cells are driven to differentiate (eg. using retinoic acid) Lecture 3: iPS cells 4 DOWNWARD trend Oct-3/4: a transcription factor that promotes the expression of genes that keep cells in an undifferentiated, pluripotent state Me-H3(K4): a histone mark associated with active transcription; typically found at the promoters of actively transcribed genes Ac-H3(K9): histone acetylation is generally associated with open chromatin and active gene transcription UPWARD trend Me-H3(K9): a histone mark associated with gene repression and heterochromatin formation DNA-me: DNA methylation is a key epigenetic modification associated with long-term gene silencing; prevents the binding of transcription factors and recruits proteins that compact chromatin ^^ This sequence is reversed in the transition to pluripotency During reprogramming, the epigenome is reset to an ESC stage Oct4 and Nanog are observed to be demethylated in ES cells and iPS, while being methylated in MEFs (differentiated cells) Lecture 3: iPS cells 5 Reprogramming into iPS cells appears universal Able to be carried out in mice, monkeys, and humans Events during reprogramming 4 factors - OKSM - occupy multiple loci to drive chromatin remodelling This early phase of reprogramming results in somatic gene suppression, mesenchymal-to-epithelial transition, and changes in basic metabolism If a cell does not complete any one of these processes ^^, programming is halted In the late phase of reprogramming, pluripotency genes are activated, tissue-specific and developmental genes are repressed, and chromatin undergoes changes in the H3K4m33/H3K27me3 in certain promoters. Reprogramming sequence Stochastic phase Random and variable changes with uncertain outcomes. Down-regulation of lineage specific genes Commencement of mesenchymal-epithelial transition Lecture 3: iPS cells 6 Proliferation Metabolism shift Deterministic phase Predictable and orderly progression to a stable pluripotent state. Bivalent methylation of H3K4m33/H3K27me3 on promoter regions Sequential activation of pluripotency genes Flat, round morphology LO: How iPSC lines are assessed for pluripotency Assessing iPSC lines - iPSC pluripotency is determined through transcriptome analysis, teratoma (”gold standard”) and chimera (but not using human iPSCs cells, using mice’s instead) formation. Teratoma generation is considered a gold standard for assessing iPSCs because it provides a definitive, in vivo test of the cells' ability to differentiate into all three germ layers. This method verifies that the iPSCs are truly pluripotent, capable of generating a diverse range of tissues. However, not all iPSCs are equal There are differences at the genomic, epigenomic, and transcriptome level between different iPSCs lines → differences impact on the differentiation ability of iPSC lines iPSCs retain epigenetic memories of their cell type of origin, which can bias their differentiation Lecture 3: iPS cells 7 Pluripotent stem cells are not all the same Differences in PSCs may further explain why differentiation to certain cell types has remained elusive Eg) ESCs and EpiSCs (epiblast-derived SC) are two types of PSCs: ESCs - embryonic stem cells Originate from pre-implanation blastocyst Naive pluripotency - high potential to differentiate into any cell type, including both embryonic and extraembryonic lineages Contribute to all tissues of the chimeric embryo Lineage cue response - require specific and targeted signals to initiate differentiation into particular cell lineages (e.g., ectoderm, mesoderm, or endoderm), responding only when those cues are present. More typical in mice PSCs EpiSSCs - epiblast-derived stem cells Derived from the epiblast of post-implantation embryo Primed pluripotent state - where cells have already begin to transition towards specific developmental pathways and are less flexible in their potential to form extraembryonic tissues. No chimeras High response - more responsive to a broader range of differentiation signals & more likely to differentiate spontaneously and with less stringent cues compared to ESCs. More typical in human PSCs Lecture 3: iPS cells 8 LO: How iPSCs may be differentiated using different cues Directed differentiation of iPSCs The most effective way to drive differentiation of a lineage is to take cues from in vivo development, and apply them in vitro LO: How iPSCs may be differentiated using different culture approaches 2D and embryoid bodies Removal of pluripotency support (eg. LIF) allows for PSCs to differentiate When PSCs are grown in non-adhesive conditions, they aggregate, allowing for cell-cell communication similar to that in the embryo Can either occur in either culture method of non-adherent plastic or PSCs in hanging drop culture Non-adherent plastic Random association of cells/ cell aggregation Random in size Lecture 3: iPS cells 9 Ease of setup PSCs in haning drop culture Defined starting population Small volumes for adding growth factors More difficult setup Embryoid Bodies: These PSCs aggregates mimic early embryogenesis but are disorganised. New 3D approaches Organoids - ex vivo culture of patient tissues, employing the ability of endogenous tissue stem cells OR produced from individualised induced pluripotent stem cell lines Organoids - ex vivo culture that retains tissue microenvironment cues allows for tissue and even organ complexity are experimentally responsive can be exposed to factors eg compound toxicity testing individualised cultures can allow for pre-clinical evaluation of patient responses LO: Basic safety considerations of translation into clinical use Care - we need to ensure: (i) the reprogramming process is well-defined and properly assessed (ii) incompletely differentiated and pluripotent cells must be identified and removed (iii) genomic and epigenetic changes induced by the reprogramming, and ongoing culture, must be identified (iv) the differentiation performance of iPSC in pre-clinical studies must be determined (v) the development of standard operating procedures (SOPs) for clinical use cells Lecture 3: iPS cells 10