Common Respiratory Disorders PDF
Document Details
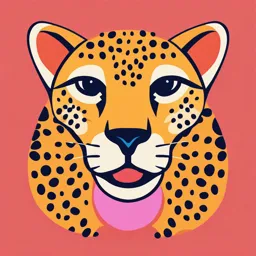
Uploaded by LavishVeena
Central Mindanao University
JACE DAKOTA JOHNNY, DENISE EVANS WARD, and HEIDI M. FAVERO
Tags
Summary
This chapter details common respiratory disorders, including pneumonia, COPD, and asthma. It covers the etiology, pathophysiology, assessment, management, and prevention of these conditions.
Full Transcript
23 Common Respiratory Disorders JACE DAKOTA JOHNNY, DENISE EVANS WARD, AND HEIDI M. FAVERO LEARNING OBJECTIVES Based on the content in this chapter, the reader should be able to: 1. Compare the etiology, pathophysiology, assessment, management, and...
23 Common Respiratory Disorders JACE DAKOTA JOHNNY, DENISE EVANS WARD, AND HEIDI M. FAVERO LEARNING OBJECTIVES Based on the content in this chapter, the reader should be able to: 1. Compare the etiology, pathophysiology, assessment, management, and prevention of community-acquired, hospital-acquired, and ventilator-associated pneumonia. 2. Discuss the pathophysiology, assessment, and management of pleural effusion. 3. Describe the pathophysiology, assessment, and management associated with pneumothorax. 4. Discuss the pathophysiology, assessment, management, and prevention of pulmonary embolism. 5. Explain the pathophysiology, assessment, management, and prevention of chronic obstructive pulmonary disease (COPD). 6. Describe the pathology, assessment, and management of a patient with asthma, including acute asthma, asthma exacerbations, and asthma with COPD. 7. Explain the key characteristics of hypoxemic acute respiratory failure and hypercapnic acute respiratory failure in terms of pathophysiology, assessment, and management. Symptoms associated with common respiratory disorders can mimic or exacerbate other disease processes. Understanding the pathophysiology of the different respiratory disorders, in conjunction with an adequate physical assessment and patient history, will enhance the ability of the nurse to quickly assess and initiate the appropriate therapy. Prompt treatment often prevents complications for the patient and, possibly, saves the patient’s life. Pneumonia Pneumonia remains a common infection found in both the community and hospital, even though there have been advances in identifying people at risk and implementing preventive measures. Critical care nurses encounter pneumonia when it complicates the course of a serious illness or leads to acute respiratory distress. Pneumonia is an infection involving the lower respiratory tract, caused by any class of organism (i.e., bacteria, viruses, fungi, amoebae, or parasites) associated with human infections. Pneumonia is the leading cause of infectious deaths worldwide. In the United States, pneumonia combined with influenza is the eighth leading cause of death and the most common cause of hospitalization behind females giving birth. 1 , 2 In most cases, the diagnosis of community-acquired pneumonia (CAP) is straightforward; however, in the older population, other common diseases such as cardiovascular disease can present with similar symptoms. Also, older populations can present with atypical symptoms. In the outpatient setting, CAP is typically a mild form of pneumonia; patients requiring hospitalization usually have a more severe form of CAP or other comorbidities, contributing to risk of mortality. 3 Hospital admission decisions can be inconsistent and may not reflect disease severity as there are no cardinal hallmarks of pneumonia. Risk- stratification tools have been developed to assist in objectively identifying pneumonia severity. Both the Infectious Disease Society of America (IDSA) and the American Thoracic Society (ATS) recommend using the Pneumonia Severity Index (PSI) over the CURB-65 score ( Box 23-1 ) to help determine the need for hospitalization in an adult diagnosed with CAP. 4 , 5 The PSI was developed as part of the Pneumonia Patient Outcomes Research Team (PORT) cohort study for the purpose of identifying patients with CAP at low risk for mortality. The PSI stratifies patients into five risk categories: the higher the score, the higher the risk of death, admission to the intensive care unit (ICU), or readmission, and the longer the length of stay. Patients in risk classes I, II, and III are at low risk for death and can likely be treated safely in the outpatient setting. Patients in risk classes IV and V should be hospitalized, with those in class V being admitted to the ICU. The PSI is a validated tool with multiple variables that requires less effort now with the availability of online calculators. 3 The CURB-65 is still utilized due to five easily measurable variables. Patients with a score of 0 or 1 can most likely be treated in the outpatient setting. Patients with a score of 2 should be hospitalized, those with a score of 3 should be assessed for possible ICU admission, and those with a score of 4 or 5 should be admitted to the ICU. 2 , 4 BOX 23-1 Community-Acquired Pneumonia Severity Index (PSI) for Adults Sex M (0 points) F (–10 points) Demographic Factors Age (1 point for each year) Nursing home resident (10 points) Comorbid Illnesses Neoplastic disease (30 points) Liver disease (20 points) Congestive heart failure (10 points) Cerebrovascular disease (10 points) Renal disease (10 points) Physical Examination Findings Altered mental status (20 points) RR ≥30/min (20 points) Systolic BP 5% mesothelial cells Asbestos effusions, drug reaction Glucose 200 units/dL Esophageal perforation, pancreatic disease, lung or pancreas malignancy pH 140 beats/min with any of the following: peripheral cyanosis, hypotension, pulseless electrical activity) Diagnostic Studies A chest radiograph or lung ultrasound is done to confirm the diagnosis of simple pneumothorax. 22 , 23 A chest CT scan is sometimes used to help with diagnosis, depending on area and size of the pneumothorax. Tension pneumothorax is considered in any rapidly deteriorating patient, and intervention should not be delayed for radiographic confirmation. A patient on mechanical ventilation is more likely to present with hypotension, subcutaneous (SQ) emphysema, and arrest. 22 Management Supplemental oxygen accelerates the rate of air resorption from the pleural space. If the patient is asymptomatic and the pneumothorax is less than 20%, no medical intervention is required. If the pneumothorax is greater than 20%, a chest tube is placed in the apical and anterior aspect of the pleural space to assist air removal. Connecting the chest tube to underwater seal drainage alone is usually adequate to resolve the pneumothorax. If the pneumothorax persists after 12 to 24 hours of underwater seal drainage, 15 to 20 cm H 2 O suction should be applied to facilitate closure. 24 In patients with suspected tension pneumothorax, a large-bore (16- or 18- gauge) needle may be placed into the anterior second intercostal space if a chest tube is not available. After needle insertion, a chest tube is placed and connected to underwater seal drainage. When the tension pneumothorax is relieved, the effect is rapid and is evidenced by an improvement in oxygenation, a decrease in heart rate (HR), and an increase in blood pressure (BP). Pulmonary Embolism Venous thromboembolism (VTE) includes both PE and deep vein thrombosis (DVT). If this condition is not quickly recognized and treated immediately, it can have deadly consequences, including sudden death when a thrombus breaks loose and enters into the pulmonary circulation. VTE affects up to 600,000 people and causes more than 100,000 deaths in the United States each year. This preventable condition has an annual mortality rate greater than acquired immunodeficiency syndrome (AIDS), motor vehicle accidents, and breast cancer combined, making prevention a top priority. Prevention strategies aim toward assessing a patient’s risk and implementing appropriate prophylactic therapy. 25 Etiology A number of environmental and genetic factors contribute to the risk of developing VTE. Acquired risk factors include age, recent surgery, cancer, and thrombophilia. Risk factors that increase the incidence of VTE are listed in Box 23-5. Most incidence of PE occur when a thrombus (clot) breaks loose and migrates to the pulmonary arteries, obstructing part of the pulmonary vascular tree ( Fig. 23-2 ). Nonthrombotic causes of PE include fat (from long bone fracture), air (during neurosurgery, or from central venous catheters), and amniotic fluid (during active labor), but these are much less common than thromboembolism. 26 BOX 23-5 Risk Factors for Thromboembolism Risk Factors Cancer Major surgery or trauma Prolonged immobilization, including long-distance flights or recent hospital stay Pregnancy and puerperium Contraceptives and hormone replacement therapy Inherited blood clotting disorders Previous VTE Atrial fibrillation Heart failure Obesity Smoking FIGURE 23-2 Sites of pulmonary emboli. (From Anatomical Chart.. Atlas of pathophysiology [3rd ed., p. 107]. Lippincott Williams & Wilkins.) Thrombus formation usually occurs in a large deep vein. Lower extremities are a common area for the formation of DVT. Other sites for thrombus formation include the right side of the heart (as in untreated atrial fibrillation) and the veins in the pelvic region. 26 Pathophysiology A cascade of events begins the process of thromboembolus development. Virchow triad of factors, which include venous stasis, hypercoagulability, and vascular endothelium damage, has long been believed to contribute to VTE. Development of venous stasis occurs when there is slowing of blood flow return to the heart. Immobility, heart failure, and varicose veins are conditions that predispose a person to thrombus formation due to venous stasis. Hypercoagulability occurs when there is disruption in the fine balance between the activation of clotting factors and the fibrinolytic system that prevents thrombus formation. Common reasons for this disruption include trauma, surgery, malignancy, pregnancy, oral contraceptive use, and hormone replacement therapy. Patients with cancer have a greater risk if they are receiving chemotherapy or radiation therapy or if they have undergone surgery or have metastatic disease. Less common factors included inherited thrombophilias, including factor V Leiden, prothrombin gene mutation 20210, and deficiencies of protein C, protein S, and antithrombin. Inherited thrombophilias are suspected in younger individuals diagnosed with VTE, those with a family history of VTE, and those in whom the development of VTE is not correlated with common risk factors (see Box 23-5 ). Damage to the vessel wall causes adhesion and aggregation of platelets and contributes to the activation of clotting factors. Common reasons for damaged vessels include local trauma, infection, surgical incisions, and atherosclerosis. Distal DVTs (e.g., in the calf) are considered stable, as only 15% will extend proximally and develop PE. Proximal DVTs (e.g., in popliteal, iliofemoral veins) are more likely to embolize into the pulmonary vascular system. 27 Approximately 50% to 70% of patients with the initial diagnosis of a PE will subsequently be diagnosed with a DVT in the lower extremity. 26 Occlusion in the pulmonary vascular artery by an embolus can produce both pulmonary and hemodynamic changes. Respiratory dead space within the pulmonary system occurs when the alveoli are ventilated but not perfused by the blood that normally flows through the pulmonary arteries and capillaries. This produces areas of mismatched ventilation and perfusion. As a result, well-ventilated alveoli are underperfused, and gas exchange is compromised. Pulmonary vascular constriction occurs due to vessel obstruction and neurohumoral reflexes. Blood flow is shunted from the underperfused alveoli to alveoli that are being perfused. This causes a state where perfusion is now greater than normal ventilation. When this occurs, a percentage of the blood will not be perfused and will return to the left side of the heart without having experienced gas exchange, leading to hypoxemia. Direct physiologic pulmonary changes to adapt to the decreased gas exchange include increased minute ventilation, decreased vital capacity, increased airway resistance, and decreased diffusing capacity. The severity of hemodynamic change in PE depends on the size of the embolus and degree of pulmonary vascular obstruction as well as preexisting cardiopulmonary conditions. In patients with no previous cardiopulmonary disease, there is a relationship between the degree of pulmonary artery obstruction and the pulmonary artery pressure. Increased right ventricular afterload results from obstruction of the pulmonary vascular bed by embolism. Hemodynamic stability is a strong predictor of mortality. If the patient survives, the most common cause of death within 1 month is right ventricular failure. Heart failure occurs due to increased wall stress and ischemia that compromises right ventricular function impairing left ventricular output. Right ventricular dysfunction can occur in patients with submassive PE, even if the patient was initially hemodynamically stable. 28 Assessment PE can be difficult to identify due to its nonspecific clinical presentation; less than 10% of those with fatal emboli receive adequate treatment. 26 PE is suspected in a patient with new onset of dyspnea, tachycardia, or sustained hypotension without other explanations. Other symptoms include chest pain, coughing with or without hemoptysis, clinical signs of DVT, and syncope. 26 PE may also be an incidental finding in asymptomatic patients undergoing imaging for other reasons. 27 Signs and symptoms of PE are listed in Box 23- 6. BOX 23-6 PATIENT SAFETY Signs and Symptoms of Pulmonary Embolism Small-to-Moderate Embolus Dyspnea Tachypnea Tachycardia Chest pain Mild fever Hypoxemia Apprehension Cough Diaphoresis Decreased breath sounds over affected area Rales Wheezing Massive Embolus A more pronounced manifestation of the abovementioned signs and symptoms, plus: Cyanosis Restlessness Anxiety Confusion Hypotension Cool, clammy skin Decreased urinary output Pleuritic chest pain: associated with pulmonary infarction Hemoptysis: associated with pulmonary infarction Signs of Pulmonary Embolism in Patients in Intensive Care Worsening hypoxemia or hypocapnia in a patient on spontaneous ventilation Worsening hypoxemia and hypercapnia in a sedate patient on controlled mechanical ventilation Worsening dyspnea, hypoxemia, and a reduction in PaCO 2 in a patient with chronic lung disease and known carbon dioxide retention Unexplained fever Sudden elevation in pulmonary artery pressure or central venous pressure in a hemodynamically monitored patient In a patient presenting with findings concerning for DVT or PE, a D-dimer assay may be used to rule out the disease in those considered low risk. An elevated D-dimer in a patient considered at an increased risk warrants further study. Computed pulmonary angiography (CTPA) is the mainstay for the diagnosis of acute PE; however, it is contraindicated in those with a contrast dye allergy or acute kidney disease. In patients with contraindications to contrast dye or potentially false-negative CTPA, a V/Q scan may be used. Transthoracic echocardiogram is often used to assess for cardiac dysfunction due to PE. 28 DVT is often associated with acute PE. It is important to assess for a DVT when patients present with symptoms of an acute PE or unilateral leg swelling and tenderness. Ultrasonography is the recommended imaging modality for the diagnosis of DVT. 29 Management Heparin and thrombolytic agents are used to treat PE. Guidelines developed by the American College of Chest Physicians (ACCP) for the treatment of VTE are shown in Table 23-5. 27 , 30 TABLE 23-5 American College of Chest Physicians Recommendations for Treatment of Venous Thromboembolism Anticoagulation Recommended Therapy Guidelines for Unfractionated Heparin (UFH) Suspected VTE Obtain baseline aPTT, PT, CBC. Check for contraindications to heparin therapy. Give heparin 5,000 units IV. Order imaging study. Confirmed VTE Re-bolus with heparin 80 units/kg IV or 5,000 units, and start maintenance infusion at 18 units/kg/h or 1,300 units/h. Check aPTT at 6 h; maintain a range corresponding to a therapeutic heparin level. If starting warfarin therapy, initiate on day 1 at 5 mg; adjust subsequent daily dose according to INR (no bolus dose) Stop heparin after 3–5 d of combined therapy, when INR is 2.0 (2.0–3.0) for 24 h. Anticoagulation with warfarin or NOAC for at least 3 mo (target INR for warfarin 2.5; 2.0–3.0). Low-Molecular-Weight Heparin (LMWH) Suspected VTE Obtain baseline aPTT, PT, CBC. Check for contraindication to heparin therapy. Give UFH, 5,000 units IV. Order imaging study. Confirmed VTE Give LMWH (enoxaparin), 1 mg/kg SQ every 12 h or 1.5 mg/kg daily If starting warfarin therapy, initiate on day 1 at 5 mg; adjust subsequent daily dose according to the INR. Consider checking platelet count between days 3 and 5. Stop LMWH if on warfarin after at least 4–5 d of combined therapy, when INR is 2.0 on 2 consecutive days. Agent of choice for continued anticoagulation in patients with active cancer. Anticoagulate with warfarin of NOAC for at least 3 mo (goal INR 2.5; 2.0–3.0), then it is recommended to continue with low- intensity therapy (INR range 1.5–1.9) with less frequent monitoring over stopping treatment. aPTT, activated partial thromboplastin time; PT, prothrombin time; NOAC: novel oral anticoagulants (e.g., apixaban, rivaroxaban). American College of Chest Physicians. (2012). Ninth ACCP consensus conference on Antithrombotic and Thrombolytic Therapy. Chest, 41 (2 Suppl.), 141(2), e419S–e496S; Kearon, C., Akl, E. A., Ornelas, J., Blaivas, A., Jimenez, D., Bounameaux, H., Huisman, M., King, C. S., Morris, T. A., Sood, N., Stevens, S. M., Vintch, J. R. E., Wells, P., Woller, S. C., & Moores, L. (2016). Antithrombotic therapy for VTE disease: CHEST guideline and expert panel report. Chest, 149 (2), 315–352. https://doi.org/10.1016/j.chest.2015.11.026. Patients with DVT or PE should immediately start treatment with parenteral anticoagulant therapy. Options include SQ low-molecular-weight heparin (LMWH), unfractionated IV heparin, SQ fondaparinux, and adjusted- dose SQ heparin. Though adjusted-dose SQ heparin is an option for the treatment of PE, it is not commonly used because of the decreased bioavailability of heparin when given SQ. LMWH or SQ fondaparinux can be substituted for unfractionated heparin (UFH) in patients with DVT and in stable patients with PE. IV UFH should only be used in unstable patients, in patient with obesity when there are concerns about absorption, and in patients with increased risk of bleeding. 30 Treatment with heparin or LMWH should continue for at least 5 days. Oral anticoagulation should be started the same day the initial IV/SQ treatment is initiated and continue until the patient’s international normalization ratio (INR) is within therapeutic treatment range. Current drug classes that are recommended for oral anticoagulation include vitamin K antagonist (VKA) (i.e., warfarin) and factor Xa/IIa (thrombin) inhibitors. Unlike warfarin, the factor Xa/IIa inhibitors do not require close monitoring of the INR and are the preferred agents for long-term anticoagulation in patients with VTE and no underlying cancer. 27 However, only a few agents have an approved reversal agent if needed for hemorrhage. 31 The recommended length of anticoagulation therapy varies, depending on the patient’s age, comorbidities, and likelihood of recurrence of PE or DVT. In most patients, anticoagulation therapy with warfarin should be continued for 3 to 6 months. 32 In patients with a DVT or PE in the surgical setting or with a transient nonsurgical risk factor, anticoagulation for 3 months is warranted. 26 , 27 The first episode of an unprovoked DVT should be treated for at least 3 months. After 3 months, the patient should be evaluated for potential risks versus benefits before extending therapy. 31 Patients with new-onset DVT and a risk factor (e.g., cancer, inhibitor deficiency state) or recurrent venous thrombosis should be treated indefinitely. 31 Patients with massive PE or severe iliofemoral thrombosis may require a longer period of heparin therapy. 31 Thrombolytic therapy is only recommended for patients at high risk for death from refractory hypotension and hypoxemia from an acute massive PE. 29 All thrombolytic agents act systemically and have the potential to lyse a fresh platelet–fibrin clot anywhere and cause bleeding at that site. 31 Intracranial disease, recent intracranial or spinal surgery, trauma, history of hemorrhagic stroke, and hemorrhagic diseases are contraindications to thrombolytic therapy. Urokinase, streptokinase, and recombinant tissue plasminogen activator are the thrombolytic agents approved for treating PE and VTE. An IVC filter is recommended to prevent PE in patients with contraindications to heparin therapy (risk for major bleeding or drug sensitivity). 30 Placement of an IVC filter is also recommended in patients with recurring thromboembolism despite adequate anticoagulation, chronic recurrent embolism and pulmonary hypertension, and concurrent surgical pulmonary embolectomy or pulmonary endarterectomy procedure. Routine placement of an IVC filter is not recommended on patients eligible for systemic anticoagulation. 27 Prevention Prevention of VTE is essential to decreasing the morbidity and mortality associated with PE. Prophylactic measures are based on the patient’s specific risk factors. 30 See Table 23-6 for preventive measures recommended by the ACCP. TABLE 23-6 American College of Chest Physicians Recommendations for Prevention of Venous Thromboembolism Patient Population Recommended Therapy Level of Evidence Low-risk general Early and frequent ambulation B1 surgery Moderate-risk LMWH, LDUH, or mechanical prophylaxis, preferably with 2B, 2C general surgery IPCD Higher risk general LMWH, LDUH three times a day, or mechanical prophylaxis surgery High-risk general LDUH three times a day, LMWH, combined with graduated 1B, 2C surgery with compression stockings, and/or intermittent pneumatic multiple risk compression factors Total hip Use one of the following for a minimum of 10–14 days: 1B, 1C, 2C replacement or LMWH, fondaparinux, apixaban, dabigatran, rivaroxaban, total knee LDUH, adjusted-dose VKA, ASA, or intermittent pneumatic replacement compression device (IPCD); LMWH: started >12 h before surgery surgery or >12 h after surgery; dual prophylaxis with an antithrombotic agent and IPCD recommended during hospitalization Major trauma, IPCD when not contraindicated. LMWH and LDUH when risk 2C traumatic brain of bleeding diminishes. injury, acute spinal injury, traumatic spine injury Myocardial infarction UFH or LMWH or bivalirudin or fondaparinux A Intermittent pneumatic compression or elastic stockings C when heparin is contraindicated Ischemic stroke with UFH or LMWH or intermittent compression devices over no 2B restricted mobility prophylaxis Medical patients with LDUH TID, LMWH BID, or fondaparinux 1B risk factors for VTE (including heart failure and chest infections) Patients with long- Suggest against routine prophylaxis with LMWH or LDUH and 2B, 2C term indwelling suggest against the prophylactic use of VKAs (Coumadin) central vein catheters Patients receiving a Use appropriate patient selection and caution when using spinal puncture or anticoagulant thromboprophylaxis epidural catheter placement *1A1: Methods strong, results consistent—randomized clinical trials (RCTs), no heterogeneity, effect clear that benefits do (or do not) outweigh risks. 2A: Methods strong, results consistent—RCTs, no heterogeneity, effect equivocal—uncertain whether benefits outweigh risks. 1B: Methods strong, results inconsistent—RCTs, heterogeneity present, effect clear that benefits do (or do not) outweigh risks. 2B2: Methods strong, results inconsistent—RCTs, heterogeneity present, effect equivocal— uncertain whether benefits outweigh risks. 1C: Methods weak—observational studies, effect clear that benefits do (or do not) outweigh risks. 2C: Methods weak—observational studies, effect equivocal— uncertain whether benefits outweigh risks. LDUH, low-dose unfractionated heparin. Data from American College of Chest Physicians. (2012). Ninth ACCP Conference on Antithrombotic and Thrombolytic Therapy. Prevention of venous thromboembolism. Chest, 133 (412 Suppl.), 7S–47S. Chronic Obstructive Pulmonary Disease The two terms associated with COPD are chronic bronchitis (disease of the small airways) and emphysema (parenchymal destruction), which were often discussed as two different disease processes. However, COPD is a combination of both the disease of the small airways and the destruction of the lung parenchymal along with other several structural abnormalities ( Fig. 23-3 ). 33 Currently, COPD is defined as an airflow limitation that is not fully reversible. The airflow limitation is usually progressive and associated with an abnormal chronic inflammatory response of the lungs to noxious particles or gases (primarily cigarette smoke), abnormal lung development, or an inherited deficiency of alpha 1 -antitrypsin (see Spotlight on Genetics 23-1 ). 33 Refer to the Global Initiative for Chronic Obstructive Lung Disease (GOLD) Global Strategy for the Diagnosis, Management, and Prevention of Chronic Obstructive Pulmonary Disease for current updates ( https://goldcopd.org ). FIGURE 23-3 Mechanisms underlying airflow limitation in COPD. (Adapted from Global Initiative for Chronic Obstructive Lung Disease.. Global strategy for the diagnosis, management, and prevention of chronic obstructive lung disease. https://goldcopd.org/wp- content/uploads/2019/11/GOLD-2020-REPORT-ver1.0wms.pdf.) SPOTLIGHT ON GENETICS 23-1 RESPIRATORY SYSTEM—ALPHA 1 -ANTITRYPSIN DEFICIENCY This disorder affects about 1 in 1,500 to 3,500 individuals of European ancestry. It is uncommon in people of Asian descent but is commonly observed in patients who have COPD. Mutations in the SERPINA1 gene cause alpha 1 -antitrypsin deficiency. This gene provides instructions for making a protein called alpha 1 -antitrypsin, which protects the body from a powerful enzyme called neutrophil elastase. The mutation leads to a deficiency of alpha 1 -antitrypsin or an abnormal form of the protein that cannot control neutrophil elastase. Without enough functional alpha 1 -antitrypsin, neutrophil elastase destroys alveoli and causes lung disease. Numerous genetic tests are available for the detection of alpha 1 -antitrypsin deficiency. National Center for Biotechnology Information. (2020). Welcome to NCBI. Accessed October 29, 2020, from https://www.ncbi.nlm.nih.gov/ ; Ferrari da Cruz, T., Rufino, R., Lopes, A. J., Noronha, A., Medeiros Anselmo, F., & Henrique da Costa, C. (2020, September 24). Evaluation of the small airways in patients with chronic obstructive pulmonary disease and alpha-1 antitrypsin deficiency. International Journal of Chronic Obstructive Pulmonary Disease, 15 , 2267–2274. https://doi.org/10.2147/COPD.S262418. eCollection 2020. PMID: 33061344. Pathophysiology COPD is a major cause of chronic morbidity and mortality, ranking as the third leading cause of death in the United States. This number is expected to increase because of the aging population. 34 In COPD, pathologic changes occur in the central airways, peripheral airways, lung parenchyma, and pulmonary vasculature. As the disease progresses, several pathophysiologic changes can occur, including mucous hypersecretion, ciliary dysfunction, airflow limitation, pulmonary hyperinflation, gas exchange abnormalities, pulmonary hypertension, and cor pulmonale. The peripheral airways become the major site of obstruction in patients with COPD. The structural changes in the airway wall are the most important cause of the increase in peripheral airway resistance. Inflammatory changes such as airway edema and hypersecretion of mucus also contribute to narrowing of the peripheral airways. Hypersecretion of mucus is caused by the stimulation of the enlarged mucus-secreting glands and the increased number of goblet cells by inflammatory mediators and oxidative stress. Ciliated epithelial cells undergo squamous metaplasia, leading to impaired mucociliary clearance, which is usually the first physiologic abnormality to occur in COPD. The inflammatory response observed in patients with COPD is a modification of the normal response to chronic irritants, such as cigarette smoke. How a person responds to the inflammation may be in part determined by genetics, accounting for the variability in the development of the disease. Abnormalities may not be evident for years in some individuals, while others have an early onset of symptoms. 33 Airway obstruction is caused by inflammation of the major and small airways ( Fig. 23-4 ). Subsequently, edema and hyperplasia of submucosal glands and excess mucus excretion into the bronchial tree occur, resulting in a chronic productive cough. Cigarette smoking is the major causal factor in the development of airway obstruction caused by inflammation. Other causes of chronic airway irritation include air pollutants; use of biomass fuels for cooking, heating, or other household needs; and occupational exposures. Nonspecific pathologic changes in the lung, including infiltration of airway mucosa and submucosa with neutrophils and mononuclear cells, smooth muscle hypertrophy, and enlargement of the submucosal secretory glands, may also contribute to the development of COPD. 33 FIGURE 23-4 Small airway changes in COPD: inflammation and thickening produce narrowing of airways. Lined areas indicate secretions. Once the airway lumina are occluded by secretions and narrowed by a thickened wall, patients develop airflow obstruction. Acute bacterial or viral infection in patients with existing COPD can increase airway and parenchymal damage, impair mucociliary clearance, obstruct bronchioles, and contribute to chronic epithelial damage and bacterial colonization that further exacerbate symptoms and airway obstruction. 33 Even in nonsmoking patients, acute viral infection may lead to the chronic airway inflammation and chronic sputum production characteristic of COPD. In these patients, the symptoms may have a reversible component if the source of chronic infection or irritation is treated. These patients normally do not have hyperinflation or abnormal diffusion test results. 31 Parenchyma destruction includes the loss of lung elasticity and abnormal, permanent enlargement of the airspaces distal to the terminal bronchioles with destruction of the alveolar walls and capillary beds without obvious fibrosis ( Fig. 23-5 ). There are three areas of destruction: centrilobular, panacinar, and paraseptal ( Fig. 23-6 ). Centrilobular emphysema is common in smokers and often localizes in the upper lung zones. Panacinar emphysema is frequently found in patients with alpha 1 -protease inhibitor deficiency and is often localized in the lower lobes. Paraseptal emphysema is also common in smokers and is localized peripherally, with possible formation of large bullae. FIGURE 23-5 Parenchymal changes in COPD. Airspaces are enlarged in the emphysematous lung. (From Anatomical Chart.. Atlas of pathophysiology [4th ed., p. 100]. Lippincott Williams & Wilkins.) FIGURE 23-6 Types of emphysema. The acinus, the gas-exchanging structure of the lung distal to the terminal bronchiole, consists of the terminal bronchiole, respiratory bronchioles, alveolar ducts, alveolar sacs, and alveoli. In centrilobular (proximal acinar) emphysema, the respiratory bronchioles are mainly involved. In paraseptal (distal acinar) emphysema, the alveolar ducts are mainly affected. In panacinar (panlobular) emphysema, the acinus is uniformly damaged. The enlargement of the airspaces in COPD results in hyperinflation of the lungs and increased total lung capacity. This is believed to result from the breakdown of elastin, a type of connective tissue component in lung parenchyma, by enzymes, called proteases, which digest proteins. These proteases, especially elastase, are released from neutrophils, alveolar macrophages, and other inflammatory cells. 35 Two recognized conditions that cause these changes are smoking and inherited alpha 1 -antitrypsin deficiency. Smoking contributes to increased inflammatory cells in the alveoli, enhanced release of elastase from neutrophils, increased elastase activity in macrophages, and activation of mast cells that release mast cell elastases. Alpha 1 -antitrypsin usually protects the lung from the destructive inflammatory cells; however, the elastic tissue–destructive process continues unabated in patients with an inherited alpha 1 -antitrypsin deficiency. Almost all people who develop COPD before 40 years of age have an alpha 1 -antitrypsin deficiency. Evidence has shown that cigarette smoking decreases the levels of alpha 1 -antitrypsin and increases the number of macrophages in the alveolar walls. This vicious cycle promotes increased numbers of neutrophils. A hereditary deficiency in alpha 1 -antitrypsin is responsible for a small number of all cases of COPD. Smoking and repeated respiratory tract infections further decrease alpha 1 -antitrypsin levels, adding to the risk for COPD in people with low alpha 1 -antitrypsin levels. 33 A common phenomenon in COPD is spontaneous pneumothorax related to rupture of thinned parenchyma. Patients may experience acute severe dyspnea and respiratory failure depending on the amount of pulmonary reserve (see the discussion of barotrauma in the section on Pneumothorax). In advanced COPD, peripheral airway obstruction, parenchymal destruction, and pulmonary vascular irregularities reduce the lung’s capacity for gas exchange, resulting in hypoxemia (low blood oxygen) and hypercapnia (high blood carbon dioxide). A V/Q ratio mismatch is the driving force behind hypoxemia in patients with COPD, regardless of the stage of the disease. Chronic hypercapnia usually indicates inspiratory muscle dysfunction and alveolar hypoventilation. As hypoxemia and hypercapnia progress late in COPD, pulmonary hypertension often develops, which causes hypertrophy of the right ventricle, better known as cor pulmonale. Right-sided heart failure leads to further venous stasis and thrombosis that may potentially result in PE and further compromise the pulmonary circulation. Lastly, COPD is associated with systemic inflammation and skeletal muscle dysfunction that may result in limitation of exercise capacity and decline of health status. 33 Assessment A physical examination is rarely diagnostic in COPD, although it remains an important aspect of patient care. When evaluating a patient, key indicators to consider include the following: dyspnea that is progressive and persistent, chronic cough that may be intermittent and nonproductive, or cough with sputum production, history of smoking, or occupational exposures to dusts or chemicals for a significant period of time. 33 History A detailed medical history of a new patient with known or suspected COPD includes: Exposure to risk factors, such as smoking and occupational or environmental exposures to pollutants Past medical history, including asthma, allergy, sinusitis or nasal polyps, respiratory infections in childhood, other chronic respiratory diseases, and other nonrespiratory disease Family history of COPD or other chronic respiratory disease Pattern of symptom development. COPD typically develops in adults, and most patients are aware of the occurrence of increased breathlessness, increased frequency of winter “colds,” and some social restriction for a number of years before seeking medical attention. History of exacerbations or previous hospitalizations for respiratory disorder. Patients may be conscious of periodic worsening of symptoms even if these episodes have not been identified as acute exacerbations of COPD. Indications for hospital assessment or admission for acute exacerbation of COPD include a marked increase in intensity of symptoms; severe underlying COPD or other serious comorbidities; onset of new physical signs; frequent exacerbations, or failure of exacerbations to respond to medical management; older age; and insufficient home support. 33 Comorbidities such as cardiovascular disease, skeletal muscle dysfunction, osteoporosis, depression, and malignancies may also contribute to restriction of activity or treatments. Impact of disease on patient’s life, including limitation of activity, missed work and economic consequences, effect on family routines, feelings of depression or anxiety, decreased well-being, and interference with sexual activity Social and family support Possibilities for reducing risk factors, especially smoking cessation 33 Physical Findings The physical examination includes inspection, palpation, percussion, and auscultation. Inspection The examiner looks for: Central cyanosis or bluish discoloration of the mucosal membranes. This feature may be present but is difficult to detect in artificial light and in many racial groups. Common chest wall abnormalities, which reflect the pulmonary hyperinflation seen in COPD, including relatively horizontal ribs, barrel- shaped chest, and protruding abdomen Flattening of the hemidiaphragms, which may be associated with paradoxical indrawing of the lower rib cage on inspiration, reduced cardiac dullness, and widening xiphisternal angle Resting RR, which is often increased to more than 20 breaths/min; breathing may be shallow. Pursed-lip breathing, which may serve to slow expiratory flow and permit more efficient lung emptying Supraclavicular wasting and nasal flaring; resting muscle activation, which may be indicative of respiratory distress. While lying supine, patients with COPD often use the scalene and sternocleidomastoid muscles. Ankle or lower leg edema, which may be an indication of right-sided heart failure Palpation and Percussion Palpation and percussion are often unhelpful in COPD. The examiner should be alert for: Heart apex beat, which may be difficult to detect because of pulmonary hyperinflation Pulmonary hyperinflation, which also leads to downward displacement of the liver and an increase in the ability to palpate this organ without it actually being enlarged Auscultation Upon auscultation, the examiner may find: Reduced breath sounds Wheezing. Occurrence during quiet breathing is a useful indicator of airflow limitation; however, wheezing heard only after forced expiration is of no diagnostic significance. Inspiratory crackles, which occur in some patients with COPD but are of little assistance diagnostically Heart sounds heard over the xiphoid area Evidence of right heart failure secondary to COPD; includes an increased second heart sound, jugular venous distention, and right ventricular heave Indications for ICU admission for patients with acute exacerbation of COPD include severe dyspnea with inadequate response to therapy, changes in mental status, persistent or worsening hypoxemia, severe or worsening respiratory acidosis in spite of supplemental oxygen, hemodynamic instability, and the need for noninvasive and invasive mechanical ventilation. 33 Diagnostic Studies Laboratory and diagnostic tests in COPD are summarized in Table 23-7. TABLE 23-7 Laboratory and Diagnostic Tests for Patients With Chronic Obstructive Pulmonary Diseases Test Rationale Spirometry Measures FVC and FEV 1 ; gold standard for diagnosing disease and monitoring progression Diffusing capacity Measures the degree of lung function and provides information on the functional impact of emphysema related changes in COPD Bronchodilator Performed once during diagnosis stage and useful for the following reversibility reasons: To rule out an asthma diagnosis (If FEV 1 returns to predicted normal range after administration of bronchodilator, airflow limitation is likely due to asthma.) To establish a patient’s best attainable lung function at that point in time Chest radiography To exclude alternative diagnoses Evaluation of bullous disease Radiologic changes seen include: Flattened diaphragm on lateral chest film Increased volume of retrosternal airspace (signs of hyperinflation) Hyperlucency of the lungs Rapid tapering of vascular markings CT Not routinely recommended, except when doubt about the diagnosis of COPD or pending lung reduction surgery or lung transplant. ABGs Performed if FEV 1 is 20 breaths/min or PaCO 2 12,000 cells/mm or 10% immature (band) forms. *Specific treatment required. TRALI is a leading cause of transfusion-related mortality and morbidity. 4 It is theorized that an interaction occurs between the recipient’s blood and the donor’s, among the bioactive compounds produced during blood storage, or a combination of both. 4 , 5 Clinical presentation is the sudden onset of respiratory distress within 1 to 2 hours after a transfusion of red blood cells or thawed plasma. 5 The blood bank should be notified of a TRALI case, and the patient should not receive further blood products from that donor. ARDS is an expected complication of COVID-19, leading to worse outcomes and intensive care unit (ICU) admission. ARDS related to COVID-19 has many shared inflammatory processes as classical ARDS causes but differs in lung compliance (more compliant) and increased vascular thrombosis. 6 – 9 Management of the patient with ARDS associated with TRALI or COVID-19 is supportive and involves the same principles of mechanical ventilation used in ARDS and avoidance of aggressive diuresis. Diagnostic criteria for ARDS have been difficult to define because ARDS closely resembles other conditions. Diagnostic testing is used to “rule out” these conditions, yet the final diagnosis of ARDS is largely based on clinical presentation. No one test, including radiographic evidence, a plasma brain natriuretic peptide less than 500 pg/mL, or a pulmonary artery occlusion pressure (PAOP, formerly known as pulmonary artery wedge pressure) less than 18 cm H 2 O, is truly indicative of ARDS. An early feature of ARDS is diffuse alveolar damage. Biomarkers of inflammation may provide earlier recognition of ARDS before deterioration is seen in the clinical presentation. Currently, work is underway to identify these biomarkers in plasma, bronchoalveolar lavage, and the patient’s exhaled breath. 10 ARDS is estimated to occur in 38 to 81 patients per 100,000 of the population in the United States annually, with a 28% to 59% mortality rate. 11 The patients most at risk for the development of ARDS are those older than 65 years with a severe acute illness on presentation, such as sepsis or a preexisting chronic disorder. Although sepsis is the most common cause of ARDS, any person with one of the potential precipitating causes of ARDS is susceptible, and nurses need to be vigilant for early warning signs (see Box 24-1 ). Most patients with ARDS require a period of mechanical ventilation support for days to weeks. Pathophysiology ARDS was first described in case reports of patients presenting with acute tachypnea, decreased lung compliance, diffuse pulmonary infiltrates on chest radiograph, and hypoxemia. 12 Researchers using histologic examination of lungs have found that lungs show fibrosis and that pathologic processes are not limited to the lung endothelium but also a result of alterations of lung epithelium and vascular tissue as well as the development of hyaline membranes. Pathologic changes in lung vascular tissue, increased lung edema, and impaired gas exchange are hallmarks of ARDS and directly related to a cascade of events resulting from release of cellular and biochemical mediators. Pathologic Changes in ARDS Mediators released as a result of either direct or indirect injury can precipitate ARDS. There is a relationship among clinical presentation (hypoxemia resistant to supplemental oxygen, tachypnea, and dyspnea), mediator release (interleukins [ILs], tumor necrosis factor- alpha [TNF-alpha], and platelet-activating factor [PAF]), and pathologic changes (microvascular permeability, pulmonary hypertension, and pulmonary endothelial damage). Some primary mediators responsible for lung damage in ARDS and their major actions as they relate to ARDS are listed in Table 24-1. 13 , 14 TABLE 24-1 Examples of Pathologic Responses to Biologic Mediators Response Biologic Mediators Persistent inflammatory Cytokines: IL-1, IL-6, interferon-gamma (INF-gamma), TNF- response alpha, complement, thromboxane Endothelial membrane Complement, thromboxane, kinins, TNF-alpha, toxic oxygen disruption metabolites, leukotrienes, prostaglandins (PGE 1 and PGE 2 ) Selective vasoconstriction Thromboxane, TNF-alpha, PAF, toxic oxygen metabolites Systemic vasodilation Complement, prostaglandins, TNF-alpha, IL-1, IL-6 Myocardial depression Complement, leukotrienes, TNF-alpha, myocardial depressant factor Bronchoconstriction Complement, thromboxane, leukotrienes, PAF Adequate pulmonary gas exchange depends on open, air-filled alveoli, intact alveolar-capillary membranes, and normal blood flow through the pulmonary vasculature. Diffuse alveolar-capillary membrane damage occurs and increases membrane permeability, allowing fluids to move from the vascular space into the interstitial and alveolar space ( Fig. 24-1 ). Air spaces fill with bloody proteinaceous fluid and debris from degenerating cells, causing interstitial and alveolar edema, impairing oxygenation. Inflammatory mediators cause vasoconstriction of the pulmonary vascular bed, inducing pulmonary hypertension and reduced blood flow to portions of the lung. Because of the reduced blood flow and decreased hemoglobin (Hgb) in capillaries, there is a decrease in oxygen available for diffusion and transport, further impairing oxygenation. FIGURE 24-1 Pathogenesis of ARDS. Following a direct or indirect insult, inflammatory mediators, leukotrienes, oxidants, platelet activating factor (PAF), and proteases, lead to disruption of the alveolar capillary endothelium and alveolar epithelium that leads to the exudative phase (panel A). During resolution of ARDS, the proliferative phase (left side of panel B) begins with type II alveolar epithelial cells (AEC) dedifferentiating and replicating into type 1 AEC. Type 1 AEC restore the epithelial/endothelial barrier and begin the process of fluid removal. Macrophages clear apoptotic neutrophils and cellular debris. In some ARDS patients, extensive basement membrane damage and inadequate or delayed reepithelialization leads to the fibrotic stage (right side of panel B) with interstitial and intraalveolar fibrosis and loss of gas exchange in that alveolus. ENaC, epithelial sodium channel; MIF, machrophage migration inhibitory factor. Reprinted with permission from Norris TL. Porth’s Pathophysiology, 10th ed. Wolters Kluwer, 2019. Adapted with permission from Norris TL. Porth’s Pathophysiology, 10th ed. Wolters Kluwer, 2019. The pathologic changes affect pulmonary blood vessels, gas exchange, and lung and bronchial mechanics ( Fig. 24-2 ). Ventilation is impaired from a decrease in lung compliance and increase in airway resistance. Lung compliance is reduced as a result of the stiffness of fluid-filled, nonaerated lung, giving the chest radiograph the classic “patchy” or “ground glass” appearance. Surfactant is lost, resulting in alveolar collapse. Mediator-induced bronchoconstriction restricts air flow. FIGURE 24-2 Pathophysiologic cascade is initiated by injury resulting in mediator release. The multiple effects result in changes to the alveoli, vascular tissue, and bronchi. The ultimate effect is ventilation–perfusion mismatch and refractory hypoxemia. Systemic Inflammatory Response Syndrome Systemic inflammatory response syndrome (SIRS) describes the inflammatory response occurring throughout the body and these symptoms are often manifested in patients with ARDS (see Box 24-1 ). The respiratory system may be the earliest and most common organ system to be involved in the systemic response. Thus, an understanding of the pathophysiology of SIRS and knowledge of the interventions used for SIRS are important in relation to ARDS. Often, patients with SIRS develop multiple organ dysfunction syndrome (MODS). As endothelial damage progresses and tissue hypoxia ensues, the inflammatory response is perpetuated, and the cascade intensifies (upregulates) with the release of more mediators. ARDS and MODS are therefore part of a vicious cycle in the continuum of SIRS. Determination of the triggers for SIRS and ARDS that are present in some individuals but not others and investigations of how to stop the cascade pathways are the subjects of ongoing research. For a more detailed discussion of SIRS and MODS, see Chapter 51. Stages of ARDS The pathologic changes associated with ARDS start with increasing pulmonary edema and progress to inflammation, fibrosis, and impaired healing in the later stages. Recognizing the dynamic nature of ARDS enables the nurse to understand the changes in physical assessment, mechanical ventilation strategies, treatment, and management that occur throughout the patient’s critical care stay. In stage 1, diagnosis is difficult because the signs of impending ARDS are subtle. Clinically, the patient exhibits increased dyspnea and tachypnea, but there are few radiographic changes. At this point, neutrophils are sequestering; however, there is no evidence of cellular damage. Within 24 hours (a critical time for early treatment), the symptoms of respiratory distress increase in severity, with cyanosis, coarse bilateral crackles on auscultation, and radiographic changes consistent with patchy infiltrates. A dry cough or chest pain may be present. It is at this point (stage 2) that the mediator- induced disruption of the vascular bed results in increased interstitial and alveolar edema. The endothelial and epithelial beds are increasingly permeable to proteins. This is referred to as the “exudative” stage. The hypoxemia is resistant to supplemental oxygen administration, and mechanical ventilation is required for worsening ratio of arterial oxygen to fraction of inspired oxygen (PaO 2 :FiO 2 ratio). Stage 3, the “proliferative” stage, develops from the 2nd to the 10th day after injury. Evidence of SIRS is now present, with hemodynamic instability, generalized edema, possible onset of nosocomial infections, increased hypoxemia, and lung involvement. Air bronchograms may be evident on chest radiography as well as decreased lung volumes and diffuse interstitial markings. Stage 4, the “fibrotic” stage, develops after 10 days and is typified by few additional radiographic changes. There is increasing multiorgan involvement, SIRS, and increases in the arterial carbon dioxide tension (PaCO 2 ) as progressive lung fibrosis and emphysematous changes result in increased dead space. Fibrotic lung changes result in ventilation management difficulties with increased airway pressure and development of pneumothoraces. Assessment History Obtaining an accurate and thorough history may provide information that allows for removal of the precipitating cause and interrupting the ensuing mediator response. The history may be difficult to obtain because of the critical presentation of the patient and problems associating a remote event with the ALI. Because the outcome is uncertain and often involves a long critical care admission, the health care team plays a large role in providing support to both the patient and the family. Developing a relationship early (e.g., by taking the time to obtain a thorough history) may assist with care throughout the course of admission. All health care team members contribute information to the history. Information about past relevant incidents (medications, blood transfusions, radiographic contrast agents), the use of medical and complementary therapies, and social factors may be helpful for the person’s care. Items of importance include assessment of risk factors for the development of ARDS (see Box 24-1 ), a social history to assess risk behaviors (e.g., human immunodeficiency virus status, smoking, substance misuse), medications (including over-the- counter medications), environmental exposures (chemical or biologic), and complementary therapies (all exogenous substances, including inhalations). This information is obtained in addition to the history of the present illness and presenting signs and symptoms. Physical Examination Acute respiratory failure initially may present within a few hours to several days, depending on the initial insult, and does not always progress to ARDS. Monitoring patients who meet the SIRS criteria (see Box 24-1 ) may aid identification of those who are at risk for the development of ARDS. There are few reliable early indicators of impending ARDS, and subtle changes may go unnoticed. Vital signs throughout the progression of ARDS vary, but the general trend is hypotension, tachycardia, and hyperthermia or hypothermia. Respiration, initially rapid and labored, varies once mechanical ventilation is instituted. Early signs and symptoms of respiratory failure include tachypnea, dyspnea, and tachycardia. Breath sounds often are clear in this phase ( Table 24-2 ). Patients with acute respiratory failure may exhibit neurologic changes, such as restlessness and agitation associated with impaired oxygenation and decreased perfusion to the brain. Use of accessory respiratory muscles is evident. The cardiovascular response is tachycardia to improve cardiac output as compensation for poor tissue oxygenation. These attempts to reduce hypoxia represent an adaptive sympathetic nervous system response and are likely to be ineffective because mediators are already circulating and triggering a cascade of systemic responses. TABLE 24-2 Integrated Assessment of the Patient With ARDS Stage Physical Examination Diagnostic Test Results Stage 1 Restlessness, dyspnea, ABG: Respiratory alkalosis (first 12 tachypnea CXR: No radiographic changes h) Moderate to extensive use of Chemistry: Blood results may vary accessory respiratory muscles depending on precipitating cause (e.g., elevated white blood cell count, changes in Hgb) Hemodynamics: Elevated PAP, normal or low PAOP Stage 2 Severe dyspnea, tachypnea, ABG: Decreased SaO 2 despite cyanosis, tachycardia supplemental oxygen administration (24 h) Coarse bilateral crackles CXR: Patchy bilateral infiltrates Decreased air entry to Chemistry: Increasing acidosis dependent lung fields (metabolic) depending on severity of Increased agitation and onset restlessness Hemodynamics: Increasingly elevated PAP, normal or low PAOP Stage 3 Decreased air entry bilaterally ABG: Worsening hypoxemia (2–10 Impaired responsiveness (may CXR: Air bronchograms, decreased d) be related to sedation lung volumes necessary to maintain Chemistry: Signs of other organ mechanical ventilation) involvement: decreased platelets and Decreased gut motility Hgb, increased white blood cell count, Generalized edema abnormal clotting factors Poor skin integrity and Hemodynamics: Unchanged or breakdown becoming increasingly worse Stage 4 Symptoms of MODS, including ABG: Worsening hypoxemia and (more decreased urine output, poor hypercapnia than gastric motility, symptoms of CXR: Air bronchograms, 10 d) impaired coagulation pneumothoraces OR Single-system involvement of Chemistry: Persistent signs of other the respiratory system with organ involvement: decreased gradual improvement over platelets and Hgb, increased white time blood cell count, abnormal clotting factors Hemodynamics: Unchanged or becoming increasingly worse ABG, arterial blood gases; CXR, chest radiograph; PAP, pulmonary artery pressure. As ARDS progresses, lung auscultation may reveal crackles secondary to an increase in secretions and narrowed airways; however, the bubbling crackles of cardiogenic pulmonary edema may be minimal. Assessment must be considered in the context of the presenting or initiating disease. For example, pneumonia, one risk factor for ARDS, may confound the ability to diagnose early-stage lung sound changes. The patient may be increasingly restless and confused secondary to hypoxia. Decreases in arterial oxygen saturation (SaO 2 ) are early signs of impending decompensation. The ability to compensate decreases with increasing pathologic changes. Dependent lung fields have decreased breath sounds as fluid accumulates and alveoli collapse. Agitation may give way to unresponsiveness, an ominous sign in which interventions to support ventilation and oxygenation are required quickly. Other later stages of progression result from tissue hypoxia and include dysrhythmias, chest pain, decreased renal function, and decreased bowel sounds. These are indications of multisystem involvement as highly perfused organ systems respond to decreased oxygen delivery with diminished function. Mechanical ventilatory support is required to maintain oxygenation in ARDS. Consolidation of the lungs with fluid reduces breath sounds. Lung compliance decreases, and increasing difficulties maintaining ventilation in the face of increasing resistance ensue. Changes in ventilation (such as decreased PaO 2 or increased peak inspiratory pressure) cannot be minimized because development of spontaneous pneumothoraces is a frequent complication of ARDS in the later stages. Transmitted sounds, poor air entry throughout all lung fields, and diffuse crackles coupled with ventilation make breath sounds difficult to assess. Cardiac output decreases despite persistent tachycardia because of inflammatory mediators, resulting in hypotension. Diagnostic Studies Throughout the stages of ARDS, the reliance on diagnostic tests is important (see Table 24-2 ). In the early stages, the need to establish cause may require specific tests, such as blood cultures, bronchoalveolar lavage cultures, and computed tomography (CT). As hypoxemia worsens, instability may preclude transport for diagnostic studies. In later stages, further vigilance is required to intervene for early management of any nosocomial infections. Ongoing monitoring of routine blood gas values, chemistry, and hematology is performed to ensure stability in metabolic parameters and optimization of existing function. Other laboratory studies are generally nonspecific and may include leukocytosis and lactic acidosis. Blood Gas Analysis Deterioration of arterial blood gas (ABG) values, despite interventions, is a hallmark of ARDS. Initially, hypoxemia (an arterial oxygen tension, or PaO 2 , of less than 60 mm Hg) may improve with supplemental oxygen; however, hypoxemia becomes refractory with a persistently low SaO 2. Early in acute respiratory failure, dyspnea and tachypnea are associated with a decreased PaCO 2 inducing respiratory alkalosis (pH greater than 7.45). As gas exchange and ventilation become increasingly impaired, carbon dioxide levels increase. Hypercarbia and elevated lactate from tissue hypoxia and anaerobic metabolism induced by hypoxemia result in a mixed respiratory and metabolic acidosis. Measurement of arterial lactate is commonly ordered as an indication of tissue hypoxia and anaerobic metabolism. Any elevated blood lactate concentration is common in early ARDS and resolves as oxygenation improves. Monitoring lactate levels can aid in ensuring adequate perfusion to tissues despite hypoxemia through manipulation of oxygen delivery, cardiac output, and Hgb. Base excess and deficit follow a similar trend, depending on the degree of tissue and organ hypoxia. Radiographic Studies In the early phase of ARDS, chest radiographic changes are usually negligible. Within a few days, the chest radiographic findings show patchy bilateral alveolar infiltrates, usually in the dependent lung fields. This may be mistaken for cardiogenic pulmonary edema. Over time, these patchy infiltrates progress to diffuse infiltrates, consolidation, and air bronchograms. CT of the chest also shows areas of infiltrates and consolidation of lung tissue. Daily chest radiographs are important in the continuing evaluation of the progression and resolution of ARDS and for ongoing assessment of potential complications, especially pneumothoraces. Intrapulmonary Shunt Measurement An intrapulmonary shunt is a type of ventilation–perfusion mismatch defined as the percentage of cardiac output that is not oxygenated owing to pulmonary blood flowing past collapsed or fluid-filled and nonventilated alveoli (a physiologic shunt), absence of blood flow to ventilated alveoli (alveolar dead space), or a combination of both of these conditions (silent unit [alveoli with no ventilation and no perfusion]; see Chapter 20 , Fig. 20-16 ). An intrapulmonary shunt of 3% to 5% is present in all people; however, advanced respiratory failure and ARDS are associated with a shunt of 15% or more because of changes in blood flow, endothelial disruption, and alveolar collapse. As the intrapulmonary shunt increases to 15% and greater, more aggressive interventions, including mechanical ventilation, are required because this level of shunt is associated with profound hypoxemia. Measurement of intrapulmonary shunt requires the use of a pulmonary artery catheter, which may be used in more severe cases. The intrapulmonary shunt fraction (Qs/Qt) is calculated using the arterial oxygen content (CaO 2 ), the mixed venous oxygen content (CvO 2 ), and the capillary oxygen content (CcO 2 ). Oxygen content is determined by Hgb, oxygen saturation (SO 2 ), and partial pressure of oxygen, measured by calculating the oxygen content in the pulmonary capillary bed, in the systemic arterial system, and in the mixed venous blood from the pulmonary artery. The intrapulmonary shunt fraction may also be estimated using the ratio of arterial oxygen to inspired oxygen (i.e., PaO 2 :FiO 2 ratio). In general, a PaO 2 :FiO 2 ratio greater than 300 is normal. Values less than 200 are associated with an intrapulmonary shunt of 15% to 20%, and a value of 100 or less is associated with an intrapulmonary shunt of more than 20%. Calculating the PaO 2 :FiO 2 ratio in patients on mechanical ventilation may be misleading, especially with high levels of support. A new formula that incorporates positive end-expiratory pressure (PEEP) may reflect true illness severity and estimate mortality 15 : 10[(PaO 2 × 10) / (FiO 2 × PEEP)] Lung Compliance, Airway Resistance, and Pressures Lung compliance, or distensibility, decreases as the alveoli fill with fluid or collapse. More effort and greater pressure are required to move air into the lungs as they become increasingly “stiff.” In addition, the resistance to airflow into and out of the lungs increases with the accumulation of secretions and mediator-induced bronchoconstriction. Because the patient with ARDS requires mechanical ventilation, lung compliance and airway resistance can be evaluated by assessing ventilator pressures and tidal volume changes. Increases in these pressures as tidal volumes are maintained to achieve a normal PaCO 2 indicate reduced compliance and increased resistance to airflow. As airway pressures rise, the lung epithelium is traumatized, resulting in further lung tissue damage. Volutrauma (lung epithelial damage) from persistently elevated airway pressures thus has additional deleterious effects on ventilation and oxygenation. Management Therapeutic modalities to actually treat ARDS have remained elusive. Although there are multiple potential causes of ARDS, management principles are similar. Treatment is supportive; that is, contributing factors are minimized, corrected, or reversed, and although the lungs heal, care is taken so that treatment does no further damage. In addition, extensive work has gone into creating “bundles,” which are elements of care considered core to the management and treatment of specific critical illnesses in ICUs. Box 24-2 lists essential critical care bundles that apply to managing ARDS. These treatments span prevention at early stages of disease onset, such as early goal- directed fluid resuscitation and longer-term prevention of complications, such as sedation protocols. Regardless, one of the most important roles for critical care nurses is ensuring attention to all these elements to prevent mortality and complications and to promote recovery. BOX 24-2 Care “Bundles” in Critical Care Ventilator-associated pneumonia “bundle” basics Elevated head of the bed 30 to 45 degrees Daily weaning assessment (spontaneous breathing trials) Daily sedation withholding Weaning protocol Deep vein thrombosis (DVT) prophylaxis Peptic ulcer prophylaxis Sepsis “bundle” basics Appropriate antibiotic therapy Early goal-directed fluid resuscitation Steroid administration Activated protein C DVT prophylaxis Peptic ulcer prophylaxis Other protocols that may be added Tight glucose control Postpyloric feeding Subglottic suctioning Electrolyte replacement Oxygenation and Ventilation Oxygen Delivery Patients with ALI and ARDS develop refractory hypoxemia. Strategies have attempted to optimize normal oxygen delivery parameters, including Hgb, cardiac output, and SO 2. Oxygen delivery (DaO 2 ), as determined by Hgb, arterial oxygenation, and cardiac output, is the amount of oxygen delivered to the tissues every minute. Adequate DaO 2 (greater than 800 mL O 2 /min) is essential to meet tissue requirements for oxygen, thereby preventing anaerobic metabolism and hypoxia, which can trigger and perpetuate SIRS. Patients who are critically ill with ARDS have high demands for oxygen to maintain organ function. Sufficient amounts of Hgb are necessary to carry oxygen to the cells. There is little research to support the intuitive concept that normal or increased Hgb is required to promote oxygen delivery in patients with SIRS or ARDS. Studies on transfusion requirements indicate that values of approximately 8.0 g/dL are sufficient for patients who are critically ill, except for those with cardiac disease. 16 Cardiac output may be altered in ARDS because of SIRS, the effect of hypoxemia on the myocardium, and the decrease in venous return induced by mechanical ventilation. Evaluation of the cardiac output is important so that oxygen delivery can be assessed and appropriate interventions initiated. Therapies to optimize cardiac output are directed toward enhancing preload and contractility and normalizing afterload. The use of a thermodilution pulmonary artery catheter to assess oxygen delivery and consumption for patients with ARDS is rare, but may be used to ensure that appropriate interventions are instituted. There are other less invasive methods to measure cardiac output utilizing the patient’s transduced arterial blood pressure, as well as utilizing the patient’s central venous catheter to measure the venous oxygen content. These methods provide data that can be trended over time without the need for placement of additional catheters. Fluid management has been used for many years in an effort to balance the type of fluid needed to manage the hallmark edema and decompensation associated with ARDS. At the onset of the disease, early goal-directed fluid resuscitation is recommended. Diuretics and reduced fluid administration have been studied to reduce lung edema. Conservative fluid management with diuresis is associated with modest improvements in oxygenation and lower hospital mortality. 17 Positive inotropic agents, such as dobutamine or milrinone, are used to enhance contractility and increase cardiac output; however, care must be taken with these agents as they may cause systemic vasodilation worsening hypotension. Vasoconstrictors, such as norepinephrine, may be added to the therapies to counteract the vasodilation induced by SIRS. Vasoconstricting agents must be administered cautiously because many vascular beds, especially in the lungs, are constricted, also as a result of SIRS mediators and hypoxia. Patients receiving inotropic or vasoactive medications require continuous arterial blood pressure monitoring and may require evaluation of cardiac output and other hemodynamic measures. Mechanical Ventilation Methods to deliver appropriate levels of oxygen and allow for removal of carbon dioxide include types of mechanical ventilation and positioning. Lung-protective ventilation strategies limit ventilator-induced lung injury (VILI); these include low tidal volumes (less than 6 mL/kg predicted body weight), the use of adequate PEEP to reduce the risk of using a high FiO 2 and precipitating oxygen toxicity, and limiting plateau pressures to 30 cm H 2 O. 18 Multiple modes of mechanical ventilation are available to support the patient with respiratory failure. (See Chapter 22 for a complete discussion of mechanical ventilation.) In general, the principle of “do no harm” includes use of the lowest FiO 2 to achieve adequate oxygenation and use of small tidal volumes to minimize airway pressures, thus preventing or reducing lung damage (volutrauma). Permissive hypercapnia may be necessary to prevent an increased respiratory rate in the face of lower tidal volumes. PEEP prevents collapse and recruits alveoli, allowing diffusion of gases across the alveolar-capillary membrane. Recommended values for PEEP are 10 to 15 cm H 2 O, but values in excess of 20 cm H 2 O are acceptable to reduce inspired oxygen requirements or maintain adequate oxygenation. 18 Permissive hypercapnia is a strategy that allows the PaCO 2 to rise slowly above normal through reduction of tidal volume, therefore limiting the plateau and peak airway pressures. A PaCO 2 between 55 and 60 mm Hg and a pH of 7.25 to 7.35 are tolerated when achieved gradually. It is necessary to monitor the increase in PaCO 2 to prevent too rapid a rise, and overall values should be no greater than 80 to 100 mm Hg because of the potential effects on cardiopulmonary function. These techniques are not used for patients with cardiac or neurologic involvement. Several modes of mechanical ventilation are directed toward minimizing airway pressures and iatrogenic lung injury, associated with conventional volume-controlled mechanical ventilation. Pressure-controlled ventilation limits the peak inspiratory pressure to a set level (as opposed to volume-controlled ventilation, which delivers a set tidal volume despite the pressure required to move the set volume into the lungs). Pressure-controlled ventilation also uses a decelerating inspiratory airflow pattern to minimize the peak pressure while delivering the necessary tidal volume. Patients on pressure-controlled ventilation mode may require sedation to prevent dyssynchrony with the ventilator. Novel Ventilation Strategies Inverse-ratio ventilation is another strategy thought to improve alveolar recruitment. Reversal of the normal inspiratory–expiratory ratio (I:E ratio) to 2:1 or 3:1 prolongs inspiration time, preventing complete exhalation. An inverse I:E ratio is achieved through manipulation of the mechanical ventilator. This increased end- expiratory volume creates auto-PEEP (intrinsic PEEP) that is added to the applied extrinsic PEEP. The theoretical advantages include reduced alveolar pressures and overall PEEP levels. This therapy requires sedation and paralytics to improve tolerance. Airway pressure release ventilation (APRV) similarly inverses the I:E ratio but with the advantage of allowing the patient to initiate breaths. These patients do not require the same level of sedation or paralysis to achieve pressure-limited ventilation and may have improved recruitment of alveoli. High-frequency oscillatory ventilation (HFOV) uses very low tidal volumes (1 to 4 mL/kg) delivered at rates of 3 to 15 Hz or cycles/s rather than breaths/min, resulting in lower airway pressures and reduced volutrauma. Deleterious effects of HFOV include increased trapping of air in the alveoli (auto-PEEP) and increased mean airway pressures to high levels in some patients. HFOV requires sedation and paralysis as any change in airway pressure will cause oscillation to cease. Although there is evidence demonstrating the safety of this approach and improvements in oxygenation, there is no evidence from randomized trials demonstrating mortality benefits over volume assist control (A/C), the primary mode used in major ARDS trials, and one study was associated with increased in-hospital mortality. 19 , 20 Partial ventilatory modes with preserved spontaneous breathing activity during mechanical ventilation have gained use in patients with ARDS. These modes may include A/C, synchronized intermittent mandatory ventilation, pressure support ventilation, proportional assist ventilation, APRV, biphasic positive airway pressure, or neurally adjusted ventilatory assist. Traditionally, these modes were reserved for use in weaning patients from mechanical ventilation, but are now used in all phases of mechanical ventilation. These modes adjust the degree of mechanical support provided to patients, preserving diaphragmatic contraction and allowing spontaneous breathing efforts. It is unclear whether partial ventilatory support modalities improve survival, but they have been shown to alleviate patient- ventilator asynchrony more efficiently than others and decrease the use of sedatives and neuromuscular blockade. Additional studies are needed to support the use of these modes in ARDS. Extracorporeal Therapies Extracorporeal lung support (ECLS) technology involves the use of large vascular cannulas to remove blood from the patient. A pumping device and circuit circulate the blood, and one or two “artificial lungs” remove carbon dioxide and oxygenate the blood. Extracorporeal membrane oxygenation (ECMO) and extracorporeal carbon dioxide removal may potentially be effective in managing ARDS. These highly invasive, high-risk technologies allow the lungs to “rest,” because near-apneic ventilation or ventilation with small tidal volumes and slow respiratory rates greatly reduce airway pressures while gas exchange takes place in the artificial membrane lungs. ECLS has gained considerable attention following the 2009 H1N1 influenza and COVID-19 pandemics. In patients with these diseases, ECMO has been used as rescue oxygenation therapy. Several randomized clinical trials and observational studies suggested that ECMO associated with protective mechanical ventilation could improve outcome, but its efficacy remains uncertain. Technologic advances have improved the size, safety, and simplicity of ECLS and may lead to an important advance in the management and outcome of patients with ARDS. Rigorous evidence on the optimal timing, disease characteristics, and indications for ECLS in patients with severe ARDS and its ability to improve short- and long-term outcomes are needed. ECLS should be considered for patients with life-threatening hypoxemia or hypercapnia refractory to conventional mechanical ventilation. 19 , 21 – 25 ECLS may generate ethical concerns within the care team. Goal-directed care with a clear plan should be developed at the outset with the care team, patient (when able), and their family. Positioning Frequent position change is well established as a means to prevent and reverse atelectasis and to facilitate removal of secretions from the airways. Although not a treatment for ARDS, elevating the head of the bed greater than 30 degrees is considered necessary care for preventing ventilator-associated pneumonia (VAP). Prone positioning in the patient’s bed, using a Stryker frame or RotoProne therapy system, improves pulmonary gas exchange, facilitates pulmonary drainage in the dorsal lung regions, and aids resolution of consolidated dependent alveoli (in the supine position), particularly in the dorsal lung regions. The evidence for the effectiveness of prone positioning, now a common intervention with ARDS, is variable. Data indicate that carefully performed prone positioning offers a survival advantage of 13%. Although there was no significant difference, it remains a recommended intervention in this specific population. 26 , 27 Prone positioning prior to mechanical ventilation has been found to improve mortality in those with COVID-19-related ARDS but has not shown a reduction in the need for invasive mechanical ventilation. 28 Associated risks include loss of airway control through accidental extubation, loss of vascular access, facial edema and development of pressure areas, and difficulties with cardiopulmonary resuscitation. Recommendations on the steps involved in prone positioning appear in Box 24-3. BOX 24-3 Summary of Key Steps to Consider for Prone Positioning 1. Evaluate with the interdisciplinary team the patient’s condition and determine whether a trial of prone positioning is warranted. 2. Organize the team to ensure familiarity with the procedure and patient care while prone. Use your hospital’s evidence-based procedure. Equipment onsite Assign and clarify team roles during prone positioning. 3. Prepare the patient for the procedure. Provide explanation to patient and family. Consider insertion of feeding tube, nasogastric tube, or both as necessary. 4. Assess and document the patient’s pre–prone positioning status. Hemodynamic and ventilatory parameters, skin or wound condition, and so forth 5. Protect and maintain the patient’s airway. Secure endotracheal tube. Apply inline suction if not already in place. 6. Use safety precautions to ensure body position will be maintained during the prone positioning procedure. 7. Administer adequate sedation and analgesic medication. 8. Complete the procedure as per protocol. Note: Risks for inadvertent extubation or line displacement are high during the procedure. 9. Assess, evaluate, and monitor the patient’s condition. 10. Implement preventive care for pressure areas, eyes, and skin. Pharmacologic Therapy Antibiotic therapy is appropriate in the presence of a known microorganism but should not be used prophylactically. The signs of SIRS are similar to those of infection (i.e., tachycardia, fever, increased white blood cell count), thus creating the temptation to treat with antimicrobial therapy. It is essential to identify a source of infection (isolation of specific bacteria through blood, wound, pulmonary, and other cultures) before initiating antibiotics. Prophylactic antibiotic therapy has not been shown to improve outcome. Emphasis is on prevention of infection, especially nosocomial infection related to the use of invasive vascular catheters and ventilators (e.g., VAP). Bronchodilators and mucolytics are useful in ARDS to assist in maintaining airway patency and reducing the inflammatory reaction and accumulation of secretions in the airways. The response to therapy is evaluated by monitoring airway resistance and pressures and lung compliance. Administration of intravenous corticosteroids at low doses has been shown to improve survival and reduce morbidity in patients with ARDS; however, the results from recent randomized controlled trials failed to show improved outcomes. Early use of high-dose corticosteroids may increase mortality and cause adverse effects in patients with ARDS and is therefore not recommended. The effectiveness of corticosteroid treatment may largely depend on the underlying diseases and the timing during the course of ARDS. 32 Nitric oxide is an inhaled gas that causes selective pulmonary vasodilation and therefore reduces the deleterious effects of pulmonary hypertension. To date, nitric oxide has not been shown to improve mortality or oxygenation beyond the first 24 hours of therapy. Nitric oxide should be reserved for those patients with life- threatening refractory hypoxemia after mechanical ventilation has been maximized. Inhaled prostacyclin has also produced pulmonary vasodilation similar to nitric oxide and may be considered. Sedation Effective use of sedation to promote comfort and reduce respiratory effort, thus decreasing oxygen demand, is an important consideration for nurses caring for patients with ARDS. Neuromuscular blocking agents (NMBAs) and general anesthetics, such as propofol, although not sedatives, are frequently used in these patients to facilitate patient-ventilator synchrony, decreasing the work of breathing and facilitating ventilation, especially when high airway pressures or prone position is applied. Improved oxygenation and reduction in barotrauma have been associated with NMBAs; however, they were not found to reduce ventilator days, duration of mechanical ventilation, or mortality. 29 , 30 NMBAs require concurrent use of sedation to prevent patients who are chemically paralyzed from being alert but unable to move. Frequent assessment of adequacy of both neuromuscular blockade and sedation is an important nursing intervention. NMBAs have been associated with critical illness polyneuropathy and polymyopathy, especially when concurrently administered with corticosteroids. Pain, anxiety, and delirium are all possible reasons for needing pharmacologic treatment, and it is important to distinguish among them because each requires a different pharmacologic intervention. It is vital to understand why each is being given, what the goals of therapy are, and what the long-term implications of overuse can be. These considerations are balanced with the need to decrease oxygen demand and provide comfort for patients requiring intensive ventilation management and undergoing potentially uncomfortable procedures. Nutritional Support Early initiation of nutritional support is essential for patients with ARDS because nutrition plays an active therapeutic role in recovery from critical illness. There are two major theoretical reasons to use early enteral feeding as a therapeutic intervention in SIRS and ARDS. Mediators (TNF-alpha and IL-1 in particular) stimulate release of proteolytic enzymes that stimulate protein catabolism from skeletal muscle. Persistent protein loss is compounded by interstitial loss through capillary leak and downregulation of messenger RNA production of intravascular proteins, such as albumin. Earlier in this chapter, reference was made to changes in circulatory patterns resulting from hypoxic sympathetic nervous system reactions. In this way, there is decreased perfusion to the gut. After resuscitation, increases in release of neutrophils further damage the injured, reperfused colon through increased vascular endothelial permeability, thus releasing normal gut bacteria into the systemic circulation and leading to increases in the incidence of peritonitis, pneumonia, and sepsis. The mechanism through which enteral feeding improves outcomes remains unproved, but the reduction in mortality in patients who are critically ill and receive enteral feedings indicates that this practice is of general benefit. A diet with a balanced caloric, protein, carbohydrate, and fat intake is calculated based on metabolic needs, with particular attention paid to specific amino acids, lipid, and carbohydrate intake. Patients with SIRS or ARDS usually require 35 to 45 kcal/kg/d. High- carbohydrate solutions are avoided to prevent excess carbon dioxide production. Recent innovations in amino acid supplementation are being reviewed because of the role of amino acids in the immune response. The role of antioxidants and omega-3 fatty acids is still being investigated as to their use in improving outcomes in ARDS patients. 31 The problem facing the practitioner is the ability to deliver enteral nutrition in the face of decreased gut motility. Insertion of small bowel feeding tubes may be considered. The role of total parenteral nutrition is controversial, and some clinicians rarely use it, either alone or in combination with enteral nutrition. The risk for aspiration associated with enteral feeding needs to be appreciated, and careful monitoring of absorption and gut function is essential. A collaborative care guide for the patient with ARDS is given in Box 24-4. BOX 24-4 COLLABORATIVE CARE GUIDE for the Patient With ARDS Outcomes Interventions Oxygenation/Ventilation Patent airway will be maintained. A Auscultate breath sounds every 2–4 h and PaO 2 :FiO 2 ratio of 200–300 or as required. more will be maintained if Intubate to maintain oxygenation and possible. ventilation and to decrease work of breathing. Suction endotracheal airway when appropriate (see Chapter 22 , Box 22-15 , “Collaborative Care Guide for the Patient on Mechanical Ventilation”). Hyperoxygenate before and after each suction pass. Lung-protective ventilation Monitor airway pressures every 1–2 h. strategies will be used. Maintain Monitor airway pressures after suctioning. a low tidal volume (