Week 1-5 Biochemistry PDF
Document Details
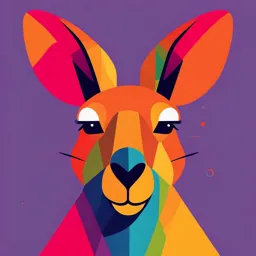
Uploaded by ExcitedSupernova
Our Lady of Fatima University
Tags
Summary
This document covers organic chemistry concepts, including hydrocarbons, isomers, nomenclature, and related topics. It's suitable for an undergraduate biochemistry course.
Full Transcript
Lesson Proper for Week 1 WHAT IS BIOCHEMISTRY? The ultimate goal of biochemistry is to explain all life processes in molecular detail. Because life processes are performed by organic molecules the discipline of biochemistry relies heavily on fundamental principles of organic chemistry and other bas...
Lesson Proper for Week 1 WHAT IS BIOCHEMISTRY? The ultimate goal of biochemistry is to explain all life processes in molecular detail. Because life processes are performed by organic molecules the discipline of biochemistry relies heavily on fundamental principles of organic chemistry and other basic sciences. ORGANIC COMPOUNDS Hydrocarbon is an organic chemical compound composed exclusively of hydrogen and carbon atoms. Hydrocarbons are naturally-occurring compounds and form the basis of crude oil, natural gas, coal, and other important energy sources. TYPES OF HYDROCARBON 1. ALKANE- Alkanes, hydrocarbons in which all the bonds are single, have molecular formulas that satisfy the general expression CnH2n + 2 (where n is an integer). Carbon is sp3 hybridized (three electron pairs are involved in bonding, forming a tetrahedral complex), and each C—C and C—H bond is a sigma (σ) bond (see chemical bonding). In order of increasing number of carbon atoms, methane (CH4), ethane (C2H6), and propane (C3H8) are the first three members of the series. a. ISOMERS- Different compounds that have the same molecular formula are called isomers. Isomers that differ in the order in which the atoms are connected are said to have different constitutions and are referred to as constitutional isomers. b. NOMENCLATURE- Naming of organic compounds is facilitated through the use of formal systems of nomenclature. The most widely used standards for organic nomenclature evolved from suggestions made by a group of chemists assembled for that purpose in Geneva in 1892 and have been revised on a regular basis by the International Union of Pure and Applied Chemistry (IUPAC). The IUPAC rules assign names to unbranched alkanes according to the number of their carbon atoms. The n- prefix is not used for unbranched alkanes in systematic IUPAC nomenclature. Beginning with five-carbon chains, the names of unbranched alkanes consist of a Latin or Greek stem corresponding to the number of carbons in the chain followed by the suffix -ane. c. CYCLOALKANE- Countless organic compounds are known in which a sequence of carbon atoms, rather than being connected in a chain, closes to form a ring. Saturated hydrocarbons that contain one ring are referred to as cycloalkanes. With a general formula of CnH2n (n is an integer greater than 2), they have two fewer hydrogen atoms than an alkane with the same number of carbon atoms. Cyclopropane (C3H6) is the smallest cycloalkane, whereas cyclohexane (C6H12) is the most studied, best understood, and most important. 2. ALKENE AND ALKYNE- Alkenes (also called olefins) and alkynes (also called acetylenes) belong to the class of unsaturated aliphatic hydrocarbons. Alkenes are hydrocarbons that contain a carbon-carbon double bond, whereas alkynes have a carbon-carbon triple bond. Alkenes are characterized by the general molecular formula CnH2n, alkynes by CnH2n − 2. Ethene (C2H4) is the simplest alkene and ethyne (C2H2) the simplest alkyne. a. NOMENCLATURE- Higher alkenes and alkynes are named by counting the number of carbons in the longest continuous chain that includes the double or triple bond and appending an -ene (alkene) or -yne (alkyne) suffix to the stem name of the unbranched alkane having that number of carbons. The chain is numbered in the direction that gives the lowest number to the first multiply bonded carbon, and adding it as a prefix to the name. Once the chain is numbered with respect to the multiple bond, substituents attached to the parent chain are listed in alphabetical order and their positions identified by number. BENZENE AND IT’S DERIVATIVES Benzene is an organic chemical compound with the molecular formula C6H6. The benzene molecule is composed of six carbon atoms joined in a planar ring with one hydrogen atom attached to each. a. NOMENCLATURE- Some common substituents, like NO2, Br, and Cl, can be named this way when it is attached to a phenyl group. Long chain carbons attached can also be named this way. The general format for this kind of naming is: (positions of substituents (if >1)- + # (di, tri,...) + substituent)n + benzene. For example, chlorine (Cl) attached to a phenyl group would be named chlorobenzene (chloro + benzene). Since there is only one substituent on the benzene ring, we do not have to indicate its position on the benzene ring. Below is a list of commonly seen benzene-derived compounds. Some of these mono- substituted compounds (labeled in red and green). ALCOHOLS, ETHERS AND THIOLS a. ALCOHOL- An alcohol is an organic compound that contains the hydroxyl group ( – OH). Alcohols are classified according to the number of other carbon atoms that are directly bonded to the carbon atom attached to the hydroxyl group. An alcohol is named by replacing the final -e of the parent hydrocarbon with -ol. The name includes the number of the carbon atom to which the hydroxyl group is attached.If the chain also has hydrocarbon or halide constituents, the lowest number is assigned to the carbon atom with the hydroxyl group. b. ETHERS- An organic compound containing an oxygen atom between 2 carbon atoms in a chain. Ethers can be synthesized from the reaction of alcohols. When the alcohols react, a molecule of water is produced in a condensation reaction. The IUPAC method to name an ether is to add the suffix -oxy to the smaller hydrocarbon group that is bonded to the larger alkane group. For example, an ether made up of a methyl group and an ethyl group joined by an oxygen molecule is called methoxyethane. c. THIOLS- A thiol is an organic compound that includes the sulfhydryl functional group, –SH. To name thiols, the suffix -thiol is added to the end of the alkane name. For example, HSCH 3 is methanethiol. AMINES An amine is a derivative of ammonia (NH3) in which one or more of the hydrogen atoms are replaced with a substituent groups such as an alkyl or aryl groups. A primary amine would be named by adding the -amine suffix to the name of the chain or ring to which it is attached. The -e is removed from this name. ALDEHYDES AND KETONES Aldehydes and ketones are organic compounds which incorporate a carbonyl functional group, C=O. The carbon atom of this group has two remaining bonds that may be occupied by hydrogen or alkyl or aryl substituents. If at least one of these substituents is hydrogen, the compound is an aldehyde. If neither is hydrogen, the compound is a ketone. The IUPAC system of nomenclature assigns a characteristic suffix -al to aldehydes. For example, H2C=O is methanal, more commonly called formaldehyde. The IUPAC system of nomenclature assigns a characteristic suffix of -one to ketones. A ketone carbonyl function may be located anywhere within a chain or ring, and its position is usually given by a location number. CARBOXYLIC ACID A carboxylic acid is an organic compound that contains a carboxyl group, –COOH. Carboxyl group is a carbon atom that is double-bonded to 1 oxygen atom and single-bonded to a hydroxyl group. To name organic compounds containing a carboxylic group, start with the alkane name for the longest chain, including the carbon atom in the carboxyl group. Drop the -e suffix of the root compound and replace it with the suffix -oic, followed by the word acid. Lesson Proper for Week 2 ORGANIC REACTION Organic reactions are the chemical reactions that are undergone by organic compounds. A few important types of organic reactions are illustrated below. There is no limit to the number of possible organic reactions and mechanisms. However, certain general patterns are observed that can be used to describe many common or useful reactions. Each reaction has a stepwise reaction mechanism that explains how it happens, although this detailed description of steps is not always clear from a list of reactants alone. Organic reactions can be organized into several basic types. Some reactions fit into more than one category. For example, some substitution reactions follow an addition-elimination pathway. TYPES OF ORGANIC REACTION 1. ADDITION REACTION- An addition reaction, in organic chemistry, is in its simplest terms an organic reaction where two or more molecules combine to form a larger one. Addition reactions are limited to chemical compounds that have multiple bonds, such as molecules with carbon– carbon double bonds (alkenes), or with triple bonds (alkynes), and compounds that have rings, which are also considered points of unsaturation. Molecules containing carbon-hetero double bonds like carbonyl (C=O) groups, or imine (C=N) groups, can undergo addition, as they too have double-bond character. There are two main types of polar addition reactions: electrophilic addition and nucleophilic addition. Two non- polar addition reactions exist as well, called free-radical addition and cycloadditions. Addition reactions are also encountered in polymerizations and called addition polymerization. 2. ELIMINATION REACTION- An elimination reaction is a type of organic reaction in which two substituents are removed from a molecule in either a one- or two-step mechanism.There are some reactions which involve the elimination or removal of the adjacent atoms. After these multiple bonds are formed and there is a release of small molecules as products. It is the principal process by which organic compounds containing only single carbon-carbon bonds (saturated compounds) are transformed to compounds containing double or triple carbon-carbon bonds (unsaturated compounds). E2 MECHANISM. An example of this type of reaction in scheme 1 is the reaction of isobutylbromide with potassium ethoxide in ethanol. The reaction products are isobutene, ethanol and potassium bromide. E1 MECHANISM. An example in scheme 2 is the reaction of tert-butylbromide with potassium ethoxide in ethanol. 3. SUBSTITUTION REACTION- A substitution reaction (also known as single displacement reaction or single substitution reaction) is a chemical reaction during which one functional group in a chemical compound is replaced by another functional group. Substitution reactions in organic chemistry are classified either as electrophilic or nucleophilic depending upon the reagent involved, whether a reactive intermediate involved in the reaction is a carbocation, a carbanion or a free radical, and whether the substrate is aliphatic or aromatic. Detailed understanding of a reaction type helps to predict the product outcome in a reaction. It also is helpful for optimizing a reaction with regard to variables such as temperature and choice of solvent. (chlorination of methane by chlorine) 4. REARRANGEMENT REACTION- A rearrangement reaction is a broad class of organic reactions where the carbon skeleton of a molecule is rearranged to give a structural isomer of the original molecule. Often a substituent moves from one atom to another atom in the same molecule. A rearrangement is a reaction in which the reactant and the products are constitutional isomers and the reactant is converted to the product by the migration of one or more ligands from one point of the reactant to another. 5. ORGANIC REDOX REACTION- Redox reactions are oxidation-reduction chemical reactions in which the reactants undergo a change in their oxidation states. The term ‘redox’ is a short form of reduction-oxidation. All the redox reactions can be broken down into two different processes – a reduction process and an oxidation process. The oxidation and reduction reactions always occur simultaneously, such class of chemical reactions was named as the redox reaction. The substance getting reduced in a chemical reaction is known as the oxidizing agent, while a substance that is getting oxidized is known as the reducing agent. esson Proper for Week 3 WHAT IS CARBOHYDRATE? A carbohydrate is a biomolecule consisting of carbon (C), hydrogen (H) and oxygen (O) atoms, usually with a hydrogen–oxygen atom ratio of 2:1 as in water and thus with the empirical formula Cm(H2O)n (where m may or may not be different from n). However, not all carbohydrates conform to this precise stoichiometric definition, nor are all chemicals that do conform to this definition automatically classified as carbohydrates. All carbohydrates consist of carbon, hydrogen, and oxygen atoms and are polyhydroxy aldehydes or ketones or are compounds that can be broken down to form such compounds. Examples of carbohydrates include starch, fiber, the sweet-tasting compounds called sugars, and structural materials such as cellulose. The term carbohydrate had its origin in a misinterpretation of the molecular formulas of many of these substances. For example, because its formula is C6H12O6, glucose was once thought to be a “carbon hydrate” with the structure C 6·6H2O. THE MAJOR DIETARY CARBOHYDRATES Class Subgroup Components (degree of polymerization) Sugars Monosaccharides Glucose, galactose, fructose, xylose Disaccharides Sucrose, lactose, maltose, isomaltulose, trehalose Polyols Sorbitol, mannitol Oligosaccharides Malto- Maltodextrins oligosaccharides Other Raffinose, stachyose, fructo-oligosaccharides oligosaccharides Polysaccharides Starch Amylose, amylopectin, modified starches Non-starch Glycogen, Cellulose, Hemicellulose, Pectins, Hydrocolloids polysaccharides CLASSIFICATION OF CARBOHYDRATES 1. MONOSACCHARIDES Monosaccharide, also called simple sugar, any of the basic compounds that serve as the building blocks of carbohydrates. Monosaccharides are polyhydroxy aldehydes or ketones; that is, they are molecules with more than one hydroxyl group (―OH), and a carbonyl group (C=O) either at the terminal carbon atom (aldose) or at the second carbon atom (ketose). Monosaccharides are classified by the number of carbon atoms in the molecule; dioses have two, trioses have three, tetroses four, pentoses five, hexoses six, and heptoses seven. Most contain five or six. The most- important pentoses include xylose, found combined as xylan in woody materials; arabinose from coniferous trees; ribose, a component of ribonucleic acids (RNA) and several vitamins; and deoxyribose, a component of deoxyribonucleic acid (DNA). Among the most-important aldohexoses are glucose, mannose, and galactose; fructose is a ketohexose. CYCLIC STRUCTURE OF MONOSACCHARIDES You might wonder why the aldehyde reacts with the OH group on the fifth carbon atom rather than the OH group on the second carbon atom next to it. Recall that cyclic alkanes containing five or six carbon atoms in the ring are the most stable. The same is true for monosaccharides that form cyclic structures: rings consisting of five or six carbon atoms are the most stable. REACTIONS OF MONOSACCHARIDES a. Ester and Ether Formation The -OH groups on a monosaccharide can be readily converted to esters and ethers. Esterfication can be done with an acid chloride or acid anhydride, while treatment with an alkyl halide by a Williamson ether synthesis leads to the ether. b. Glycoside Formation Acetal derivatives formed when a monosaccharide reacts with an alcohol in the presence of an acid catalyst are called glycosides. Glycosides abound in biological systems. By attaching a sugar moiety to a lipid or benzenoid structure, the solubility and other properties of the compound may be changed substantially. c. Phosphorylation The most common ionic group in biologically important organic compounds is phosphate, thus the phosphorylation of alcohol groups is a critical metabolic step. In alcohol phosphorylations, ATP is almost always the phosphate donor, and the mechanism is very consistent: the alcohol oxygen acts as a nucleophile, attacking the gamma-phosphorus of ATP and expelling ADP d. Oxidation Sugars may be classified as reducing or non-reducing based on their reactivity with Tollens', Benedict's or Fehling's reagents. If a sugar is oxidized by these reagents it is called reducing, since the oxidant (Ag(+) or Cu(+2)) is reduced in the reaction, as evidenced by formation of a silver mirror or precipitation of cuprous oxide. The Tollens' test is commonly used to detect aldehyde functions; and because of the facile interconversion of ketoses and aldoses under the basic conditions of this test, ketoses such as fructose also react and are classified as reducing sugars. e. Reduction Sodium borohydride reduction of an aldose makes the ends of the resulting alditol chain identical, HOCH2(CHOH)nCH2OH, thereby accomplishing the same configurational change produced by oxidation to an aldaric acid. Thus, allitol and galactitol from reduction of allose and galactose are achiral, and altrose and talose are reduced to the same chiral alditol. 2. DISACCHARIDES A disaccharide, also called a double sugar or biose is the sugar formed when two monosaccharides are joined by glycosidic linkage. Like monosaccharides, disaccharides are simple sugars soluble in water. disaccharides differ from one another in their monosaccharide constituents and in the specific type of glycosidic linkage connecting them. There are three common disaccharides: maltose, lactose, and sucrose. All three are white crystalline solids at room temperature and are soluble in water. The joining of monosaccharides into a double sugar happens by a condensation reaction, which involves the elimination of a water molecule from the functional groups only. Breaking apart a double sugar into its two monosaccharides is accomplished by hydrolysis with the help of a type of enzyme called a disaccharidase. As building the larger sugar ejects a water molecule, breaking it down consumes a water molecule. 3. OLIGOSACCHARIDES An oligosaccharide is a saccharide polymer containing a small number typically three to ten of monosaccharides (simple sugars). Oligosaccharides can have many functions including cell recognition and cell binding. For example, glycolipids have an important role in the immune response. GLYCOPROTEINS Membrane proteins are often covalently linked to oligosaccharides, which are branched glycoside-linked sugars (averaging around 15 sugar residues). As glycans, they are the sugars linked to glycoproteins. Glycoproteins are rare in the cytosol, but common on secreted and membrane proteins. Sugars are commonly attached to proteins in a process called glycosylation. Typically the attachment is to a hydroxyl or other functional group. The majority of proteins synthesized in the endoplasmic reticulum are glycosylated. 4. POLYSACCHARIDES Polysaccharides or polycarbohydrates, are the most abundant carbohydrate found in food. They are long chain polymeric carbohydrates composed of monosaccharide units bound together by glycosidic linkages. This carbohydrate can react with water using amylase enzymes as catalyst, which produces constituent sugars (monosaccharides, or oligosaccharides). They range in structure from linear to highly branched. Examples include storage polysaccharides such as starch, glycogen and galactogen and structural polysaccharides such as cellulose and chitin. The polysaccharides are the most abundant carbohydrates in nature and serve a variety of functions, such as energy storage or as components of plant cell walls. STARCH Starch is the most important source of carbohydrates in the human diet and accounts for more than 50% of our carbohydrate intake. Starch is a mixture of two polymers: amylose and amylopectin. Natural starches consist of about 10%–30% amylose and 70%–90% amylopectin. Amylose is a linear polysaccharide composed entirely of D- glucose units joined by the α-1,4-glycosidic linkages we saw in maltose Amylose is a linear chain of α-D-glucose units joined together by α-1,4-glycosidic bonds GLYCOGEN Glycogen is the energy reserve carbohydrate of animals. Practically all mammalian cells contain some stored carbohydrates in the form of glycogen, but it is especially abundant in the liver (4%–8% by weight of tissue) and in skeletal muscle cells (0.5%–1.0%). Like starch in plants, glycogen is found as granules in liver and muscle cells. When fasting, animals draw on these glycogen reserves during the first day without food to obtain the glucose needed to maintain metabolic balance. CELLULOSE Cellulose is an organic compound with the formula (C6H10O5)n, a polysaccharide consisting of a linear chain of several hundred to many thousands of β(1→4) linked D-glucose units. Cellulose is an important structural component of the primary cell wall of green plants, many forms of algae and the oomycetes. CHITIN Chitin is one of many naturally occurring polymers. It forms a structural component of many animals, such as exoskeletons. Over time it is bio-degradable in the natural environment. Its breakdown may be catalyzed by enzymes called chitinases, secreted by microorganisms such as bacteria and fungi, and produced by some plants. Some of these microorganisms have receptors to simple sugars from the decomposition of chitin. If chitin is detected, they then produce enzymes to digest it by cleaving the glycosidic bonds in order to convert it to simple sugars and ammonia. Lesson Proper for Week 4 PROTEINS Proteins are large size macromolecules, polymers of structural units called amino acids. A total of 20 different amino acids exist in proteins and hundreds to thousands of these amino acids are attached to each other in long chains to form a protein. Amino acids can be released from proteins by hydrolysis. Hydrolysis is the cleavage of a covalent bond by addition of water in adequate conditions. FUNCTIONS OF PROTEINS Proteins perform essential functions throughout the systems of the human body. In the respiratory system, hemoglobin (composed of four protein subunits) transports oxygen for use in cellular metabolism. Additional proteins in the blood plasma and lymph carry nutrients and metabolic waste products throughout the body. The proteins actin and tubulin form cellular structures, while keratin forms the structural support for the dead cells that become fingernails and hair. Antibodies, also called immunoglobins, help recognize and destroy foreign pathogens in the immune system. Actin and myosin allow muscles to contract, while albumin nourishes the early development of an embryo or a seedling. Proteins are a class of macromolecules that perform a diverse range of functions for the cell. They help in metabolism by providing structural support and by acting as enzymes, carriers, or hormones. Protein Types and Functions Type Examples Functions Digestive Amylase, lipase, pepsin, trypsin Help in digestion of food by catabolizing nutrients into Enzymes monomeric units Transport Hemoglobin, albumin Carry substances in the blood or lymph throughout the body Structural Actin, tubulin, keratin Construct different structures, like the cytoskeleton Hormones Insulin, thyroxine Coordinate the activity of different body systems Defense Immunoglobulins Protect the body from foreign pathogens Contractile Actin, myosin Effect muscle contraction AMINO ACID Amino acids are organic compounds that contain amine (–NH2) and carboxyl (–COOH) functional groups, along with a side chain (R group) specific to each amino acid. The key elements of an amino acid are carbon (C), hydrogen (H), oxygen (O), and nitrogen (N), although other elements are found in the side chains of certain amino acids. About 500 naturally occurring amino acids are known, though only 20 appear in the genetic code and can be classified in many ways. In the form of proteins, amino acid residues form the second-largest component (water is the largest) of human muscles and other tissues. Beyond their role as residues in proteins, amino acids participate in a number of processes such as neurotransmitter transport and biosynthesis. The proteins in all living species, from bacteria to humans, are constructed from the same set of 20 amino acids, so called because each contains an amino group attached to a carboxylic acid. The amino acids in proteins are α-amino acids, which means the amino group is attached to the α-carbon of the carboxylic acid. Humans can synthesize only about half of the needed amino acids; the remainder must be obtained from the diet and are known as essential amino acids. However, two additional amino acids have been found in limited quantities in proteins: Selenocysteine was discovered in 1986, while pyrrolysine was discovered in 2002. (The structure of an alpha amino acid in its un-ionized form) https://en.wikipedia.org/wiki/Amino_acid ZWITTERION FORM OF AMINO ACID A zwitterion is a molecule with functional groups, of which at least one has a positive and one has a negative electrical charge. The net charge of the entire molecule is zero. Amino acids are the best-known examples of zwitterions. They contain an amine group (basic) and a carboxylic group (acidic). The -NH2 group is the stronger base, and so it picks up H+ from the -COOH group to leave a zwitterion (i.e. the amine group de-protonates the carboxylic acid). UNCOMMON AMINO ACIDS All amino acids have a carboxyl group and an amino group bonded to the same carbon atom (the α carbon). But, they differ from each other in their R group or the side chain group. The nature of the R-group differentiates each amino acid in terms of their acid/basic nature, solubility, stability, and ultimately the proteins. Proteins in organisms may have some modified residues of the 20 amino acids. 4-hydroxyproline, a derivative of proline, 5-hydroxylysine, derived from lysine and α-carboxyglutamate from glutamic acid are some examples. Selenocysteine is a special case of having selenium rather than the sulfur of cysteine. Some 300 additional amino acids have been found in cells but they are not constituents of proteins. These amino acids are commonly known as uncommon amino acids. Structurally the ‘uncommon’ amino acids can be divided into two categories; those which are close chemical analogues of the ‘common’ amino acids and those which are not. There are three possible ways in which the close analogues may arise: they may be formed by the modification of ‘common’ amino acids; they may arise as a result of modifications to the biosynthetic pathways normaily associated with the synthesis of ‘common’ amino acid; or they may be synthesised by novel routes. Examples of ‘uncommon’ amino acids arising in each of these ways are known. Modifications of either the ‘common’ amino acids themselves or the biosynthetic pathways leading to them may reflect relatively minor changes in the genomes of species already equipped with enzymes required for the synthesis of a full complement of ‘common’ amino acids. When however an ‘uncommon’ amino acid is structurally unlike any ‘common’ amino acid or when it is formed by an unexpected or novel route then its presence probably reflects more extensive changes in the genes controlling amino acid biosynthesis. FORMATION OF PEPTIDE BONDS AND FORMATION OF PROTEINS A peptides is a molecule composed of two or more amino acids. The bond that holds together the two amino acids is a peptide bond, or a covalent chemical bond between two compounds (in this case, two amino acids). It occurs when the carboxylic group of one molecule reacts with the amino group of the other molecule, linking the two molecules and releasing a water molecule. A peptide bond is a chemical bond formed between two molecules when the carboxyl group of one molecule reacts with the amino group of the other molecule, releasing a molecule of water (H2O). Long chain polypeptides can be formed by linking many amino acids to each other via peptide bonds. The amide bond can only be broken by amide hydrolysis, where the bonds are cleaved with the addition of a water molecule. The peptide bonds of proteins are metastable, and will break spontaneously in a slow process. Living organisms have enzymes which are capable of both forming and breaking peptide bonds. PROPERTIES OF PROTEINS Physical Properties of Proteins 1. Colour and Taste Proteins are colourless and usually tasteless. These are homogeneous and crystalline. 2. Shape and Size The proteins range in shape from simple crystalloid spherical structures to long fibrillar structures. 3. Molecular Weight The proteins generally have large molecular weights ranging between 5 × 103 and 1 × 106. It might be noted that the values of molecular weights of many proteins lie close to or multiples of 35,000 and 70,000. 4. Colloidal Nature Because of their giant size, the proteins exhibit many colloidal properties. 5. Denaturation Denaturation refers to the changes in the properties of a protein. In other words, it is the loss of biologic activity. In many instances the process of denaturation is followed by coagulation— a process where denatured protein molecules tend to form large aggregates and to precipitate from solution. 6. Amphoteric Nature Like amino acids, the proteins are amphoteric, i.e., they act as acids and alkalies both. These migrate in an electric field and the direction of migration depends upon the net charge possessed by the molecule. The net charge is influenced by the pH value. Each protein has a fixed value of isoelectric point (pl) at which it will move in an electric field. 7. Ion Binding Capacity The proteins can form salts with both cations and anions based on their net charge. 8. Solubility The solubility of proteins is influenced by pH. Solubility is lowest at isoelectric point and increases with increasing acidity or alkalinity. This is because when the protein molecules exist as either cations or anions, repulsive forces between ions are high, since all the molecules possess excess charges of the same sign. Thus, they will be more soluble than in the isoelectric state. 9. Optical Activity All protein solutions rotate the plane of polarized light to the left, i.e., these are levoratotory. 10. Lesson Proper for Week 5 11. PRIMARY STRUCTURE OF PROTEIN 12. The primary structure of a protein refers to the sequence of amino acids in the polypeptide chain. The primary structure is held together by peptide bonds that are made during the process of protein biosynthesis. For example, the hormone insulin has two polypeptide chains, A and B, shown in diagram below. (The insulin molecule shown here is cow insulin, although its structure is similar to that of human insulin.) Each chain has its own set of amino acids, assembled in a particular order. For instance, the sequence of the A chain starts with glycine at the N-terminus and ends with asparagine at the C-terminus, and is different from the sequence of the B chain. 13. The gene, or sequence of DNA (deoxyribonucleic acid), ultimately determines the unique sequence of amino acids in each peptide chain. A change in nucleotide sequence of the gene’s coding region may lead to a different amino acid being added to the growing polypeptide chain, causing a change in protein structure and therefore function. 14. For instance, a single amino acid change is associated with sickle cell anemia, an inherited disease that affects red blood cells. In sickle cell anemia, one of the polypeptide chains that make up hemoglobin, the protein that carries oxygen in the blood, has a slight sequence change. The glutamic acid that is normally the sixth amino acid of the hemoglobin β chain (one of two types of protein chains that make up hemoglobin) is replaced by a valine. This substitution is shown for a fragment of the β chain in the diagram below. 15. Image of normal and sickle cell mutant hemoglobin chains, showing substitution of valine for glutamic acid in the sickle cell version. 16. SECONDARY STRUCTURE OF PROTEIN 17. The next level of protein structure refers to local folded structures that form within a polypeptide due to interactions between atoms of the backbone. (The backbone just refers to the polypeptide chain apart from the R groups – so all we mean here is that secondary structure does not involve R group atoms.) The most common types of secondary structures are the α helix and the β pleated sheet. Both structures are held in shape by hydrogen bonds, which form between the carbonyl O of one amino acid and the amino H of another. 18. The secondary structure of a protein describes the type of structure that forms when amino acids form hydrogen bonds within a polypeptide or between polypeptide chains. The three most common types of secondary structure are alpha helix, beta-pleated sheet and triple helix. 19. 20. Images showing hydrogen bonding patterns in beta pleated sheets and alpha helices. 21. In an α helix, the carbonyl (C=O) of one amino acid is hydrogen bonded to the amino H (N-H) of an amino acid that is four down the chain. (E.g., the carbonyl of amino acid 1 would form a hydrogen bond to the N-H of amino acid 5.) This pattern of bonding pulls the polypeptide chain into a helical structure that resembles a curled ribbon, with each turn of the helix containing 3.6 amino acids. The R groups of the amino acids stick outward from the α helix, where they are free to interact^33cubed. 22. In a β pleated sheet, two or more segments of a polypeptide chain line up next to each other, forming a sheet-like structure held together by hydrogen bonds. The hydrogen bonds form between carbonyl and amino groups of backbone, while the R groups extend above and below the plane of the sheet^33cubed. The strands of a β pleated sheet may be parallel, pointing in the same direction (meaning that their N- and C-termini match up), or antiparallel, pointing in opposite directions (meaning that the N-terminus of one strand is positioned next to the C-terminus of the other). 23. Many proteins contain both α helices and β pleated sheets, though some contain just one type of secondary structure or do not form either type. 24. Collagen is the most abundant protein in the body, makes up as much as one-third (1/3) of all proteins in vertebrates. It is found in connective tissues, blood vessels, skin, tendons, ligaments, cornea of the eyes and cartilage. The strong structure of collagen is a result of three polypeptides woven together like a braid form of a triple helix. 25. Structure of Collagen 26. TERTIARY STRUCTURE OF PROTEIN 27. The tertiary structure of a protein involves attractions and repulsion between R groups of the amino acids in the polypeptide chains. As interaction occur between different parts of the peptide chain, segments of the chain twist and bend until the protein acquires a specific three-dimensional shape. 28. Interactions in Tertiary Structure 29. 1. HYDROPHOBIC INTERACTIONS- are interactions between two non-polar amino acids that have non- polar R groups. Within a protein, the amino acids with non-polar R groups are pushed away from aqueous environment to form a hydrophobic center at the interior of the protein molecule. 30. 2. HYDROPHILIC INTERACTION- are interactions between the external aqueous environment and the R group of the amino acid that are pulled to the outer surface of globular proteins where they form a hydrogen bonds with water. 31. 3. SALT BRIDGES- are ionic bonds between ionized R groups of basic and acidic amino acid. For example, the ionized R group of arginine, which has a positive charge, can form a salt bridge with the R group in aspartic acid, which has a negative charge. 32. 4. HYDROGEN BONDS- form between H of the polar R group and the O or N of another amino acid. For example, a hydrogen bond can form between the -OH groups of two series or between the -OH of serine and the -NH2 in the R group of glutamine. 33. 5. DISULFIDE BONDS- (-S-S-) are covalent bonds that form between the -SH groups of cysteines in polypeptide chains. 34. 35. Image of a hypothetical polypeptide chain, depicting different types of side chain interactions that can contribute to tertiary structure. These include hydrophobic interactions, ionic bonds, hydrogen bonds, and disulfide bridge formation. 36. QUATERNARY STRUCTURE 37. While many proteins are biologically active as tertiary structures, some proteins require two or more tertiary structures to be biologically active. When a biologically active protein consists of two or more polypeptide chains or subunits, the structural level is referred to as quaternary structure. It refers to how these protein subunits interact with each other and arrange themselves to form a larger aggregate protein complex. The final shape of the protein complex is once again stabilized by various interactions, including hydrogen-bonding, disulfide-bridges and salt bridges. 38. Many proteins are made up of a single polypeptide chain and have only three levels of structure. However, some proteins are made up of multiple polypeptide chains, also known as subunits. When these subunits come together, they give the protein its quaternary structure. 39. An example is DNA polymerase, an enzyme that synthesizes new strands of DNA and is composed of ten subunits. 40. THAI SARS-CoV-2 Replication/Transcription Complex Quaternary Structure. 41. Flowchart Depicting the Four Orders of Protein Structure. 42. 43. Image modified from Open Stax Biology's modification of work by the National Human Genome Research Institute. 44. PROTEIN DENATURATION 45. Due to the nature of the weak interactions controlling the three-dimensional structure, proteins are very sensitive molecules. The term native state is used to describe the protein in its most stable natural conformation in situ. This native state can be disrupted by several external stress factors including temperature, pH, removal of water, presence of hydrophobic surfaces, presence of metal ions and high shear. The loss of secondary, tertiary or quaternary structure due to exposure to a stress factor is called denaturation. 46. Denaturation results in unfolding of the protein into a random or misfolded shape. A denatured protein can have quite a different activity profile than the protein in its native form, usually losing biological function. In addition to becoming denatured, proteins can also form aggregates under certain stress conditions. 47. The loss of secondary and tertiary structures occurs when conditions change, such as temperature increase or making the pH very acidic or basic. 48. Heat can be used to disrupt hydrogen bonds and non-polar hydrophobic interactions. This occurs because heat increases the kinetic energy and causes the molecules to vibrate so rapidly and violently that the bonds are disrupted. 49. 50. GLOBULAR AND FIBROUS PROTEIN 51. Globular Protein have compact, spherical shapes because sections of the polypeptide chain fold over on top of each other due to the various interactions between R groups. It is the globular proteins that carry out the work of the cells: functions as synthesis, transport and metabolism. Myoglobin is a globular protein that stores oxygen in the skeletal muscle. 52. Fibrous Proteins are proteins that consist of long, thin, fiber-liked shapes. They are typically involved in the structure of cells and tissues. 53.