Week one notes.docx
Document Details
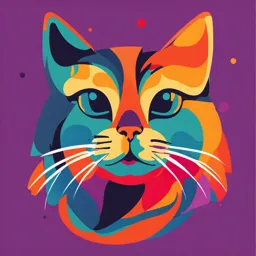
Uploaded by FavorableMetaphor7683
La Trobe University
Full Transcript
Week one notes. Structure and function of the CNS: The central nervous system (CNS) comprises the brain and the spinal cord. It acts as the body\'s control centre, processing sensory information and directing responses. As the CNS coordinates voluntary and involuntary functions, knowing how differ...
Week one notes. Structure and function of the CNS: The central nervous system (CNS) comprises the brain and the spinal cord. It acts as the body\'s control centre, processing sensory information and directing responses. As the CNS coordinates voluntary and involuntary functions, knowing how different structures impact different functions is essential for studying how disordered functioning results in disease. ### ### **Divisions of the human nervous system**  ### ### ### ### **Structure and function of the brain** ### 1. Cerebrum (Pink & Blue Area) - **Controls reasoning** → The **frontal lobe** of the cerebrum is responsible for higher cognitive functions, including reasoning, problem-solving, and decision-making. - **Initiates voluntary activity** → The **motor cortex**, located in the frontal lobe of the cerebrum, controls voluntary muscle movements. - **Integrates sensory and neural function** → The **parietal lobe** of the cerebrum processes sensory input from the body, helping in perception and coordination of movement. ### 2. Brainstem (Lower Pink & Yellow Area) - **Controls heart rate** → The **medulla oblongata**, part of the brainstem, regulates vital autonomic functions like heart rate and breathing. - **Controls blood pressure** → Also managed by the **medulla oblongata**, which adjusts blood vessel diameter to control blood pressure. ### 3. Cerebellum (Purple & Green Area) - **Coordinates motor function** → The **cerebellum** fine-tunes movements, ensuring smooth, coordinated motor activity. - **Responsible for motor control of balance** → The **cerebellum** also helps maintain posture and balance by processing sensory information related to body position ### **Why These Functions Belong There** - The **cerebrum** handles higher-level functions like thought, movement initiation, and sensory integration. - The **brainstem** controls basic life-support functions, like heart rate and breathing. - The **cerebellum** refines movements and ensures balance and coordination. **Structure and function of the spinal cord.** The spinal cord originates in the brain stem and runs through the spine to the lower back, carrying nerve signals between the CNS and the rest of your body. As such, it is surrounded and protected by the vertebrae. Cross section A cross-section of the spinal cord shows this typical \'butterfly\' pattern made from the internal grey matter surrounded by white matter. The dorsal structures (such as the dorsal root and ganglion) are involved in sensory input, while the ventral structures are responsible for motor output. The lateral horn is typically involved in autonomic functions.  Motor neurons Figure 1.2 shows a cross-section of the spinal cord, highlighting the motor neurone pathways, particularly alpha motor neurones, responsible for transmitting signals from the spinal cord to muscles. Motor neurones are located in the grey matter of the ventral horn and conduct signals from the CNS to effector organs. Hence, they are called **efferent neurones**. The dorsal root is associated with sensory input, while the ventral root is associated with motor output. Sensory Neurons Figure 1.3 shows how sensory neurones in the dorsal root ganglia, called **afferent neurones**, carry information from sensory receptors in the periphery to the CNS. Sensory information is transmitted to the spinal cord, processed by interneurons, and translated into motor commands that control muscle movement. This process is essential for coordinating bodily functions and responding to environmental stimuli.  Different levels of the spinal cord interact with different organs. Hence, when the spinal cord is damaged, the outcomes depend on both the amount of damage and the level of the spine impacted. Figure 1.4 demonstrates the levels of signalling for the sympathetic nervous system. Protection and support of the CNS ================================= Our brains are critical to support life and yet are quite delicate, relying on several structures for protection and support. ============================================================================================================================= Meninges ======== The first layer of defence is the skin, followed by the skull, the thick layer of bone that encloses the brain. Beneath the brain are three protective membranes collectively known as the meninges. The most external of these is the dura mater, a thick, strong and fibrous membrane that attaches to the skull and acts as a shock absorber. The middle layer, the arachnoid mater, attaches to the innermost layer and forms the subarachnoid space that is filled with cerebrospinal fluid (CSF). The pia mater is the closest to the brain tissue and provides essential support and nourishment to the neural tissue. Figure 1.5 shows these layers. ============================================================================================================================================================================================================================================================================================================================================================================================================================================================================================================================================================================================================================================================  ### The brain filters blood into CSF, (**Cerebrospinal fluid)**, which circulates throughout the subarachnoid space and ventricles, providing further cushioning and support. The blood-brain barrier, which we will discuss shortly, is a collective term for four sites that prevent unwanted substances and pathogens from entering the brain from the bloodstream and also provide further protection. The cerebrospinal fluid is the fluid found within the brain and spinal cord. The role of the CSF is to do the following: 1. Cushion the brain and spinal cord from mechanical shocks 2. Exchange nutrients and waste between the blood and tissue 3. Provide a constant chemical environment and one suitable for neuronal signalling There are four fluid-filled cavities within the brain, known as the ventricles. The ventricles\' choroid plexus (formed by ependymal cells) filters blood to form the CSF secreted into the ventricles. The CSF then circulates from the lateral, third and fourth ventricles to the cisterns of the brain. Then, it flows into the subarachnoid space, where it envelops the cortical convexities of the brain. From here, the CSF can enter the central canal of the spinal cord or circulate through the subarachnoid space to the arachnoid granulations. The arachnoid granulations are small protrusions of the arachnoid mater that extend into the dural venous sinuses, through which the CSF returns to the venous circulation. Normally, the rate of CSF production is equal to the rate of CSF absorption and is independent of blood or intracerebroventricular pressure. The left side of Figure 1.6 shows this process. In the inset box on the right of Figure 1.6, we see that from the subarachnoid space, there is a retrograde influx of CSF into the parenchyma, where CSF and interstitial fluid interact in the perivascular space alongside blood vessels that course throughout the brain. Astrocytes lining the perivascular space aid in transporting fluid that removes inflammatory waste proteins, which are continually secreted by neurons as byproducts of neuronal activity and would otherwise build up in the brain. Finally, fluid carrying these inflammatory waste products returns to the subarachnoid space and drains into meningeal lymphatic vessels and arachnoid granulations. **Cerebrospinal Fluid (CSF) Circulation** 1. **Production**: Choroid plexuses in the ventricles secrete CSF. 2. **Flow Path**: - Lateral ventricles → interventricular foramina → third ventricle → cerebral aqueduct → fourth ventricle → median and lateral apertures → subarachnoid space. 3. **Absorption**: Arachnoid granulations reabsorb CSF into the venous bloodstream.  The CSF normally consists of glucose, salts, enzymes, and a few white blood cells (typically lymphocytes). Its constituents are tightly regulated as they impact neuronal functioning and the cerebral environment: - The concentrations of both positively and negatively charged ions are highly regulated in the CSF. Ions are key signalling molecules within the CNS, responsible for maintaining neuronal resting membrane potential, facilitating action potentials and neurotransmission. - Large molecules such as immunoglobulins and other proteins are unable to cross the blood-brain barrier; hence, they are typically found in much smaller amounts in the CSF. Indeed, the presence of increased protein in the CSF may indicate various health conditions. - Have you ever heard of MSG? Glutamate is an important amino acid neurotransmitter in the mammalian CNS, so its levels in the CSF are tightly controlled. As a signalling molecule, glutamate fluctuates, but increased plasma levels of glutamate will not impact CSF concentrations. Explore Table 1.1, which follows, to learn more about the concentrations of selected molecules in human CSF. The blood-brain barrier. The blood-brain barrier (BBB) is a collective term for four main interfaces between the blood (from the periphery) and the central nervous system.  These barriers maintain the neural environment by regulating the passage of molecules into and out of the brain. The BBB also protects the brain from microorganisms or toxins circulating in the blood. A **neurovascular unit** is a complex network of cells that work together to ensure the proper functioning of the brain. It plays a critical role in regulating blood flow, maintaining the blood-brain barrier, providing nutrients and removing waste products from the brain tissue. Vascular endothelial cells, astrocytes and pericytes form the neurovascular unit of the BBB: - Endothelial cells that form the walls of the capillaries are joined by **tight junctions**, which prevent large molecules and pathogens from passing through. - Endothelial cells are surrounded by astrocyte foot processes that wrap around the blood vessel, enhancing the barrier and releasing factors that maintain the integrity of the **tight junctions**. - Astrocytes exchange nutrients and waste products between blood vessels and neurons. They take nutrients from the blood and deliver them to neurones while removing waste products from the brain tissue. - Pericytes surround blood vessels and help regulate blood flow by contracting or relaxing. - Microglia are immune cells that provide further support by continuously scanning the brain environment for damage or pathogens. Figure 1.7 illustrates the neurovascular unit and how the various cell types work together to regulate blood flow and maintain the brain\'s environment.  Crossing the blood-brain barrier The BBB selectively controls what enters the brain. Lipid-soluble molecules can pass through the lipid membranes of the cells of the BBB, whereas water-soluble molecules cannot. Large molecules are also unable to pass through the tight junctions. Hence, the following specific transport systems exist to allow larger or water-soluble molecules to cross the BBB.   Cells of the CNS ================ There are two main types of cells in the CNS. Neurones are the functional units that transmit information within the nervous system through electrical and chemical signalling. The different glial cells have roles in supporting and protecting the CNS. As the main functional unit, neurones take up 50% of the space in the CNS. However, they are outnumbered 3:1 by glial cells, which are critical to maintaining a healthy environment. ### **Structure of neurones** The parts of the neurone play different roles, receiving and transmitting information via dendrites and axons: - **Cell body:** Responsible for metabolism, genetic information (in the nucleus) and protein synthesis. - **Dendrites:** Receive incoming signals. - **Axons: **Convey electrical signals (0.1--3 mm). - **Myelin: **Increases the speed of action potential, effectively insulating from loss of electrical signal. - **Node of Ranvier:** These gaps in the myelin boost the amplitude of the action potential to prevent dying out.  ### **Glial cells** Glial cells are non-neuronal cells in the central nervous system (CNS) and peripheral nervous system (PNS) that provide structural and functional support to neurons. While neurons are responsible for transmitting electrical signals, glial cells assist with various support functions to ensure the neurons can operate efficiently, playing crucial roles in many body processes.  Signalling within the CNS ========================= The central nervous system (CNS) serves as the communication hub within the body. Its primary function is transmitting signals between different brain regions, the spinal cord, and other body parts. We will now explore the properties of neurones that make them able to send electrical signals, the widespread use of chemical signalling within the CNS, and how we can achieve diversity in signalling messages. ### ### **Neurones are excitable cells** When we say that neurones are excitable cells, we refer to their ability to generate and transmit electrical signals. Neurones at rest have a resting membrane potential, which refers to the uneven distribution of charged ions on either side of the membrane. Neurones can then respond to various stimuli, with voltage changes that increase or decrease the likelihood of an electrical signal being sent. This video \[2.00 min.\] will discuss resting membrane potential, explaining how the charges are organised on either side of the neuronal plasma membrane. This will assist you in visualising what is happening at rest so we can build on this to show how our changes in these charges are used for cell signalling.  The resting membrane of the neuron is -70MV also known as the resting membrane potential  A close-up of a white background Description automatically generated  A screenshot of a cell structure Description automatically generated  #### **Action potentials** An action potential is a brief, rapid change in the electrical potential of a neurone's cell membrane. The voltage change is an important signalling mechanism that can transmit electrical impulses at considerable distances along the neuronal membrane. Action potentials are long-range signals that are actively propagated (their amplitude doesn't change) in one direction, away from the cell body and toward the axon terminals. They are also all or nothing. An action potential is triggered, or it isn't. The likelihood of generating an action potential depends on ion movement. If the incoming signal allows more negative ions into the cell, then the membrane becomes hyperpolarised (more negative) and less likely to trigger an action potential. If the signal results in the influx of positive ions, then the membrane is depolarised (more positive). This increases the likelihood of triggering an action potential.      ### **Synaptic transmission** Once an action potential reaches its terminus, the signal needs to be relayed to adjacent cells. This process is referred to as synaptic transmission. This is signal transmission that occurs at a synapse, which is the point at which one neurone communicates with another. The average neurone is estimated to form approximately 1,000 synaptic connections and receives up to 10,000 (100,000 for cerebellar Purkinje cells). As the estimated number of human brain cells ranges from 80 billion to 120 billion, that's **a lot** of connections! Synapses can be electrical or chemical. Electrical synapses are simple depolarising (excitatory) signals -- they do not lend themselves to inhibitory signals that make lasting changes. Signal transduction is instantaneous and occurs at specialised regions called gap junctions. Chemical synapses are more common in humans, where chemicals are released that exert an effect on adjacent cells. Diversity in signalling can be achieved depending on what chemical (neurotransmitter) is present and which receptors are in the adjacent cell's membranes. Figure 1.8 shows synaptic transmission. Most images you see will be a typical axodendritic synapse (axon to dendrite); however, it is important to remember that there are many other possible connections between neurones at which synapses can be found and different ways that neurones communicate with each other, as shown in Figure 1.9.  ### **Diversity in signalling** The diversity of signalling in the CNS arises from the intricate network of neurones, each with unique properties. As we study different disease processes, I will draw your attention to specific neuronal populations, networks and neurotransmitters. Hence, it will be helpful to understand how diversity in signalling is achieved within the CNS.   **Neurotransmitters and receptors** At least 100 different types of neurotransmitters have been identified, each acting as different receptors and releasing specific neurotransmitters that bind to receptors on target cells. There is considerable diversity in receptors, as many neurotransmitters can act at both ion channel-coupled receptors and G protein-coupled receptors. Binding at an ion channel-coupled receptor allows the opening of an ion channel. These are typically selective for different cations or anions. Hence, activation can result in depolarisation or hyperpolarisation of the neuronal membrane. The composition of receptor subunits in different receptor subtypes influences to which ions the channel is permeable. Binding to G protein-coupled receptors activates one of a number of possible G proteins, which then activate specific enzyme systems that result in the activation of different intracellular signalling pathways. Neuropeptides can also act as neurotransmitters but tend to work more slowly and have longer-lasting effects. Metabolic needs of the CNS ========================== The human brain has a high metabolic rate and oxygen requirement. It is over three times larger than the brains of other animals our size, contains over one hundred billion neurons, and has more neuronal connections than we can count. It is estimated that we each have over 16,800 GHz of processing power, 1 million GB of memory and potential ports for 21 senses. Hence, although the brain represents approximately 2% of our body weight, it receives approximately 15% of cardiac output. Neurones have an **absolute** reliance on ATP produced in the mitochondria (remember oxidative phosphorylation through the electron transport chain generated 30--36 molecules of ATP per molecule of glucose compared with two from glycolysis) and, as a result, a high oxygen requirement. The brain consumes approximately 20% of O~2~ and requires 100 g glucose (\~50% RDI of carbohydrates) to maintain its function. To deliver glucose and oxygen, the brain receives a blood flow of 750--1000 ml/min. The blood flow in the grey matter is four times that of the white matter. ### **Cerebral autoregulation** Maintaining constant cerebral blood flow is thus critical for optimum function. Hence, cerebral blood flow is autoregulated. This autoregulation means cerebral blood vessels can alter their diameter in response to changes in physiological conditions such as blood pressure and blood gas concentrations.    