WEEK 4.docx
Document Details
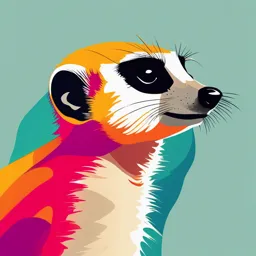
Uploaded by HandyConflict
Affiliated Hospital of Nanjing University of Chinese Medicine
Full Transcript
LGs: What is the pathogenesis of AS? Atherosclerosis is a complex cardiovascular disease characterized by the buildup of plaques within the arterial walls, leading to reduced blood flow and potentially severe health consequences such as heart attacks and strokes. Here’s a detailed explanation of the...
LGs: What is the pathogenesis of AS? Atherosclerosis is a complex cardiovascular disease characterized by the buildup of plaques within the arterial walls, leading to reduced blood flow and potentially severe health consequences such as heart attacks and strokes. Here’s a detailed explanation of the pathogenesis of atherosclerosis: Initial Stages 1. Endothelial Dysfunction: Damage or dysfunction of endothelial cells (ECs) lining the arterial walls is typically the first step in atherosclerosis. Factors contributing to endothelial injury include hypertension, high levels of low-density lipoprotein (LDL), smoking, diabetes, and inflammation. 2. Lipid Accumulation: Following endothelial injury, LDL cholesterol circulating in the blood accumulates in the walls of the arteries. Once inside, LDL undergoes oxidative modification to form oxidized LDL (oxLDL), which is highly inflammatory. 3. Monocyte Recruitment and Foam Cell Formation: OxLDL triggers an inflammatory response, leading to the recruitment of monocytes from the blood into the intima (inner layer) of the artery. These monocytes differentiate into macrophages, which engulf oxLDL and become lipid-laden foam cells. Foam cells accumulate to form fatty streaks, the earliest visible signs of atherosclerosis. Progression of Atherosclerosis 1. Fibrous Cap Formation: As the fatty streaks grow, smooth muscle cells (SMCs) migrate from the media (middle layer) to the intima, where they proliferate and produce extracellular matrix (ECM) components, including collagen and elastin. This results in the formation of a fibrous cap over the fatty streak, creating a more complex atheromatous plaque. 2. Necrotic Core Development: The core of the plaque contains dead cells (apoptotic and necrotic), free cholesterol, and cellular debris, forming the necrotic core. Ineffective clearance of these dead cells, a process known as defective efferocytosis, exacerbates inflammation and plaque instability. Plaque Instability and Complications 1. Thinning of the Fibrous Cap: Chronic inflammation within the plaque can lead to thinning of the fibrous cap, primarily due to the degradation of collagen by enzymes such as matrix metalloproteinases (MMPs) secreted by activated macrophages. 2. Plaque Rupture and Thrombosis: As the fibrous cap weakens, the plaque is prone to rupture, exposing the thrombogenic contents of the necrotic core to the bloodstream. This exposure can lead to the formation of a thrombus (blood clot), which can obstruct blood flow and lead to acute cardiovascular events like myocardial infarction or stroke. 3. Calcification: Advanced plaques may also undergo calcification, where calcium phosphate crystals deposit within the plaque, further reducing arterial elasticity and contributing to the stiffening of the vessel walls. Calcification is considered a hallmark of advanced, stable plaques but can also contribute to their brittleness. Management and Prevention Lifestyle Modifications: Diet, exercise, and smoking cessation are crucial in managing and preventing atherosclerosis. Medications: Statins (to lower cholesterol), antihypertensive drugs, and antiplatelet agents are commonly used. Surgical Interventions: In advanced cases, procedures such as angioplasty or arterial bypass grafting may be necessary. Cell types beyond macrophages, each contributing uniquely to the development, progression, and complications of the disease. Endothelial Cells (ECs) Location & Function: Line the internal surface of blood vessels, regulating vascular tone, blood flow, and barrier function. Contribution to Atherosclerosis: ECs respond to mechanical stress and metabolic changes by increasing vascular permeability and expressing adhesion molecules like VCAM-1 and ICAM-1. This facilitates leukocyte adhesion and promotes a proinflammatory state, contributing to early plaque development. Vascular Smooth Muscle Cells (VSMCs) Location & Function: Found in the tunica media of arteries, regulating vessel tone and structural integrity. Contribution to Atherosclerosis: VSMCs undergo phenotypic switching in response to atherosclerotic stimuli, transitioning from a contractile to a synthetic state. They migrate to the intima, proliferate, and produce extracellular matrix, contributing to plaque formation and stabilization. Dendritic Cells (DCs) Location & Function: Located within the intimal and adventitial layers of arteries, acting as antigen-presenting cells that bridge innate and adaptive immunity. Contribution to Atherosclerosis: DCs have dual roles, with mature DCs promoting atherosclerosis by activating T cells, and immature DCs potentially protecting against it by inducing regulatory T cells (Tregs). T Cells Location & Function: Present in various regions of the artery including the adventitia and intima, involved in adaptive immune responses. Contribution to Atherosclerosis: - Initiation: Activated by oxLDL and other antigens, leading to cytokine secretion and further recruitment of immune cells. - Plaque Progression: Cytokines from T cells stimulate SMC proliferation and collagen synthesis, worsening plaque growth. - Plaque Instability: Cytotoxic T cells can induce apoptosis in plaque cells, contributing to necrotic core formation and cap weakening. B Cells Location & Function: Found in the adventitia and within plaques, capable of producing antibodies, presenting antigens, and regulating T cells. Contribution to Atherosclerosis: - Pro-inflammatory Role: B cells can exacerbate atherosclerosis by promoting inflammation and adaptive immune responses within plaques. - Protective Role: B cells also produce antibodies that neutralize modified LDL and facilitate the clearance of apoptotic cells, potentially stabilizing plaques. Platelets Location & Function: Circulate in the bloodstream and accumulate at sites of endothelial injury. Contribution to Atherosclerosis: Platelets adhere to damaged endothelium, secreting growth factors and cytokines that promote leukocyte recruitment, VSMC migration, and proliferation. They play a crucial role in thrombus formation following plaque rupture. What are the different macrophage subsets present in the atherosclerotic plaque? What is their function? (most recent knowledge: transcriptomics) Macrophage Subsets in Atherosclerosis: 1. Resident Macrophages: - Origin: Derived from monocyte progenitors present in the artery since birth. - Location: Primarily found within the tunica adventitia or near the tunica media in the plaque. - Function: These macrophages are involved in homeostatic functions and contribute to early atherogenesis by potentially becoming foam cells. They also play a role in the recruitment of monocytes and influence the trans-differentiation of vascular smooth muscle cells (VSMCs) to osteogenic-like cells, contributing to vascular calcification. 2. Inflammatory Macrophages: - Origin: Derived from circulating monocytes that infiltrate the plaque. - Location: Located near the plaque’s lumen (shoulder regions) and within the adventitia. - Function: These macrophages express pro-inflammatory cytokines and chemokines, contributing to the progression of inflammation in the plaque. They are involved in the degradation of the extracellular matrix, thinning the fibrous cap and increasing susceptibility to rupture. 3. Foamy Macrophages: - Origin: Arise from both resident macrophages and circulating monocytes. - Location: Distributed throughout the plaque but are particularly concentrated where lipid accumulation is significant. - Function: Characterized by the ingestion of oxidized LDL, leading to foam cell formation. They contribute to the growth and instability of the plaque due to their involvement in lipid accumulation and inflammatory processes. 4. Smooth Muscle Cell-Derived Macrophages: - Origin: Derived from VSMCs that have undergone phenotypic switching in response to atherosclerotic stimuli. - Location: Found throughout the plaque, contributing to its structural complexity. - Function: These cells contribute to plaque formation and remodeling by synthesizing and remodeling the extracellular matrix. They also play roles in plaque stability and progression through their involvement in calcification processes. Transcriptomic Insights from Single-Cell RNA Sequencing: - Resident-like macrophages express genes associated with tissue maintenance and homeostasis. - Inflammatory macrophages show upregulation of genes linked to pro-inflammatory responses and matrix remodeling. - Foamy macrophages are characterized by the expression of genes involved in lipid processing and storage. These findings underscore the complexity of macrophage roles in atherosclerosis, challenging the traditional M1/M2 polarization paradigm and highlighting the dynamic nature of macrophage phenotypes in response to microenvironmental cues within atherosclerotic plaques. Clinical Implications: Understanding the diverse roles of macrophage subsets in atherosclerosis can inform therapeutic strategies aimed at modulating macrophage functions to stabilize plaques and prevent acute cardiovascular events. Targeting specific macrophage pathways, such as those involved in inflammation, lipid metabolism, or calcification, may offer new avenues for the treatment and management of atherosclerosis. Additionally, the knowledge of macrophage diversity and plasticity underscores the importance of personalized and targeted approaches in cardiovascular therapy. What are existing models to study atherosclerosis? Mouse Models Mouse models are crucial in atherosclerosis research due to their genetic tractability, ease of handling, and the relevance of their immune and metabolic systems to human pathophysiology. Key mouse models include: 1. Apolipoprotein E-deficient (ApoE-/-) Mice: - Lack the gene for ApoE, critical in lipid metabolism. - Develop spontaneous hypercholesterolemia and atherosclerotic lesions when fed a normal diet. - Used to study lipid metabolism dysfunctions and macrophage behavior in atherosclerosis. 2. Low-density lipoprotein receptor-deficient (LDLR-/-) Mice: - Lack LDL receptors, important for clearing LDL from the bloodstream. - Develop atherosclerosis when fed a high-fat diet, mimicking human-like hypercholesterolemia. - Useful for studying the impact of elevated LDL levels and the effects of diet on atherosclerosis. 3. Double Knockout Mice (ApoE-/- LDLR-/-): - Deficient in both ApoE and LDL receptors. - Show severe hyperlipidemia and rapid atherosclerosis progression even on a regular diet. - Employed to test the efficacy of anti-atherosclerotic therapies under severe pathological conditions. 4. ApoE-Leiden Mice: - Carry a mutant form of ApoE, leading to moderate hypercholesterolemia. - Develop atherosclerosis when fed a Western-type diet. - Useful for studying human-like lipoprotein profiles and the response to dietary interventions. Other Animal Models While mice are invaluable, other animal models offer complementary insights due to differences in lipid metabolism, cardiovascular anatomy, and plaque characteristics: 1. Rabbits: - Naturally more sensitive to dietary cholesterol. - Develop atherosclerotic lesions primarily in the aorta and are excellent for studying lipid-driven atherosclerosis. 2. Pigs: - Similar cardiovascular system size to humans, allowing for interventional studies. - Develop complex atherosclerotic lesions that more closely mimic human disease, including responses to mechanical stress in arteries. 3. Non-human Primates: - Genetically and physiologically closer to humans. - Develop atherosclerosis naturally when subjected to diets high in fat and cholesterol. - Used to study the long-term progression of atherosclerosis and the effectiveness of pharmacological interventions. In Vitro and In Silico Models To complement in vivo studies, in vitro and in silico models offer controlled environments to dissect cellular mechanisms and predict disease progression and drug efficacy: 1. In Vitro Models: - Single-cell and co-culture systems: Allow detailed study of cell-cell interactions and the effects of drugs on specific cell types involved in atherosclerosis. - Advanced 2D models and microfluidic chips: Mimic blood flow and shear stress, providing insights into the effects of mechanical forces on atherosclerotic development. 2. Ex Vivo Models: - Cultures of human atherosclerotic plaques maintain the complex architecture and cellular composition, enabling studies on cell functionality and intercellular interactions within the plaque environment. 3. In Silico Models: - Utilize computational simulations to model disease progression and drug responses based on genetic, cellular, and biochemical data. - Can predict patient-specific responses to treatments, facilitating personalized medicine approaches. What are current/novel treatments for AS? Current Treatments for Atherosclerosis 1. Statins: - Mechanism: Inhibit the enzyme HMG-CoA reductase in the cholesterol biosynthesis pathway, reducing low-density lipoprotein cholesterol (LDL-C) levels and providing anti-inflammatory and antioxidant effects. - Clinical Benefit: Statins are the cornerstone of atherosclerosis treatment, significantly reducing the risk of cardiovascular events. 2. PCSK9 Inhibitors: - Examples: Evolocumab and Alirocumab -Mechanism: Monoclonal antibodies that inhibit proprotein convertase subtilisin/kexin type 9 (PCSK9), enhancing the recycling of LDL receptors and thereby lowering LDL-C levels. - Clinical Benefit: Used primarily in patients with genetic lipid disorders or those who do not achieve adequate LDL-C reduction with statins alone. 3. Ezetimibe: - Mechanism: Inhibits the Niemann-Pick C1-like 1 (NPC1L1) protein, reducing the absorption of cholesterol from the small intestine. - Clinical Benefit: Often used in combination with statins to further lower LDL-C levels. 4. Bempedoic Acid: - Mechanism: Inhibits ATP citrate lyase, an enzyme upstream of HMG-CoA reductase in the cholesterol synthesis pathway. - Clinical Benefit: Provides an additional LDL-C lowering effect, particularly beneficial for patients who cannot tolerate statins. 5. Bile Acid Sequestrants: - Examples: Cholestyramine, Colestipol, Colesevelam - Mechanism: Bind bile acids in the intestine, preventing their reabsorption and promoting cholesterol catabolism to replace bile acids. - Clinical Benefit: Used as an adjunct therapy for reducing LDL-C. Novel Treatments for Atherosclerosis 1. Olpasiran: - Mechanism: A small interfering RNA (siRNA) therapy targeting LPA messenger RNA to reduce the synthesis of apolipoprotein(a), thereby lowering lipoprotein(a) levels, a risk factor for atherosclerosis. - Clinical Benefit: Promising new therapy for patients with elevated lipoprotein(a) levels, an independent risk factor for atherosclerotic cardiovascular disease. 2. Olezarsen: - Mechanism: An antisense oligonucleotide targeting hepatic APOC3 mRNA, reducing the production of apolipoprotein C-III, which inhibits lipoprotein lipase. - Clinical Benefit: Lowers triglyceride levels and may have beneficial effects on cardiovascular outcomes. 3. Evinacumab: - Mechanism: A monoclonal antibody that inhibits angiopoietin-like protein 3 (ANGPTL3), enhancing the activity of lipoprotein lipase and reducing levels of triglycerides and LDL-C. - Clinical Benefit: Particularly beneficial for patients with familial hypercholesterolemia and those who do not adequately respond to other lipid-lowering therapies. Immunomodulatory Treatments 1. Ziltivekimab: - Mechanism: Targets and inhibits interleukin-6 (IL-6) signaling, reducing inflammation associated with atherosclerosis. - Clinical Benefit: May reduce biomarkers of inflammation and potentially lower the risk of cardiovascular events, currently under investigation in clinical trials. 2. Colchicine: - Mechanism: Anti-inflammatory medication that affects multiple cellular mechanisms including leukocyte recruitment and inflammasome activation. - Clinical Benefit: Shown to reduce the risk of cardiovascular events in patients with coronary artery disease. 3. Losmapimod: - Mechanism*: Inhibits p38 MAP kinase, an enzyme involved in inflammatory responses linked to atherosclerosis. - Clinical Benefit: Although initial cardiovascular outcomes trials were not successful, ongoing research continues to explore the potential benefits in specific patient subsets.