Week 2_S2 2024 Lecture Slides PDF
Document Details
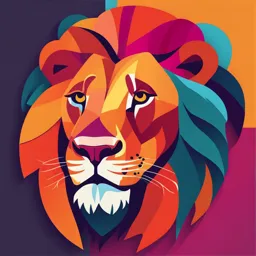
Uploaded by AngelicGroup2698
The University of Sydney
2024
Dr Sandhya Clement
Tags
Related
- Week 3_S2 2024 Biomedical Instrumentation Lecture Slides PDF
- Ch5_BME420_Biopotential Electrodes PDF
- Chapter 6: Biopotential Amplifiers PDF
- BMET3802/9802 Biomedical Instrumentation Week 02 Lecture Slides 2024 PDF
- Biomedical Instrumentation (BME420) Chapter 5 Bio-potential Electrodes PDF
- Mechatronic for Health Sciences Lecture No. 3 PDF
Summary
This is a university lecture on biomedical instrumentation, focusing on biopotential, including the details of cell membranes, resting potentials, action potentials, and volume conduction. It covers the basics and includes information on measurements and examples.
Full Transcript
BMET3802/9802 Biomedical Instrumentation Week 02: Biopotential Dr Sandhya Clement Lecturer School of Biomedical Engine...
BMET3802/9802 Biomedical Instrumentation Week 02: Biopotential Dr Sandhya Clement Lecturer School of Biomedical Engineering The University of Sydney I would like to acknowledge the Traditional Owners of Australia and recognise their continuing connection to land, water and culture. I am currently on the land of the Cadigal people of the Eora Nation, and I pay my respects to their Elders, past, present and emerging. I further acknowledge the Traditional Owners of the country on which you are on and pay respects to their Elders, past, present and future. The University of Sydney Pre-Semester Survey feedback Was there anything unclear with the course structure/ unit outline/ CANVAS site etc Not many suggestions, most of them mentioned that everything is clear. How can I best support you during this semester? Be kind and reply to emails. Easy lecture slides, examples, timely upload of lecture recording Answering and asking questions Help to improve circuit knowledge Not much idea about biology/physiology Ed Discussion forum Help in the lab Clear expectation. The University of Sydney This lecture – Biopotential in detail – Cell membrane – Resting potential – Action potential – Volume conduction field – Measurement of Biopotential activity – Electroneurogram (ENG) – Signal conditioning for Biopotential recordings – Filter introduction (for lab) The University of Sydney Page 4 Biopotentials The University of Sydney Page 5 Introduction – Biopotentials are formed by electrochemical activity of a class of cells known as excitable cells – Excitable cells are the fundamental components of nervous, muscular and glandular tissue – Electrically they exhibit a ‘resting potential’ (RP) – When stimulated (by an electric charge, sense or chemical) they can produce an ‘action potential’ (AP) – Neurons, muscle cells (skeletal, cardiac, and smooth), and some endocrine cells (e.g., insulin-releasing pancreatic β cells) are excitable cells. – A peculiar subclass of excitable cells does not require stimulation to produce APs – cardiac pacemaker – All the bioelectric potentials (the potentials that we can measure and use clinically like EEG, ECG,... ) are produced as a result of electrochemical activity of the excitable Cells that are components of Nervous and Muscular tissue The University of Sydney Page 6 Cell membrane – Membrane separates the interior of the cell from the outside environment – Composed of two layers of phospholipids (5-15 nm) – The head is hydrophilic (attracted to water). – The tails are hydrophobic (repelled by water). – So, in water they form clumps – On the surface they form a layer. The University of Sydney Page 7 Membrane potential – There are different ions that exist on both sides of the cell membrane. – Most of these ions can travel through the permitted channels. – These channels are electrically or chemically opened. – sodium, potassium, and chloride molecules flow through the membrane and generate the bioelectric phenomena. – Membrane potential is the difference in electrical potential between the interior and exterior of the cell membrane. – There are two forms for membrane potential 1. Resting Potential (RP) 2. Action potential (AP) https://en.wikipedia.org/wiki/Membrane_potential The University of Sydney Page 8 Resting potentials (RP) The University of Sydney Page 9 Membrane Resting potential (RP) – Individual excitable cells (including other cells) maintain a steady electric potential difference between their internal and external internal environment when they are at rest. – The resting potential of the cell is -40 to -90 mV relative to the outside of the cell. – There are two basic mechanisms that maintain the membrane resting potential. 1. Diffusion (does not need the energy) 2. Active transport (Sodium and Potassium pumps; needs energy). – Energy for the pump is provided by a common source of cellular energy, adenosine triphosphate (ATP) produced by mitochondria in the cell. The University of Sydney Page 10 Resting potential due to Diffusion – Cell membranes at rest are freely permeable to K+ than Na+ – Also, the concentration of K+ is higher inside the cell than outside. – This concentration difference creates a diffusion and K ions start moving inside to the outside of the membrane. – This creates the inside of the cell more negative than the outside. – As a result, a transmembrane potential, called resting potential is developed. – This potential also called diffusion potential, or the equilibrium potential is calculated using the Nernst equation as 𝑅𝑇 𝐾𝑜 𝐾𝑜 𝐸𝑘 = 𝑙𝑛 = 0.065 log 𝑛𝐹 𝐾𝑖 𝐾𝑖 Ko and Ki are the concentration of the K ions (moles/litre) outside and inside of a membrane, R is the universal gas constant, T the absolute temperature of the body, n is the valence of the ions, and F is the Faraday’s constant. The University of Sydney Page 11 More generalized Resting potential equation – Goldman-Hodgkin-Katz (GHK) equation Where Vm is the membrane resting potential; PM is the Permeability Coefficient of the membrane to the particular ionic species M; [M]O is the concentration of the ionic species M outside the cell and [M]I is the concentration of the ionic species M inside the cell. R is the gas constant (8.31 J/(mol.K)); F is Faraday’s constant (96500 C/mole), and T is the absolute temperature in kelvin. The University of Sydney Page 12 Active transport (Sodium and Potassium pumps; needs energy) – There is an active transport mechanism located within the cell and this is called the sodium-potassium pump. – The energy for the pump is provided by a common source of cellular energy, adenosine triphosphate (ATP) produced by mitochondria in the cell. – This pump actively transports Na+ out of the cell and From Wikipedia K+ into the cell in the ratio of 3: 2. – This makes the inside of the cell more negative than the outside or we can say that it allows to maintain the ion gradient. The University of Sydney Page 13 Example Resting Potential Values Cell type Resting Potential Skeletel muscle cell -95 mV Neurons -80 to -90 mV Smooth muscle cells -60 mV Aorta smooth muscle cells -45 mV Hair cells (Cochlea) -15 to -40 mV The University of Sydney Page 14 The University of Sydney Page 15 Membrane potential: electrical equivalent (Hodgkin-Huxley) model – In this diagram, VNa, VK, and VL represent the absolute values of the respective potential of each ion channel. – The signs indicate the direction when the extracellular medium has a normal composition (high Na and Cl, and low K, concentrations). The University of Sydney Page 16 HHmodel – https://sites.google.com/view/andrewkrause/t ools-code/hodgkin-huxley-simulation-with- javascript The University of Sydney Page 17 A glimpse on Nerve cell (Neuron) – The nerve cell may be divided on the basis of its structure and function into three main parts: – cell body, or soma; – Dendrites – a single long nerve fiber, the axon. – Myelinated fibers are similar to coaxial cables The University of Sydney Page 18 Action potentials (AP) The University of Sydney Page 19 The Action Potential (AP) – Biopotentials are formed by the electrochemical activity of a class of cells known as excitable cells. – All cells exhibit resting potential. The excitable cells When stimulated (by an electric charge, sense, or chemical) can produce an ‘action potential’ (AP) – Neurons, muscle cells (skeletal, cardiac, and smooth), and some endocrine cells (e.g., insulin-releasing pancreatic β cells) are excitable cells. – The action potential will be explained by considering nerve cells. The University of Sydney Page 20 Action potential (AP) – The AP is a transient disturbance of the membrane resting potential when an adequate stimulus is given to the excitable cell. The University of Sydney Page 21 Depolarization INSIDE The University of Sydney Page 22 Repolarisation The University of Sydney Page 23 AP and refractory periods At the beginning of the action potential, the Na+ channels open and Na+ moves into the axon, causing depolarization. Repolarization occurs when the K+ channels open and K+ moves out of the axon. The cell is unable to respond to another stimulus for the Refractory Period (RP) where it may be hyperpolarized https://www.youtube.com/watch?v=HnKMB11ih2o&t=13s The University of Sydney Page 24 Refractory Period – The cell membranes can not respond to any stimulus during the initial portion of the AP. – This unresponding period is called the absolute refractory period (ARP). – The relative refractory period (RRP) follows the absolute refractory period. – In the RRP, the generation of a new AP is possible, but only upon a super threshold stimulus. In the graph, g represents the conductance The University of Sydney Page 25 Action potential (AP) – As an action potential travels down the axon, there is a change in polarity across the membrane. – Ion channels create a change in polarity between the outside of the cell and the https://www.youtube.com/watch?v=8yC--NvBn_M inside. – The impulse travels down the axon in one direction only, to the axon terminal where it signals to other neurons. – Due to the local generation of the signal, there is no attenuation in AP. – The speed of propagation of AP is different in unmyelinated (0.5m/sec) compared to the myelinated fiber (100 m/sec) The University of Sydney Page 26 AP Measurement from a nerve fiber – A stimulator will provide a brief pulse current to the axon. – A micropipette is used to record the biopotential. – The diameter of the pipette tip must be small compared to the size of the cell. – There will be movement artifact recorded at the beginning and then it shows the resting potential. – Once the stimulus is applied, its electric field effect instantaneously as artifacts, but after a time period L (latent period), you can see the conduction of action potential with a constant velocity. The University of Sydney Page 27 Volume Conduction Field The University of Sydney Page 28 Volume conduction field – So far, the measurement of AP in a single nerve cell is considered. – Clinically, often we considered measuring the electrical activity of various organs such as the heart (ECG), brain (EEG), etc. – So, most of the measurements happen at the surface of the body. – How does microscopic cellular electrical activity conduct to the body’s surface for external measurement? The University of Sydney Page 29 Volume conduction field – The transfer of electrical potentials to a site a distance away from the generator is called volume conduction. – The system now comprises two main parts – The bioelectric source, is a constant current one. – It’s bathing medium as an electric load. – The current source produces an electric field in the medium, which causes a potential difference between any pair of electrodes within the bathing medium. – The associated potential waveform measured at the surface in compared to the previously discussed AP is – Triphasic in nature – Has greater spatial (time) extend – Much smaller in peak-peak amplitude. The University of Sydney Page 30 Example measurement of extracellular field potential – APs are studied in isolated cells immersed in a large bath of saline solution, this is a first (and trivial) example of volume conductor (active frog sciatic nerve) – The complexity of the problem increases when N interactive excitable cells (i.e. N>100 like in an entire trunk of the nerve) are studied in a saline tank – The complexity of the problem increases, even more, when the same sample is studied “in vivo”. – This understanding of volume-conductor fields later allowed the recording of biosignals associated with various organs from the body surface. The University of Sydney Page 31 Biopotential Measurement: ENG The University of Sydney Page 32 Electroneurogram (ENG) – Recording of the activity of the neurons in the central nervous system (CNS: brain, spinal cord) or peripheral nervous system (PNS: nerves, ganglions). – Please note EEG is a type of ENG with a special focus on brain activity. – The ENG helps to study the functioning of the peripheral nerves (both sensory and motor nerves) and has high clinical importance, especially in the period of regeneration after some injury. – The main parameters that are measured and assessed are – the conducting velocity of the peripheral nerve – the latency (time duration) of the response from muscle The University of Sydney Page 33 A glimpse of nerve system communication https://www.christopherreeve.org/blog/life-after-paralysis/sensory-nerves The University of Sydney Page 34 ENG in general: Neural conduction velocity measurement – Nerves are simulated simultaneously at two different sites (S0 and S1) at a known distance of D apart. – The signals are recorded for both sites. – The conduction velocity is calculated as 𝐷 𝑣𝑒𝑙𝑜𝑐𝑖𝑡𝑦 = 𝑡1 − 𝑡2 – This conduction velocity is having a clinical value and is useful in the assessment of peripheral nerve functions. – For eg: the conduction velocity of a regenerating nerve fiber is slowed following nerve injury. The University of Sydney Page 35 Measurement of Sensory Nerve conduction velocity – The study of evoked potential from a sensory nerve has a greater role in diagnosing peripheral nerve disorders. – Extracellular field responses from sensory nerves can be easily measured from the median or ulnar nerves of the arm by using ring stimulating electrodes applied to the fingers. – The stimulating signal must be a short but strong pulse, because long period pulses cause muscle contraction, limb movements, and therefore artifacts. 𝐷 (100V, 100-300 micro sec) 𝑣𝑒𝑙𝑜𝑐𝑖𝑡𝑦 = 𝑡1 − 𝑡2 The University of Sydney Page 36 Measurement of Sensory Nerve conduction – These types of stimuli can excite only the large, rapidly conducting nerve fibers such as sensory nerves but not others (like small pain fibers or surrounding muscle fibers). – The patient ground electrode is placed in between the stimulating electrode and recording electrodes. – This will help to reduce the effect of simulating the signal on the recorded ENG. – The recording instrumentation must have a very good sensor and bio- potential amplifier (high gain, high CMRR, high input impedance) to record and display 𝐷 the signal. 𝑣𝑒𝑙𝑜𝑐𝑖𝑡𝑦 = 𝑡1 − 𝑡2 The University of Sydney Page 37 Measurement of Motor Nerve conduction velocity – The patient ground electrode is placed in between the stimulating electrode and recording electrodes. – In this example only, one set of electrodes for recording. – The time difference between stimuli and the recorded signal is used for velocity calculation.. The University of Sydney Page 38 Measurement of Reflexly evoked potential – With a smaller intensity stimulus, only the large fibers (sensory fibers) from the muscle spindle get stimulated. – This stimulated conduction of sensory fiber towards the CNS connects with motor fibers in the spinal cord via a single synapse. – This produces a response (H wave) gastrocnemius muscle of the leg. – With a medium intensity stimulus, both smaller motor fibers and sensory fibers are stimulated resulting in both M and H waves. – With stronger stimuli, the impulses travel centrally along the motor fibers and produce only an M wave. The amplitude of the H wave is nil or low depending upon the number of motor neurons discharged. M wave H Wave The University of Sydney Page 39 Bipotential Summary – Biopotential signals are generated by the volume conduction of current generated by excitable cells. – Biopotential signal measurements are important for clinical monitoring and diagnostics. – Example biopotential measurements are ENG, ECG, EMG, EEG, etc. – We will be discussing ECG and EEG and EMG in detail in our future classes. https://www.sciencedirect.com/topics/engineering/biopotential You will be getting hands-on experience in examining the electrophysiology of the Nerve in Week 5 Laboratory! The University of Sydney Page 40 Always Remember: General Design Criteria for Biomedical Instruments The University of Sydney Page 41 Signal Conditioning: Filter Basics for Week 2 Lab The University of Sydney Page 42 You know Biopotential now, what is next – Bioelectric signals are small and require conditioning before sending it to display. – Conditioning allows the sensor output to be ready for display. – Some of the signal conditioning processes are – Amplification – Filtering – Impedance matching – Domain converters (eg: time to frequency conversion) Signal amplification in detail will be discussed next week! The University of Sydney Page 43 Why Filtering is needed – The biosignals acquired from the human body are always subjected to various noises. – There is always a set of unwanted signals captured in addition to the desired signal. – So, filtering or removal of this unwanted signal is always necessary. – There are various filters available for filtering the various frequency components. – Low Pass Filter (LPF) – High Pass Filter (HPF) – Band Pass Filter (BPF) – Band Stop Filters (BSF) (also called Band rejection filter or notch filter if the attenuating frequency range is too narrow) The University of Sydney Page 44 Example filtering in biomedical instrumentation – Electrocardiogram records the electrical activity of the heart. Also, called recording of the cardiac activity. – LPF is used in ECG circuits for eliminating muscle noise and high-frequency signal. – Notch filters of 50Hz/60Hz to reduce the power line interference. – HPF are used to eliminate the respiratory variation and motion artefacts Israel, Steven A., et al. "ECG to identify individuals." Pattern recognition 38.1 (2005): 133-142. The University of Sydney Page 45 Filter classifications: – Filters are broadly classified into two as – Passive filters: These filters use passive components such as resistors, capacitors, and inductors. Passive low Pass Filter – Active Filters: These filters use active components such as op amps in addition to resistors and capacitors. – Almost all the filters employed in biomedical instrumentation are active filters. – We will be learning more about the op-amps and op amp-based circuits such as amplifiers and filters next week. Active low Pass Filter The University of Sydney Page 46 Basic Filter working to start this week lab: – We will be learning a simulation software LT spice lab to simulate various passive filters to understand their response to signals with various frequencies. – LPF: A low pass filter passes all input signals that have a frequency less than the cut-off frequency and attenuates/stops all input signals with a frequency higher than the cut-off frequency. Passive low Pass Filter – These filters are commonly used as an anti-aliasing filter prior to conditioning for analog-to-digital conversion. – Cut-off frequency for a low pass filter is 𝑋𝑐 𝑉𝑜𝑢𝑡 =( )𝑉𝑖𝑛 1 𝑅 + 𝑋𝑐 𝐹𝑐 = 2𝜋𝑅𝐶 – The simple RC filters are also known as first-order filters. The University of Sydney Page 47 Passive LPF frequency response: – The cut-off frequency determines the pass band and stop band. – Higher orders provide greater roll-off between the pass band and the stop band. – The output voltage for the LPF can be calculated using the formula 𝑋𝑐 𝑉𝑜𝑢𝑡 = ( )𝑉 𝑅 + 𝑋𝑐 𝑖𝑛 Xc is the capacitive reactance = 1/2πfC Where C is the capacitance in Farad, f is the signal frequency in Hz and R is the resistance in ohm. Note: The gain in db= 20 log (Vout/Vin) At cut-off frequency, the gain is -3db, which means, the output voltage will be 0.707 of the input voltage. https://en.wikipedia.org/wiki/Low-pass_filter Note: Active filters are used in Biomedical applications, we will be discussing active filters in next week’s lecture. The University of Sydney Page 48 End Don’t forget to prepare for tomorrow’s lab The University of Sydney Page 49