Chapter 6: Biopotential Amplifiers PDF
Document Details
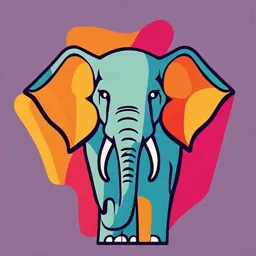
Uploaded by TriumphalEclipse
Yarmouk University
Dr. Qasem Qananwah
Tags
Related
- Week 3_S2 2024 Biomedical Instrumentation Lecture Slides PDF
- Ch5_BME420_Biopotential Electrodes PDF
- Week 2_S2 2024 Lecture Slides PDF
- BMET3802/9802 Biomedical Instrumentation Week 02 Lecture Slides 2024 PDF
- Biomedical Instrumentation (BME420) Chapter 5 Bio-potential Electrodes PDF
- BG3105 Biomedical Instrumentation - Introduction To Bioinstrumentation 2024 PDF
Summary
This document is chapter 6 of a textbook on biomedical instrumentation focusing on biopotential amplifiers and electrocardiograms. It covers topics including the need for these amplifiers, their characteristics, the ECG signal, and common problems encountered in ECG systems.
Full Transcript
Biomedical Instrumentation (BME420 ) Chapter 6: BIOPOTENTIAL AMPLIFIERS John G. Webster, 4th Edition Dr. Qasem Qananwah 4/25/2023 BME420: Biomedical Instrumentation Biome...
Biomedical Instrumentation (BME420 ) Chapter 6: BIOPOTENTIAL AMPLIFIERS John G. Webster, 4th Edition Dr. Qasem Qananwah 4/25/2023 BME420: Biomedical Instrumentation Biomedical Systems and Informatics Engineering Department 1 Chapter 6: BIO-POTENTIAL AMPLIFIERS Chapter Outline The need for Bio-potential Amplifiers Bio-potential Characteristics Electrocardiogram (ECG) Signal Electrocardiograph Block Diagram Problems Frequently Encountered in ECG System 4/25/2023 BME420: Biomedical Instrumentation Biomedical Systems and Informatics Engineering Department 2 Chapter 6: BIO-POTENTIAL AMPLIFIERS The need for Bio-potential Amplifiers Why we need for Bio-potential Amplifiers 1. To increase the amplitude of a weak electric signal of biological origin 2. Typically process voltages but in some cases also process currents 3. Isolation and protection circuitry to limit the current through the electrode to safe level 4/25/2023 BME420: Biomedical Instrumentation Biomedical Systems and Informatics Engineering Department 3 Chapter 6: BIO-POTENTIAL AMPLIFIERS The need for Bio-potential Amplifiers Requirements on Bio-potential Amplifiers 1. High input impedance -greater than 10 MΩ 2. Safety: protect the organism being studied (careful design to prevent macro and microshocks) 3. Output impedance of the amplifier should be low (for matching and minimal distortion) 4. Gain greater than 1000 (biopotentials are typically less than a millivolt) 5. High common mode rejection ratio ( High CMRR) 6. Most biopotential amplifiers are differential 4/25/2023 BME420: Biomedical Instrumentation Biomedical Systems and Informatics Engineering Department 4 Chapter 6: BIO-POTENTIAL AMPLIFIERS Bio-potential Characteristics Figure 6.16: Voltage and frequency ranges of some common biopotential signals;(From J. M. R. Delgado, "Electrodes for Extracellular Recording and Stimulation," in Physical Techniques in Biological Research, edited by W. L. Nastuk, New York: Academic Press, 1964) 4/25/2023 BME420: Biomedical Instrumentation Biomedical Systems and Informatics Engineering Department 5 Chapter 6: BIO-POTENTIAL AMPLIFIERS Electrocardiogram (ECG) Signal The electric dipole, consists of two equal and opposite charges, separated by some (usually small) distance The potential differences arising in the heart (cardiac dipoles) can be represented by electrical vectors Amplitude and direction All basic vector operations can be applied to the cardiac vectors Each depolarizing myocardial cell is in fact a dipole and thus can be represented by a Figure 6.1: Rough sketch of the dipole field of the heart when the R wave is maximal. vector = elementary vector 4/25/2023 BME420: Biomedical Instrumentation Biomedical Systems and Informatics Engineering Department 6 Chapter 6: BIO-POTENTIAL AMPLIFIERS Electrocardiogram (ECG) Signal The sum of all elementary vectors will create an instantaneous vector the potential differences generated by the heart change from moment to moment during the cardiac cycle Once a single cell is stimulated the depolarization will propagate in every direction: a propagating wave of depolarization will be created Each of these moments can be described by an instantaneous vector (with a different size and orientation) All these vectors can be brought to a single Figure 6.1: Rough sketch of the dipole field of the heart when the R wave is maximal. common point: electrical center of the heart 4/25/2023 BME420: Biomedical Instrumentation Biomedical Systems and Informatics Engineering Department 7 Chapter 6: BIO-POTENTIAL AMPLIFIERS Electrocardiogram (ECG) Signal The projection of Cardiac vector as function of time on an axis corresponding to a lead is actually the ECG in that particular lead M → Cardiac vector a → Lead vector Figure 6.2: Relationships between the two lead vectors a1 and a2 and the cardiac vector M. The component of M in the direction of a1 is given by the dot product of these two vectors and denoted on the figure by va1. Lead vector a2 is perpendicular to the cardiac vector, so no voltage component is seen in this lead. 4/25/2023 BME420: Biomedical Instrumentation Biomedical Systems and Informatics Engineering Department 8 Chapter 6: BIO-POTENTIAL AMPLIFIERS Electrocardiogram (ECG) Signal 4/25/2023 BME420: Biomedical Instrumentation Biomedical Systems and Informatics Engineering Department 9 Chapter 6: BIO-POTENTIAL AMPLIFIERS Electrocardiogram (ECG) Signal Electrodes for recording the potential changes of the heart are placed on the body surface in a standard way Potential changes recorded by specifically connected electrodes is called a lead(A pair of electrodes, or combination of several electrodes through a resistive network that gives an equivalent pair, is referred to as a lead) Each lead will be assigned with an axis and each of the axes will have an orientation The projection of the cardiac vectors as function of time on the axis corresponding to a lead is actually the ECG trace in that particular lead 4/25/2023 BME420: Biomedical Instrumentation Biomedical Systems and Informatics Engineering Department 10 Chapter 6: BIO-POTENTIAL AMPLIFIERS Electrocardiogram (ECG) Signal 1. Two different points on the body (bipolar leads) 2. One point on the body and a virtual reference point with zero electrical potential, located in the center of the heart (unipolar leads) The standard ECG has 12 leads: 1. 3 Standard Limb Leads 2. 3 Augmented Limb Leads 3. 6 Precordial Leads Limb leads Precordial leads Bipolar Lead I, II, III (Standard limb leads) - Unipolar aVR, aVL, aVF (augmented limb leads) V1-V6 Plane Frontal Plane Transverse Plane (The axis of a particular lead represents the viewpoint from which it looks at the heart( 4/25/2023 BME420: Biomedical Instrumentation Biomedical Systems and Informatics Engineering Department 11 Chapter 6: BIO-POTENTIAL AMPLIFIERS Electrocardiogram (ECG) Signal Figure 6.3: Cardiologists use a standard notation such that the direction of the lead vector for lead I is 0°, that of lead II is 60°, and that of lead III is 120°. An example of a cardiac vector at 30° with its scalar components seen for each lead is shown. 4/25/2023 BME420: Biomedical Instrumentation Biomedical Systems and Informatics Engineering Department 12 Chapter 6: BIO-POTENTIAL AMPLIFIERS Electrocardiogram (ECG) Signal Generation of ECG signal in Einthoven limb leads 4/25/2023 BME420: Biomedical Instrumentation Biomedical Systems and Informatics Engineering Department 13 Chapter 6: BIO-POTENTIAL AMPLIFIERS Electrocardiogram (ECG) Signal Generation of ECG signal in Einthoven limb leads 4/25/2023 BME420: Biomedical Instrumentation Biomedical Systems and Informatics Engineering Department 14 Chapter 6: BIO-POTENTIAL AMPLIFIERS Electrocardiogram (ECG) Signal Electrocardiograph Leads Wilson central terminal Three limb electrodes connected through equal-valued resistors to a common node Augmented leads some nodes disconnected increase the amplitude of measurement using Figure 6.4: Connection of electrodes to the body to obtain Wilson’s central terminal 4/25/2023 BME420: Biomedical Instrumentation Biomedical Systems and Informatics Engineering Department 15 Chapter 6: BIO-POTENTIAL AMPLIFIERS Electrocardiogram (ECG) Signal Example 6.1: Show that the voltage in lead aVR is %50 greater than that in lead VR at the same instant. Solution: Considering the connections for aVR and VR, we can draw the equivalent circuits. The voltages between each limb and ground va , vb , vc Figure E6.1 (a) aVR (b) VR (c) Simplified circuit of (a) 4/25/2023 BME420: Biomedical Instrumentation Biomedical Systems and Informatics Engineering Department 16 Chapter 6: BIO-POTENTIAL AMPLIFIERS Electrocardiogram (ECG) Signal R should be very high (~5 MΩ) or buffers (voltage followers) should be used between each electrode so that the loading of any particular lead will be minimal. Figure 6.5: (a), (b), (c) Connections of electrodes for the three augmented limb leads, (d) Vector diagram showing standard and augmented lead-vector directions in the frontal plane. 4/25/2023 BME420: Biomedical Instrumentation Biomedical Systems and Informatics Engineering Department 17 Chapter 6: BIO-POTENTIAL AMPLIFIERS Electrocardiogram (ECG) Signal Figure 6.6 (a) Positions of precordial leads on the chest wall, (b) Directions of precordial lead vectors in the transverse plane. 4/25/2023 BME420: Biomedical Instrumentation Biomedical Systems and Informatics Engineering Department 18 Chapter 6: BIO-POTENTIAL AMPLIFIERS Electrocardiogram (ECG) Signal 6 limb leads – define electrical activity in frontal plane 6 precordial leads – define electrical activity in transverse plane The 3 augmented leads compare one limb electrode to the average of the other two. (aVR, aVL, aVF). Leads are made of a combination of electrodes that form imaginary lines in the body along which the electrical signals are measured. 4/25/2023 BME420: Biomedical Instrumentation Biomedical Systems and Informatics Engineering Department 19 Chapter 6: BIO-POTENTIAL AMPLIFIERS Electrocardiograph Block Diagram Figure 6.7: Block diagram of an electrocardiograph 4/25/2023 BME420: Biomedical Instrumentation Biomedical Systems and Informatics Engineering Department 20 Chapter 6: BIO-POTENTIAL AMPLIFIERS Problems Frequently Encountered 1. Frequency Distortion a. High frequency distortion if band pass filter has 0 – 25 Hz response b. Low frequency distortion if band pass filter has 1 – 150 Hz response In low frequency distortion, the baseline is no longer horizontal, especially immediately following any event in the tracing. Monophasic waves appear to be biphasic. 4/25/2023 BME420: Biomedical Instrumentation Biomedical Systems and Informatics Engineering Department 21 Chapter 6: BIO-POTENTIAL AMPLIFIERS Problems Frequently Encountered 2. Saturation or Cutoff Distortion The peaks of QRS can be cutoff because the output of the amplifier cannot exceed the saturation voltage or even the lower portion of the S wave will be cutoff. 3. Ground Loop 4/25/2023 BME420: Biomedical Instrumentation Biomedical Systems and Informatics Engineering Department 22 Chapter 6: BIO-POTENTIAL AMPLIFIERS Problems Frequently Encountered 4. Open Lead Wire Always make sure that the electrodes are in good contact. Otherwise, usually high potentials can often be induced in the open wire as a result of electric field. Coming from a power line, it causes a wide-constant- amplitude deflection on the ECG trace. 4/25/2023 BME420: Biomedical Instrumentation Biomedical Systems and Informatics Engineering Department 23 Chapter 6: BIO-POTENTIAL AMPLIFIERS Problems Frequently Encountered 5. Artifacts From Large Electric Transients Occurred due to cardiac defibrillation Figure 6.8: Effect of a voltage transient on an ECG recorded on an electrocardiograph in which the transient causes the amplifier to saturate, and a finite period of time is required for the charge to bleed off enough to bring the ECG back into the amplifier's active region of operation. This is followed by a first-order recovery of the system. 4/25/2023 BME420: Biomedical Instrumentation Biomedical Systems and Informatics Engineering Department 24 Chapter 6: BIO-POTENTIAL AMPLIFIERS Problems Frequently Encountered 6. Interference from Electric Devices Figure 6.9: (a) 60 Hz power-line interference, (b) Electromyographic interference on the ECG. 4/25/2023 BME420: Biomedical Instrumentation Biomedical Systems and Informatics Engineering Department 25 Chapter 6: BIO-POTENTIAL AMPLIFIERS Problems Frequently Encountered 6. Interference from Electric Devices Example: For a 9 m cable, id ≈ 6 nA , the electrodes impedance can differ as much as 20kΩ. Then, Figure 6.10 A mechanism of electric-field pickup of an electrocardiograph resulting from the power line. Coupling capacitance between This can be minimized by shielding the leads. the hot side of the power line and lead wires causes current to flow through skin–electrode impedances on its way to ground. 4/25/2023 BME420: Biomedical Instrumentation Biomedical Systems and Informatics Engineering Department 26 Chapter 6: BIO-POTENTIAL AMPLIFIERS Problems Frequently Encountered 6. Interference from Electric Devices Look at this situation or example: Figure 6.11 Current flows from the power line through the body and ground impedance, thus creating a common-mode voltage everywhere on the body. Zin is not only resistive but, as a result of RF bypass capacitors at the amplifier input, has a reactive component as well. This is noticeable on the ECG and very objectionable on EEG. 4/25/2023 BME420: Biomedical Instrumentation Biomedical Systems and Informatics Engineering Department 27 Chapter 6: BIO-POTENTIAL AMPLIFIERS Problems Frequently Encountered 6. Interference from Electric Devices Figure 6.12: Magnetic-field pickup by the electrocardiograph (a) Lead wires for lead I make a closed loop (shaded area) when patient and electrocardiograph are considered in the circuit. The change in magnetic field passing through this area induces a current in the loop, (b) This effect can be minimized by twisting the lead wires together and keeping them close to the body in order to subtend a much smaller area. 4/25/2023 BME420: Biomedical Instrumentation Biomedical Systems and Informatics Engineering Department 28 Chapter 6: BIO-POTENTIAL AMPLIFIERS Transient Protection of Electrocardiograph Figure 6.13: A voltage-protection scheme at the input of an electrocardiograph to protect the machine from high-voltage transients. Circuit elements connected across limb leads on left-hand side are voltage-limiting devices. Figure 6.14: Voltage-limiting devices (a) Current– voltage characteristics of a voltage-limiting device. (b) Parallel silicon-diode voltage-limiting circuit. (c) Back- to-back silicon zener-diode voltage-limiting circuit. (d) Gas-discharge tube (neon light) voltage-limiting circuit element. 29 4/25/2023 BME420: Biomedical Instrumentation Biomedical Systems and Informatics Engineering Department Chapter 6: BIO-POTENTIAL AMPLIFIERS Transient Protection of Electrocardiograph 4/25/2023 BME420: Biomedical Instrumentation Biomedical Systems and Informatics Engineering Department 30 Chapter 6: BIO-POTENTIAL AMPLIFIERS Transient Protection of Electrocardiograph A transient-voltage-suppression (TVS) diode is an electronic component used to protect sensitive electronics from voltage spikesinduced on connected wires 4/25/2023 BME420: Biomedical Instrumentation Biomedical Systems and Informatics Engineering Department 31 Chapter 6: BIO-POTENTIAL AMPLIFIERS Transient Protection of Electrocardiograph 4/25/2023 BME420: Biomedical Instrumentation Biomedical Systems and Informatics Engineering Department 32 Chapter 6: BIO-POTENTIAL AMPLIFIERS Driven-Right-Leg (DRL) system Figure 6.15 Driven-right-leg circuit for minimizing common-mode interference. The circuit derives common-mode voltage from a pair of averaging resistors connected to v3 and v4 in Figure 3.5. The right leg is not grounded but is connected to output of the auxiliary op amp. 4/25/2023 BME420: Biomedical Instrumentation Biomedical Systems and Informatics Engineering Department 33 Chapter 6: BIO-POTENTIAL AMPLIFIERS Driven-Right-Leg (DRL) system Example: Figure E6.3 Equivalent circuit of driven-right-leg system 4/25/2023 BME420: Biomedical Instrumentation Biomedical Systems and Informatics Engineering Department 34 Chapter 6: BIO-POTENTIAL AMPLIFIERS Typical Electrocardiograph System Design Figure 6.18: This ECG amplifier has a gain of 25 in the dc-coupled stages. The high-pass filter feeds a noninverting- amplifier stage that has a gain of 32. The total gain is 25 X 32 = 800. When mA 776 op amps were used, the circuit was found to have a CMRR of 86 dB at 100 Hz and a noise level of 40 mV peak to peak at the output. The frequency response was 0.04 to 150 Hz for ±3 dB and was flat over 4 to 40 Hz. A single op amp chip, the LM 324, that contains four individual op amps could also be used in this circuit reducing the total parts count. 4/25/2023 BME420: Biomedical Instrumentation Biomedical Systems and Informatics Engineering Department 35 Chapter 6: BIO-POTENTIAL AMPLIFIERS Cardiotachometer 4/25/2023 BME420: Biomedical Instrumentation Biomedical Systems and Informatics Engineering Department 36 Chapter 6: BIO-POTENTIAL AMPLIFIERS Cardiotachometer 4/25/2023 BME420: Biomedical Instrumentation Biomedical Systems and Informatics Engineering Department 37 Chapter 6: BIO-POTENTIAL AMPLIFIERS Cardiotachometer 38 4/25/2023 BME420: Biomedical Instrumentation Biomedical Systems and Informatics Engineering Department Chapter 6: BIO-POTENTIAL AMPLIFIERS Fetal ECG System Figure 6.22 Typical fetal ECG obtained from the maternal abdomen. F represents fetal QRS complexes; M represents maternal QRS complexes. Maternal ECG and fetal ECG (recorded directly from the fetus) are included for comparison. (From "Monitoring of Intrapartum Phenomena," by J. F. Roux, M. R. Neuman, and R. C. Goodlin, in CRC Critical Reviews in Bioengineering, 2, pp. 119-158, January 1975, © CRC Press. Used by permission of CRC Press, Inc.) 4/25/2023 BME420: Biomedical Instrumentation Biomedical Systems and Informatics Engineering Department 39 Chapter 6: BIO-POTENTIAL AMPLIFIERS Fetal ECG System Figure 6.23 Block diagram of a scheme for isolating fetal ECG from an abdominal signal that contains both fetal and maternal ECGs. (From "Monitoring of Intrapartum Phenomena," by J. F. Roux, M. R. Neuman, and R. C. Goodlin, in CRC Critical Reviews in Bioengineering, 2, pp. 119–158, January 1975, © CRC Press. Used by permission of CRC Press, Inc.) 4/25/2023 BME420: Biomedical Instrumentation Biomedical Systems and Informatics Engineering Department 40 Chapter 6: BIO-POTENTIAL AMPLIFIERS Fetal ECG System 4/25/2023 BME420: Biomedical Instrumentation Biomedical Systems and Informatics Engineering Department 41 Chapter 6: BIO-POTENTIAL AMPLIFIERS Cardiac Monitor System Figure 6.24 Block diagram of a cardiac monitor. 4/25/2023 BME420: Biomedical Instrumentation Biomedical Systems and Informatics Engineering Department 42 Chapter 6: BIO-POTENTIAL AMPLIFIERS Cardiac Monitor System 4/25/2023 BME420: Biomedical Instrumentation Biomedical Systems and Informatics Engineering Department 43 Chapter 6: BIO-POTENTIAL AMPLIFIERS Cardiac Monitor System Figure 6.25 Block diagram of a system used with cardiac monitors to detect increased electrode impedance, lead wire failure, or electrode fall-off. 4/25/2023 BME420: Biomedical Instrumentation Biomedical Systems and Informatics Engineering Department 44 Chapter 6: BIO-POTENTIAL AMPLIFIERS Electrode Failure Circuitry 4/25/2023 BME420: Biomedical Instrumentation Biomedical Systems and Informatics Engineering Department 45 Chapter 6: BIO-POTENTIAL AMPLIFIERS Electrode Failure Circuitry 4/25/2023 BME420: Biomedical Instrumentation Biomedical Systems and Informatics Engineering Department 46 And this concludes… 4/25/2023 BME420: Biomedical Instrumentation Biomedical Systems and Informatics Engineering Department 47