Eyes PDF
Document Details
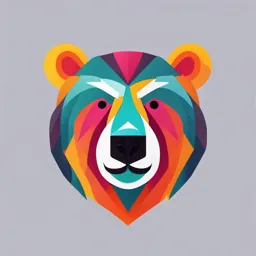
Uploaded by GlamorousWashington
Young Harris College
Tags
Related
- Special Senses: Eye and Vision PDF
- Special Senses PDF
- Special Senses in Human Anatomy PDF
- BIOL 232 Biology II: Anatomy of Sense Organs Lecture Outline PDF
- MLS 111L Human Anatomy and Physiology With Pathophysiology PDF
- Human Anatomy and Physiology Eleventh Edition - Chapter 15 Part A - Special Senses PDF
Summary
This is a lecture on the special senses, focusing on vision. It covers the accessory structures of the eyes, like eyebrows and eyelids, and the lacrimal apparatus. It also details the anatomy and physiology of focusing and how light creates electrical signals.
Full Transcript
NOTE: Transcripts are made from the auto-generated Lecture Captions, so are not edited for grammar/spelling. Lecture 21 - Special Senses: Vision Video 1 Introduction Welcome to the next online lecture. In this lecture, we're going to continue our look at the special senses by looking at vision....
NOTE: Transcripts are made from the auto-generated Lecture Captions, so are not edited for grammar/spelling. Lecture 21 - Special Senses: Vision Video 1 Introduction Welcome to the next online lecture. In this lecture, we're going to continue our look at the special senses by looking at vision. So we're going to talk about how we can take light information from our external environment and turn that into an electrical signal that our brain can interpret. We'll also talk about things like, why does your nose run when you cry? And what are those things that sometimes you see moving through your visual field, but don't actually exist in the real world. Also, we'll talk about things like why you can't see colour in dark situations or at nighttime. So today we're going to start by looking at some of the external structures of the eye that we'll look at the anatomy of the eye, and we'll talk about the physiology of focusing. And then we'll look at how we actually create those electrical signals using light information. So let's get started. Slide 1 The material for this online lecture module can be found in chapter 15 of your textbook. Specifically, we are going to be focusing on Section 15.3 called the visual system. Slide 2 We will begin by looking at the accessory structures of the eye, the eyebrows, as well as the eyelashes, that help protect the eye from foreign objects, perspiration, and help shade the eyes from the sun. The second accessory structure for the eye is the palpebrae, commonly known as the eyelids. Palpebrae is plural and palpebra is singular. The upper eyelid is called the superior palpebra, and the lower eyelid is called the inferior palpebrae. Between the two palpebrae is the palpebral fissure, which is basically the opening for the eye or we can actually see the eyeball, the palpebrae shade the eyes during sleep. They protect them from excessive late and foreign objects, and they spread lubricating secretions over the eye, commonly known as tears. The location where the two palpebrae meet are known as the canthus or commissure. We have a medial commissure and a lateral commissure. At the medial commissure, we have a pink mound called the lacrimal caruncle, or just caruncle for short. The caruncles contains modified oil and sweat glands and these produce that whitish material that often collects in that area. Sometimes overnight this material dries and as a kid we would call this the sleep in our eyes. And other accessory structure is the conjunctiva, which is a mucous membrane that forms the inner lining of the eyelid. It attaches at the palpebral fissure, so on the anterior openings of the eyelid, and it actually lines the inside of the eyelid and then folds back on itself to cover the white of the eye. So in this image at the bottom here, we're looking at a lateral view of a cut through the eyeball and you can see the eyelid. And then this pink membrane is lining the inside of the eyelid and then it comes back up, folds on itself and attaches onto the white portion of the eye up to the level of the cornea, which is this bulged region of the eyeball, which we'll talk about more in a second. The portion lining the eyelid is called the palpebral conjunctiva, and the portion lining the white of the eye is called the bulbar conjunctiva. Basically the conjunctiva forms a pocket to stop debris or objects, like if you wear contact lenses, or even eyelashes from entering the posterior portion of the eye where we have the optic nerve and other blood vessels. So it's protecting the structures at the back of the eye from debris moving in from our external environment and causing damage. The conjunctiva can also respond to irritants. The irritants can cause inflammation of the conjunctiva, and we get something known as conjunctivitis. Common conjunctivitis will lead to bloodshot eyes. Some conjunctivitis can also be caused by a bacterial infection, which is commonly referred to as pink eye. On this image, we can also see the iris or coloured part of the eye, and the pupil, which is the darken spot in the middle of the iris that acts as a window to let light into the eye, we'll talk more about those in a moment. Slide 3 The next accessory structure that we're going to be talking about is the lacrimal apparatus. It's actually a collection of structures that are involved in the production of tears. The lacrimal gland produces the tears and releases them onto the surface of the eye through the lacrimal ducts located on the superior lateral side of the eye. The tears then move medially and inferiorly towards the medial commissure. Most of the fluid that's released actually evaporates throughout the day, but if there's excess tears produced, they collect on the medial corner of the eye and enter in one of two small openings called the puncta. These are located on both the upper and lower eyelids, and from there they move into small passageways known as the lacrimal canaliculi. There's a superior canaliculus, so the canaliculus is singular, and canaliculi is plural, and an inferior canaliculus connecting to each puncta respectively. This leads to the lacrimal sac, and then the tears then pass into the nasolacrimal duct and drain into the nasal cavity. This explains why your nose runs when you cry. The excessive tears that are produced when you're crying collect into this lacrimal sac, drain into the nasolacrimal duct, then drain into your nasal cavity where it creates your runny nose. Slide 4 The final accessory structures that we're going to look at are the extrinsic muscles of the eye, the six muscles that control the movement of the eyeball. And the first image we have a superior view of the orbit of the eye, and then we have the lateral view of the right eye in figure b, and then in this last image, which comes from our old textbook, you can see the movement patterns for each of these muscles of the eye. You may remember seeing the names of some of these muscles in the cranial nerves lecture, and now we're going to go over there and a little more detail. There are four rectus muscles, each attached to the anterior portion of the eyeballs sclera, or the white part of the eye, and then extend towards the posterior of the eyeball socket or the eye orbit and attach onto the bone. There's a superior, a inferior, a medial, and a lateral rectus muscle. So their names are based on their attachment point onto the eyeball. The superior rectus is attached onto the top of the eye, the lateral is on the lateral side, the medial is on the medial side, and the inferior attaches to the bottom of the eye. When these muscles contract, they contract along their length. So this allows for movement of the eyeball up, down, left and right. Also because the superior rectus, inferior rectus are attached at a slight angle, they can also both contribute to medial movement of the eye. There are also two oblique muscles, the inferior oblique and the superior oblique. These are involved in rotation of the eye. The superior oblique runs through a structure known as the trochlea, this acts as a pulley and redirects the line of pull from that muscle, allowing for inferior lateral rotation of the eye. The inferior oblique has a different orientation. It doesn't require a trochlea, so it acts by rotating the eye by moving it towards the superior lateral side. Slide 5 Moving onto the structures of the eye. The eye is made up of three main layers, also referred to as coats or tunics. We'll look at them from most superficial to deep on an upcoming image, but I will list them here first. The outermost tunic is called the fibrous tunic, which makes up the white portion of the eye. The posterior portion of the fibrous tunic is called the sclera. The sclera is a tough, thick connective tissue layer which helps maintain the shape of the eye, it also provides an attachment point for those extrinsic muscles of the eye. It will also provide protection for the structures of the eye that are deeper to it as well. The anterior portion of the fibrous tunic, is the cornea, which is a transparent, curve shaped structure that helps us to focus light. It's also a vascular, which means it has no blood vessels or blood supply. So therefore it gets some of its oxygen from the outside air. That's why if you have contact lenses, they're supposed to be breathable to allow air to move through or you have to remove your contact lenses regularly to allow the cornea to get some oxygen from the outside air. The next deepest layer is called the vascular tunic because it contains a lot of blood vessels and it also contains the cells which contain melanin. Melanin is a pigment that makes the inner surface of the eye appear black, which reduces light from reflecting around inside the eye. It helps us to improve visual acuity or the sharpness of our images. The posterior portion of this layer, which is associated with the sclera, is called the choroid. Here we have the rich blood supply. The anterior portion contains the ciliary body as well as the iris. The ciliary body contains the smooth muscles which are involved in changing the shape of the lens. And the iris contains smooth muscles which change the diameter of the pupil. These muscles are referred to as the intrinsic muscles of the eye. We'll look more closely at those structures and how they work in a moment. The innermost or deepest layer is called the retina, which is also called the neural tunic. It's located on the posterior portion of the eye, only up to the level of the ciliary body. It contains specialized receptor cells called photoreceptor cells. There are two types of photoreceptor cells. There are the cone cells which are involved in colour vision as well as visual acuity, so seeing sharper, clearer images. And rod cells which are used for low light conditions and seeing black and white. We have many, many more rods versus cones, about 6 to 7 million for cones and a 120 million for rods, and we'll look at the distribution of these on an upcoming slide. It's these photoreceptor cells that turn our light stimulus into an action potential or a signal that we can send to the brain. Slide 6 I've added this slide in to highlight this table that came from our previous textbook. It gives a nice overview of the structures that we're going to be talking about and colour codes the various layers or tunics. So let's take a pause here and then we'll return and talk more about the anatomy of the eye. Video 2 Slide 7 Welcome back. This image is showing all of the internal structures of the eye that we just started to talk about, including the tunics. In the fibrous tunic, you can see the curved shaped cornea on the anterior surface of the eye, surrounded by the sclera or tough connective tissue layer that makes the white portion of the eye. You can see here the bulbar conjunctiva. It's actually continuous with our epithelial layer of our cornea, but it's only referred to as a conjunctiva up to the level of the cornea itself. This is showing the bulbar portion. But you can imagine what the palpebral conjunctiva would look like if it folds upon itself underneath the eyelid. In the vascular tunic, we can see the choroid layer that happens on the posterior side of the eye that contains melanin to help absorb the scattered light in the eye. You can also see the numerous blood vessels in this layer represented by the red and blue dots. On the anterior portion of this layer we have the ciliary body. It contains muscles called the ciliary muscles and extensions towards the lens called the ciliary processes. These attached to the lens via the zonular fibers or suspensory ligaments. So if you can imagine the muscles within the ciliary body when they contract will change the tension on the suspensory ligaments and alter the shape of the lens. Notice how the ciliary body and ligaments wrap all the way around the lens. We'll see a frontal image of this in a moment as well. Another anterior structure of this layer is the iris, which is the coloured part of the eye. It also contains smooth muscles which alter or change the diameter of the pupil. Most of you are familiar with the pupil. It's the black circle in the center of the iris, but it's really an opening and it just appears black because of the melanin in the choroid layer behind it. The deepest layer in the eye is the retina, where we have this specialized photoreceptor cells, the rods and cones. Within this layer we have some regions where these photoreceptor cells are highly organized, called the macula lutea and the fovea centralis. This is the region that provides us with the clearest images. So we typically want to move our eyes, although the light entering the eye is directed to these regions, we'll talk more about them in a moment. Notice this area at the back of the two eyes where the three layers are broken. This is a region known as the optic disk or the blind spot, because this is a hole in the retina that allows for the axons of the optic nerve to lead the eye, along with blood vessels that enter and exit the eye, called the central retinal artery and vein. There are also three chambers in the eye, two of which are in the anterior cavity, just anterior to the lens, and one in the posterior portion or posterior to the lens. The anterior cavity of the eye is broken into two chambers. There's the anterior chamber, which lies between the cornea and the iris, as well as the posterior chamber, which lies between the iris and the lens and the suspensory ligaments. The anterior cavity is filled with a fluid called the aqueous humor, which is produced by the ciliary processes and is released into these two anterior compartments. The aqueous humor helps to maintain interocular pressure and to help maintain the shape of the eye. It also provides nutrients for the cornea and the lens, which are both avascular. So the cornea can receive some of its oxygen from the external air, but the rest of its oxygen and nutrients come from the aqueous humor that's located in the anterior and posterior chamber. The lens also gets its oxygen and nutrients from this aqueous humor because it doesn't have its own blood supply either. The aqueous humor also helps with the refraction or bending of light as it enters the eye, like their cerebral spinal fluid, as it gets produced, it also gets reabsorbed. It gets reabsorbed into these little sinuses that you can see here at the edge of the cornea. You don't need to know the names of them, but you just need to know that as we produce the aqueous humor, it will circulate through the two chambers and be reabsorbed at the edge of the cornea. So basically as it circulates through these chambers, is providing the nutrients and oxygen that these avascular tissues require. It has a very high turnover rate, approximately every 90 minutes it replenishes itself or every hour and a half. If we get a blockage of the sinuses, it begins to build up within the eye and we get a disorder known as glaucoma, or an increase in interocular pressure that can cause damage to the eye. If left untreated, it will eventually lead to blindness. The posterior chamber is called the vitreous chamber because it's filled with the vitreous humor or vitreous body. It's a jelly like substance which has a very slow turnover rate compared to the aqueous humor. It also helps to keep the shape of the eye, but more importantly, it holds the retina layer flat against the choroid so that we can have nice clear images. The slow turnover of the vitreous body can cause a build up of some debris that naturally forms from cell death in this chamber. So these dead cells will float around within the vitreous body. Normally we have phagocytes that will engulf the debris and break it down get rid of it. But as you get older, these phagocytes can't keep up as well with the demand. And you may notice the debris floating through your visual field. These are sometimes referred to simply as floaters. So you'll notice sort of black spots floating through your visual field that simply just normal debris that the phagocytes within your vitreous body have not degraded yet. The last structure here is the lens. The lens is actually a fairly amazing structure because it's the only solid tissue in the body that's transparent. It achieves this in a very interesting way. As the lens is developing it contains organelles and other features, just like all cells. But the lens begins a self-destruct program that starts the breakdown of the contents within these cells. Normally, if we start this self-destruct program and break down the organelles within our cells, the cells would die. But something about the lens causes the self-destruct program to stop right before the cell dies. Therefore, our lens cells exist with no organelles or internal structures. This allows the cell to become clear of any structures that may scatter the light rays. It's interesting because researchers are actually studying the lenses ability to stop cell death right before this all dies and tried to apply this to diseases that actually have programmed cell death, like Alzheimer's disease or Parkinson's disease. So looking at all the structures of the eye now you can see that light entering into the eye first moves through the cornea, then the aqueous humor in the anterior cavity, and then the lens, and then the vitreous body before hitting the retina and the region of the macula lutea and the fovea centralis. All of these structures are involved in bending the light to help focus the image on the retina. Slide 8 So the next special sense we're going to talk about is the sense of taste, also known as gustation. Like we did with the olfactory area, we first need to look at the anatomy of the structures where these specialized taste receptors are going to be found. Now the majority by far of the specialized taste receptors are going to be found on the tongue. And the tongue of course, is inside the oral cavity. So in this image we're looking at the surface of the tongue. This would be the anterior portion and this is the posterior portion. The majority of this posterior portion is actually deep within your throat, so you can't actually see it when you stick your tongue out. Most of what you can see ends at about this level right here. Now while the specialized taste receptors are primarily found in the tongue, there also are some of these receptors in other portions of the oral cavity, specifically on the roof of your mouth, on your lips and in your throat as well. So know that you can have some of these specialized receptors elsewhere, but we're going to be focusing primarily on the tongue because that's where most of them are going to be located. The other region of the tongue that we haven't talked about yet is this really posterior region known as the epiglottis. So this is basically on the base of the tongue and it's deep within your throat. You can't really see it when you're trying to look in the mirror, but your epiglottis is the structure that covers over your windpipe when you're swallowing. So we will talk more about this structure in term two with digestion. But for now, just know that this is the structure at the base of the tongue because there will also be some of our specialized taste receptors found in that region as well. So the surface of the tongue is covered with small epithelial projections called papillae or papilla (singular). These epithelial projections differ depending where on the tongue you're looking. And they also differ in their function and the amount of taste receptors that they have in them as well. So there are four main types of papillae. The first type is known as the vallet papillae. So the vallet papillae are by far the largest of the papillae. And if you stick your tongue out really far and you look in the mirror, these are the really large bumps that you see at the back of your tongue. In fact, they form a V on the posterior portion of the tongue. So the largest of all of the epithelium projections, in fact, we have anywhere from eight to 12 of them in total, So you can sort of see them back here, it's showing about eight of these vallet papillae. And the reason they're called vallet papillae is because they make this V shape and the posterior aspect of the tongue. But notice what the papillae actually look like. So the papillae, as I said, are epithelial projections. So the outside layer is epithelium, and then it sort of folds in on itself to make these little pockets. In the pockets is where the taste receptors are going to be found, and for our special sense of taste and the taste receptors are found in the taste buds. So depending on the type of papillae will have a varying number of taste buds. In the vallet papillae, as I said, we only have about eight to 12 total vallet papillae, so not very many, but they do have a large number of taste buds. In fact, they can have anywhere from a 100 to 300 taste buds within each papillae. So they do contain a large number of those taste buds. So that's the vallet papillae. The next type of papillae are the foliate papillae. So the foliate papillae are found on the sides of the tongue, so the lateral surface of the tongue. Their named the foliate papillae because they take on the shape of a leaf or foliage. They're structured a little bit more differently and they're quite a bit smaller than our vallet papillae. And we have a larger number of them, but you can see that they still form these little pockets. They solve a layer of epithelium and they still contain taste buds. Now in the foliate papillae, this type of papillae, the taste buds do start to degenerate with time or age. So we have a larger number as children and then they start to deteriorate over time. So this papillae won't be quite as active when you're older because the taste buds within them will no longer exist. By By far, the largest number of papillae are going to be on the surface of the tongue. So the first type that we're going to talk about that's on the surface of the tongue are the fungiform papillae. So the fungiform papillae get their name from the fact that they look like little mushrooms. So again, structurally, they're all fairly similar in the fact that they create these little pockets, that's why there are projections of the epithelium, but they will have varying numbers of taste buds. And in fact, the fungiform papillae only have about five taste buds per papillae, but there's so many more papillae in total that overall they're going to have a larger number of the taste buds in general, mixed in or scattered amongst the fungiform papillae we have the last of the papillae called the filiform papillae. So the filiform papillae gets its name from the fact that it's shaped like a flame. Now what makes the filiform papillae different is that it doesn't have any taste buds. In fact, the filiform papillae, its main job is to create the rough surface of your tongue, and that's going to help you to manipulate your food when you're eating. So these two are basically going to be scattered throughout this superior surface of the tongue. Now if you wanted to look at this in an electron microscope, this is what it looks like. So the filiform papillae are basically these rough shaped little projections, whereas the fungiform papillae are much broader, more mushroom shaped and they will have the taste buds, whereas these filiform papillae do not. So overall, with all of the papillae and all of the taste buds, on average, we have about 10 thousand taste buds, but as I said, this will decrease with age, especially in that region of the foliate papillae. Also the other regions as well, but the foliate papillae decay at a more rapid rate and tend to be gone by adulthood. This is a close-up look of the anterior portion of the vascular tunic. This is a sagittal section, and this is showing a front view of the ciliary body surrounding the lens. Here you can see the sclera layer as well as the cornea. You can also see a little bit of that conjunctiva again, so that's the bulbar conjunctiva. For the vascular tunic, you can see the ciliary body as well as the iris. In these structures, the smooth muscle is oriented in two ways. The outer muscles are oriented in a radial manner, and the inner muscles are oriented in a circular orientation. The muscles that make up the ciliary body form what's called the ciliary ring. On the innermost side of the ciliary ring are the ciliary processes which are attached to the lens by the suspensory ligaments or zonular fibers. They also make the aqueous humor, as it begins at the ciliary processes, it fills the posterior chamber, then moves through the pupil up into the anterior chamber, fills the anterior chamber, and then gets reabsorbed into those sinuses that I mentioned earlier. The iris is anterior to our ciliary processes and our suspensory ligaments, and it partially covers over the lens depending on the contraction state of the iris and the dilation of our pupil. I added this other image here to show how the muscles of the ciliary body contract. When the ciliary muscles contract, they change the shape of the lens. So there's a ring of smooth muscle that's oriented radially on the outside of this structure. And then there's a ring of smooth muscle in this center that's oriented circularly. Remember that muscle always contracts along its length and that's important when we're talking about what happens when the muscle contracts. When the muscles at rest, the diameter of this ring is very large, and the suspensory ligaments are actually pulled tight. This pulls on the lens which is naturally more spherical and it flattens it out. When the circular muscle contracts, what happens is it actually decreases the diameter of this circle. This results in decrease tension on the suspensory ligaments. So imagine the muscle contracts, the diameter gets smaller and the tension between the suspensory ligament and the lens reduces, or the suspensory ligament is slacken somewhat, and the lens is now able to bounce back to it's more spherical natural form using the elastic properties that are part of the lens. Slide 9 These images demonstrate this concept a little bit better. The difference is that it's showing muscles of the iris, but imagine the muscles of the ciliary body would act in a similar way. When the circular muscle contracts, it reduces the diameter of the circle. And when the radial muscle contracts, it increases the diameter of the circle. When this happens in the ciliary body, it changes the shape of the lens. But when we're talking about the smooth muscle of the iris, it changes the diameter of the pupil. In the iris, the circular smooth muscle is called the sphincter pupillae. It responds to bright light and is activated by the parasympathetic nervous system to constrict the pupil and make the diameter smaller. The radiating muscle is called the dilator pupillae. When the radiating muscle contracts, we get an increase in the diameter of the pupil causing dilation. Hence it's called the dilator pupillae. This allows more light to enter the eye when there's a dim light condition and is caused by the sympathetic nervous system. So let's take a little pause here, we'll try out some more practice questions and then we'll come back and look at the retina. Video 3 Slide 10 This slide is showing a retinal image that's been taken with an ophthalmoscope. It shows the anterior view of the retinal layer through the pupil. You can see that there's an extensive vasculature as well as some other distinctive features. The dark center spot in this image is the macula lutea, which in its very center has the fovea centralis. This is a region of the retina where we want to focus the light entering the eye to achieve the greatest visual acuity. It's essentially our target when the light is entering the eye, since it's located close to the center of the retina, this is why we see objects most clearly when we look directly at them. The fovea centralis is actually a small depression in the macular region of the retina, and it contains only cone photoreceptor cells. The macula contain some rods, but mostly cones, and because of the high density of cones, this is why this region of the eye has the greatest visual acuity. As we move out from the macular reagent into the periphery of the retina, we get a greater and greater density of rod cells. The other structure I want to highlight on this image is the optic disk, which is represented here by the yellowish circle. This is where the optic nerve and blood supply enters the eye, and because this area has no photoreceptor cells, it's referred to as the blind spot. There's actually a little test in your textbook where you can test for the blind spot in your visual field. You normally don't notice the blind spot because your brain actually fills in the image for you, but since there's no action potentials coming from that area, there is technically no visual stimulation or information going to the brain, so the brain has to fill that information in. Slide 11 This slide shows the retinal layer in more detail. There are two main layers of the retina. The outer layer, or more superficial layer, is called the pigmented layer. It's comprised of pigment cells which are filled with melanin. This makes a black matrix which is essential for enhancing visual acuity and decreasing this scattering of light which enters the eye. This layer adheres very closely to our choroid layer and our vascular tunic, which I've already talked about, also contains this melanin. The sensory part of our photoreceptor cells are located adjacent to this pigmented layer. So this is where we're actually detecting the light signal. The inner layer, or next deepest layer is called the neural layer, and it also has several sublayers, and we'll talk about three of those. The photoreceptor layer is where we have our specialized photoreceptor cells called the rods and cones. These have modify dendrite ends that are sensitive to light and are oriented next to the pigment cells in that retinal layer. You can also see the rod and cone cells in this electron micrograph, and the fact that they get their name from their shape. These can synapse with the bipolar cells in the next layer, which is the bipolar cell layer. So remember this was one of the types of neurons that we talked about before, the bipolar cells. The bipolar cells can then synapse with the ganglion cells of the ganglion layer. The ganglion cells are the ones that actually synapse with the optic nerve itself. So if you want to orient yourself to what this diagram is actually looking at, we actually have the optic nerve entering into the posterior or center of the eye. And these neural cells are actually moving backwards towards the posterior portion of the eye. The light would actually move through the optic nerve axons, through all of these neural layers before it's detected at the level of the pigmented layer. But then when it's detected in our photoreceptor cells, the action potentials are actually moving back down through the photoreceptor cells, through the bipolar layer, into the ganglionic layer before it reaches the optic nerve. Slide 12 Before we move on to the physiology of the eye, I just wanted to remind you of what happens to light as it moves through transparent substances of different densities. The light will bend or refract at the junction between the two transparent substances of different densities. So you can imagine all the structures that the light must travel through to reach the retinal layer, you can visualize the light refracting several times along the way. Slide 13 If we want to see clearly an image, we have to do something called focusing, and this is showing a simplified version of that. If we have an object that we're trying to look at, we want to take light rays from that object and get them to reach the eye. Once they reach the eye, we want to bend those light rays so that we can focus them on regions of the fovea centralis and the macula within the retina. This is how we have a nice clear image. If we have an object that's far away, the light rays are actually entering the eye in an almost parallel fashion. That's what's represented here. An image a. When they reach the cornea. The cornea, because of its curve shape, will actually bend the light rays or refract the light rays to a fairly large extent. We'll also get some refraction of light in the anterior and posterior chambers, as well the lens will have a significant amount of refraction of light. There's also some bending of the light in the vitreous humor, but most of the bending occurs in the anterior portion of the eye. Why do we want to bend the light? We actually want to converge the light rays from the top and bottom of our object so that they cross at a point known as the focal point, which is represented here by the crossed green lines. There isn't actually an image at the focal point, but this allows us to focus on a region that's just beyond the focal point. This is where the actual image appears and becomes inverted on our retina. Now our brain doesn't actually interpret our images inverted, or brain is actually able to adjust the image and interpreted as upright. Interestingly, back in the 18 hundreds they tested this year using some glasses called inversion glosses. So scientists back in the 18 hundreds actually wore these glasses that completely reversed their environment. When they put the glasses on, everything was flipped. So the floor looked like it was on the ceiling and everything that was on the ceiling looked like it was on the floor. So you can imagine this created a bit of a sensory challenge for them. And while they wore the glasses during the first four days, the images of the brain still appeared this way, so they were appearing inverted. However, after four days of wearing these inversion glasses, the images flipped. And the brain that gathered enough sensory information from their environment to readjust and interpret the images that they were seeing in the proper way. So based on other sensory information it was collecting over that period of time, it was able to readjust the image. So that's actually a pretty cool thing, that your brain is able to use all of that sensory information from your environment to interpret the visual signals that are being collected at the level of the eye. When we're looking at an image that's far away, the ciliary muscles are in a relaxed state, which means the diameter around the lens is actually quite wide. Because the ciliary muscles are relaxed, they're actually pulling on the suspensory ligaments and that creates high tension. The high tension actually flattens out the lens. So the lens actually takes on a more flattened shaped when the ciliary muscles are at rest. Or when your trying to look at an image that's far away. The majority of the light bending that's happening when we're looking far away, therefore, is happening because of the shape of the cornea. When the ciliary muscles are relaxed like this, we tend to see most clearly a point that's about 20 feet from our eyes. That point is called the far point of vision. So that's about the most clear distance we can see when our lens is in the flattened shape. If we want to focus on something that's closer than 20 feet, we actually need to bend the light rays even more than before, to get the image to appear clear. This is what's represented here in figure b. So you can imagine you're looking at something or trying to read something that's closer than 20 feet to your eye. Here the light rays are not entering the cornea in a parallel fashion. They're actually displaced a little bit or they're divergent. So in order to focus these light rays on the fovea centralis and the macular region, we actually need to bend the light rays more than we did before in order to focus them. So how do we achieve this bending of the light rays? Will we actually contract the circular ciliary muscles which decrease the diameter of this ciliary ring, so the ciliary ring gets smaller. This releases the tension on the suspensory ligaments and allows the lens to bounce back to it's more natural spherical shape. The rounded surface of the lens will then bend or refract the light more than the flatten lens does, and this allows for more focusing on the near object. The point at which the ciliary muscles are completely contracted and the lens is at its most spherical form is our near point of vision, or the nearest point that we can see. In a typical young adult that's about four to six inches away from your eye. But it gets farther and farther away as we age because the lens actually loses some of its elastic properties and becomes less and less spherical when those ligaments are slacken. This causes a loss of near vision, and you may notice this in your parents. As they start reading, those start to hold their reading materials farther and farther away from their eyes or their arms outstretched in order to see them. That's because they're lens or the shape of their lens isn't bouncing back to it's more spherical shape. So they actually have to move the object farther away to be able to focus on it. They have lost some of that near point of vision. Slide 14 So really if we want to focus an image on the retina, we have three events occurring. The first one is accommodation. This is what I've just described. The ciliary muscles contract, the lens becomes more spherical and we have greater light refraction, so therefore we get a focused object. The second his pupil constriction. The diameter of the pupil will determine what we call the depth of focus. This is the distance an object can be moved and still remain in focus. So just like when you're taking pictures, there's a person in the foreground that's in focus, the background, maybe out-of-focus or vice versa. The smaller diameter pupil, the greater the depth of focus. So it's just like the aperture of your camera. And you have a small aperture, you're able to have more things in focus. So say I'm looking at the students in a lecture theatre. If I have a small pupil diameter, I may be able to see people on the front row very clearly and people in the back row very clearly. And everyone else in between will be clear or in focus. However, if I have a large pupil diameter, the depth of focus will decrease. And I may be only able to see people in the middle rows in focus, and now the people on the front and the back rows will become blurry. This also helps explain why you squint to focus when you're having problems seeing. It's another way of reducing the amount of light that's entering your eye. In low light, the pupils dilate to let more light in, so we actually wind up losing depth of focus and low light. This is part of the reason why we don't see very well at night. The third event that needs to happen in order to focus an image on the retina is convergence. Because we have biocular vision or we have two eyes, there are actually corresponding regions on each retina that the brain detects as the same location in the visual field. So that we'll only see one object instead of two. When the objects far away, the light naturally hits the corresponding regions. But as you move our object closer and closer, we must rotate our eyes medially in order to maintain light on the same corresponding portion of the retina. If we don't move the eyes, the late would actually reach non corresponding regions of the retina. And the image will be blurred because the brain would interpreted as two objects instead of one. So let's pause here and do some more practice questions, and then we can watch a little animation that summarizes some of the things that we've talked about and highlights some of the neural pathways that we're going to talk about in the next segment. Animation No caption file Video 4 Slide 15 So how do those specialized receptor cells in the retina and take the light stimulus and turn that into an electrical impulse? We're going to start by looking at the structure of those specialized photoreceptor cells. Both the rod cells and cone cells, are bipolar cells with modified dendrite ends that are light sensitive. In rods, the light-sensitive part is cylindrical, and in cones it's conical. That's where basically where they get their names. Rod cells are used for non-colour vision and low light vision and contain a photosensitive molecule or pigment called rhodopsin. Rhodopsin is made up of a protein which spans the plasma membrane called opsin and a pigment called retinal. Retinal is located within the opsin protein. Retinal is made of vitamin A, So when your mom told you to eat your carrots because it was going to help you see more clearly, she was actually right. Carrots are filled with vitamin A. In cone cells we have a similar structure except now it's referred to as the cone photo pigments called iodopsin or photoopsin and also contains retinal, but the opsin proteins in photopsin and are slightly different. There's specialized as red, blue, or green opsin. This allows for the cones to detect color. So cone cells are used for colour vision, and they're also associated with increased visual acuity, but because of this, they require a greater amount of light or a more lightened environment. In both cells, the photosensitive molecules are located in the plasma membrane to maximize the number of these molecules. The membranes in the end of these cells closest to the pigmented layer of the retina are folded on themselves to form disk-like structures to maximize the surface area of the plasma membrane. These photosensitive molecules are also linked to as sodium gated channel, which will allow for the light stimulus to be converted into an electrical signal. The difference with the photoreceptor cells is that the sodium gated ion channel is actually open at rest or in the dark condition, which is opposite to what we've already learned when we're talking about neurons. So therefore, this cell is actually depolarized at rest. So how does this work? Slide 16 The process by which we can create the change in membrane potential with a light stimulus is a cyclic process. So we're going to start with the unstimulated condition or the dark condition, which is represented here by the purple structures. In this case, our retinal molecule is in its inactive or it's bent configuration and the opsin is in it's dark configuration, which is why it's represented in purple. The sodium channel is open and the sodium channel is actually held open by a molecule called cyclic GMP or cGMP. When this cGMP molecule is bound to the sodium ion channel, it actually keeps the gate open. So in this case, our membrane is depolarized. When we get a light stimulus, it converts the pigment retinal into its active form or a more straighten configuration. This changes our opsin molecule or opsin protein into its light configuration, and this in turn activates an enzyme known as phosphodiesterase. When phosphodiesterase becomes active, it converts cyclic GMP into GMP. This causes a decrease in the concentration of cyclic GMP, which then causes the cyclic GMP to diffuse away from the sodium ion channel and allows it to close. So therefore, no more sodium ions can move through the membrane and we actually get hyperpolarization. The active form of the retinal then detaches from the opsin protein and the phosphodiesterase becomes inactive. The cyclic GMP reforms and the concentration increases, and once again, it binds to our sodium channel and opens it, which will lead to depolarization again. At the same time, the retinal then uses some energy from ATP to return to its inactive form. It then reattaches to our opsin and returns it to its dark configuration, and that's now the cycle ready to begin again. Slide 17 So how do we use hyperpolarization in our rods and cones to produce a signal that we send to the brain? While I keep showing the rhodopsin here in these examples, it will be the same situation that's happening with our photopsin and in our cone cells as well. In order to send a signal when we have a light stimulus, we use those other cells in the neural layer of the retina that we've already discussed to create action potentials that reach the optic nerve and travel to the brain; and this is how it works. So on the graph you can see that the membrane is depolarized at rest or in the dark condition. Our rod cell is unstimulated and our rhodopsin is in its dark configuration, and also the sodium channel is open. Because the cell is depolarized in this unstimulated state, it's constantly releasing neurotransmitter. The neurotransmitter in this case is glutamate. This is detected by the bipolar cell, which is the postsynaptic membrane in this instance. The difference here is that the glutamate causes an IPSP or an inhibitory postsynaptic potential. So the neurotransmitter is causing hyperpolarization in the bipolar cell. So no action potential is produced and the bipolar cell and the signal is not being transmitted to the optic nerve. With a light stimulus, the sodium channels close. The rhodopsin converts to it's light configuration, as we can see here, and this causes the hyperpolarization, which we can see on the slide. So the rod cells become hyperpolarized. With hyperpolarization, We have no action potentials in our rod cells. Therefore, the neurotransmitter that gets released from our rod cell actually decreases, and so therefore our neurotransmitter is not binding to the ligand-gated channels on our bipolar cell. This allows our bipolar cell to be no longer inhibited and it actually depolarizes. We get an action potential that results from the lack of neurotransmitter binding to our bipolar cell. The bipolar cells action potential then releases neurotransmitters towards the postsynaptic membrane of the ganglion cell. And the ganglion cells can then create an action potential which will move on to our optic nerve. So if we're looking here in the dark, we're seeing our signal moving through the rods and cones cells. That inhibits the bipolar cells and stops the signal. Or with a light situation, we stop the action potentials and our rod and cone cells from occurring, this releases the inhibition of the bipolar cells, which activates an action potential stimulating our ganglion cells, which will then send the action potential through the optic nerve. Slide 18 Finally, once we create an action potential in the optic nerve, we want to know where that signal goes or what's the neural pathway. Each eye has a visual field which is separated into the temporal half of the visual field, which is the side closest to your ears, and the nasal visual fields, which are the visual fields closest to your nose. Nasal visual fields actually project onto the temporal side of the retina, and the temporal visual fields project onto the nasal side of the retina. So they actually converge or cross over inside the eye. The neurons from the retina then converge into the optic nerves, which we know from our previous lecture, is cranial nerve number two. The optic nerves then converge into a region known as the optic chiasm. This is an interesting area because from here, the visual fields on the right side. So the temporal visual field of the right eye and the nasal visual field of the left eye, travel to the left side of the brain and vice versa for the left visual fields. So you can see here that the right visual field for the right eye is the temporal half, and the right visual field for the left eye is the nasal half. So these two are actually moving to the left side of the retina, as well as the left side of the brain. Where as the left visual fields will wind up on the right sides of the brain. After the optic chiasm, they form the optic tract in some of the neurons will go to the superior colliculi of the midbrain, where they're involved in the visual reflexes. The rest of the neurons will continue on to the lateral geniculate nuclei of the thalamus that we talked about earlier in lectures. They synapse here and then travel to the occipital lobe as the optic radiations, where they reach the primary visual cortex for interpretation in the occipital lobe. Conclusion So that's what we're going to end today's online lecture. Today we began by looking at the accessory structures for the eye like the lacrimal apparatus and the extrinsic muscles of the eye. And then we started to look more deeply at the structure of the eye itself and the various tunics that make up the eye. Then we focused primarily on the retina region of the eye and how we can focus light from our external environment on to that region of the retina. Then we talked about how we actually turn a light signal into an electrical impulse that can be sent to the brain, and the neural pathways that carried that signal to the different regions of the brain. So in our next online lecture, we're going to finish off the special senses by talking about hearing and balance. So until then, take care.