Manufacturing of Composites - MECH 415/6521 PDF
Document Details
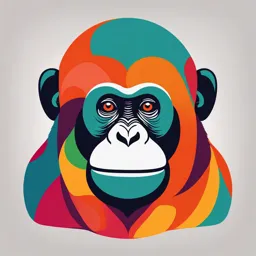
Uploaded by IndulgentBamboo9863
Concordia University
Suong V. Hoa
Tags
Summary
This document provides an overview of the manufacturing of composite materials, focusing on different types of matrix materials, polymer matrix materials, thermoset matrix polymers, and more. Specific examples and tables offer insights into materials and their properties. The document is likely a part of a larger course on composite materials or for similar research purposes.
Full Transcript
Manufacturing of composites MECH 415/6521 Suong V. Hoa Concordia Center for Composites Mechanical and Industrial Engineering Concordia University Matrix Different types of matrix materials Polymer matrix composites – Carbon/epoxy – Glass/epoxy...
Manufacturing of composites MECH 415/6521 Suong V. Hoa Concordia Center for Composites Mechanical and Industrial Engineering Concordia University Matrix Different types of matrix materials Polymer matrix composites – Carbon/epoxy – Glass/epoxy – Glass/polyester – Kevlar /epoxy Metal matrix composites – Silicon carbide/aluminum – Carbon fiber/aluminum Ceramic matrix composites – Carbon/carbon – Carbon/alumina There are more polymer matrix composites as compared to other types of composites due to the Compatibility condition. Different types of polymer matrix materials Thermoset matrix composites – Carbon/epoxy – Glass/epoxy – Glass/polyester – Kevlar /epoxy Thermoplastic matrix composites – Carbon/PEEK – Carbon/PPS – Glass/nylon There are more thermoset matrix composites as compared to thermoplastic matrix composites due to the Availability condition. Material 20oC 25 oC ToC Air 0.0187 Water 1 Polyester 100-300 Vinyl ester 100-300 #10 Motor oil 500 Golden syrup 2,500 Epoxy (Shell Epon 828-14 600 phrMPDA, 15 phr BGE) Epoxy (Shell 826 – 16 phr 750 MPDA, 10phr BGE) Epoxy (Dow 332-16 phr MPDA, 500 10 phr BGE) Molasses 105 Epoxy 5208 100@177oC BMI 1000@150 oC Ryton (thermoplastic) 107 @313oC PEEK (thermoplastic) 106@400 oC Utem (thermoplastic) 108@305 oC Torlon (thermoplastic) 109@350 oC Table 2.1: Viscosity of a few thermoset and thermoplastic materials (in centipoise) 1 Pa-sec = 10 Poise = 1000 centi-Poise (a) (b) (c) (d) (e) Figure 2.1 : Schematic of (a) the molecules in a thermoset resin, (b) the linking molecules, (c) the resin molecules and the linking molecules in a container before linking reactions, (d) the thermoset resin network after linking reactions, and (e) a partially linked network. Thermoset matrix polymers Thermoset matrix composites – Carbon/epoxy – Glass/epoxy – Glass/polyester – Kevlar /epoxy Thermoplastic matrix composites – Carbon/PEEK – Carbon/PPS – Glass/nylon There are more thermoset matrix composites as compared to thermoplastic matrix composites due to the Availability condition. Figure 2.2: Schematic of the molecules in a thermoplastic resin. Polyester production Example 2.1: It is desired to make a polyester resin using 100 g of maleic acid and a corresponding amount of ethylene glycol. A stoichiometric amount of ethylene glycol is used. After the condensate is removed, how many grams of polyester are obtained? Mass of different atoms: C = 12 g/mol, H = 1 g/mol, O = 16 g/mol, N = 14g/mol REPEATING UNIT Maleic acid: 4 C + 4 H + 4 O = 4 ( 12 + 1 + 16) =116 g/mole Ethylene glycol: 2 C + 6 H + 2 O = 24 + 6 + 32 = 62 g/mole The reaction takes place as shown in the following: It can be seen that one molecule of maleic acid reacts with one molecule of ethylene glycol to make a unit of ester and two water molecules. Mass of the water molecule: 2 H + O = 18 g/mole. Let Mp be the mass of the polyester made using 100 g of maleic acid, we have: (Mp/100) = (116 + 62 - 36)/116 = 1.224. Mp = 122.4 g. Initiators Name of peroxide Chemical structure Hydrogen peroxide H-OO-H Hydroperoxides R-OO-H Dialkyl peroxides R-OO-R Diacyl peroxides R-C(O)-OO-C(O)-R Peroxyesters R-C(O)-OO-R Peroxy Acids R-C(O)-OO-H Peroxy Ketals R2-C-OO-R2 Peroxy Dicarbonates R-OC(O)-OO-C(O)O-R Table 2.2: Structures of commercial organic peroxides Polyester cross linking Example 2.2: Example on cross linking of polyester: It is desired to make a polyester using 100 g of maleic acid and ethylene glycol. A stoichiometric amount of ethylene glycol is used. Cross linking is done using styrene. Assume that one styrene monomer corresponds to one oligoester (this assumption is to simplify the calculation to illustrate the principle; in reality the crosslink between oligoester molecules can be in the range from 1 to 4 styrene monomers). From the cross linking process, how many C=C bonds are broken, how many C- C bonds are formed? Solution: Continuing from the same problem in Example 2.1. Number of bonds broken and formed: Therefore for each polyester unit, there are 2 C=C bonds broken and 4 C-C bonds formed. The chemical formula for a polyester unit is: O O The mass of a polyester unit is: C CH CH C O CH2 CH2 O n 6 C + 6 H + 4 O = 72 + 6 + 64 = 142 g/mole. Since there are 122.4 g of polyester made, the number of bonds involved is: C=C bonds: (122.4/142) 2 = 1.724 mole or 1.038 x 1024 bonds. (note that 1 mole = 0.602 x 10 24). C-C bonds: 2 x 1.724 = 3.446 moles or 2.076 x 1024 bonds. Atomic bond energy Bond Energy (kJ/mole) C-C 370 C=C 680 C-H 435 O-H 500 N-H 430 C-O 360 C=O 535 Example 2.3: Example on heat generation and temperature increase: It is desired to make a polyester using 100 g of maleic acid and ethylene glycol. A stoichiometric amount of ethylene glycol is used. Cross linking is done using styrene. Continuing from the same problem in Examples 2.1 and 2.2. The energy required to break a C=C bond is 680 kJ/mole and the energy created by the forming a C-C bond is 370 kJ/mole. How much energy is generated during the polymerization process? 1 mole = 0.602 x 1024. The heat capacity of polyester is 0.25 cal/g/oC. Assuming no heat loss, what is the increase in temperature of the polyester? Mass of carbon C= 12 g/mole, H = 1 g/mole, O = 16 g/mole Solution: a) Energy generated: Considering the energy in the bonds, one has: Energy inputted into the system to break the double bonds: (1.724mole) (680 kJ/mole) = 1172.32 kJ. Energy generated from the system to form the single bonds: (3.446 moles)(370 kJ/mole) = 1275.02 kJ. Net energy generated: 1275.2- 1172.32 = 102.7 kJ. b) Temperature increase: Heat stored in the material: Q = m c ∆T or ∆T = Q/(mc) For the mass of the material, apart from the polyester, there is also the styrene. Each unit of the polyester is corresponding to each unit of the styrene for complete crosslinking. The chemical formula for styrene is shown in the following: The mass of a styrene molecule is therefore: 8 C + 8 H = 96 + 8 = 104 g/mole. Different types of reactants O HO CH2 CH2 OH Ethylene glycol Orthophthalic acid C OH HO CH CH2 OH (Ortho) C OH Propylene glycol CH3 O O O O Orthophthalic C Maleic acid (also HO C CH CH C OH anhydride O Fumeric acid) C O CH CH C O Maleic anhydride O C O O C OH Isophthalic acid (Iso) C O OH Polyester use and Storage Container for shipping and storage Shelf life Pot life Inhibitors Accelerators Prepregs Coupling agents Fillers Epoxy CH CH CH CH CH2 CH3 GROUPS O O C CH2 CH CH2 Cl + H O OH Epoxy Group Glycidyl O CH3 REACTANTS Epichlorohydrin Bisphenol-A CH3 CH2 CH CH2 O C O H + Cl CH2 CH CH2 O O CH3 1. Most commonly used resin for advanced composites 2. Good adhesive strength 3. Low shrinkage 4. Operating temperature up to 140 oC Diglycidyl ether of bisphenol A (DGEBPA) PRODUCT CH3 CH2 CH CH2 O C O CH2 CH CH2 O CH3 O Formation of epoxy resin CH3 CH2 CH CH2 Cl + H O C OH O Epichlorohydrin Bisphenol-A CH3 Add another Epichlorohydrin CH3 CH2 CH CH2Cl Epichlorohydrin CH2 CH CH2 O C O H O O CH3 CH3 CH3 CH CH2 O CH2 CH CH2 O C CH2 CH CH2 Add another Epichlorohydrin CH2 C O O O O CH3 OH CH3 CH2 CH CH2 Cl Epichlorohydrin O CH3 CH2 CH CH2 O C O CH2 CH CH2 O O CH3 Add another Bisphenol A CH3 HO C OH Bisphenol-A CH3 CH3 CH3 CH2 CH CH2 O C O CH2 CH CH2 O C OH O OH CH3 CH3 Specialty epoxy resins CH3 CH3 CH2 CH CH2 O C O CH2 CH CH2 O C O CH2 CH CH2 O n O CH3 OH CH3 Diglyci dyl Ether of Bisphenol A (DGEBPA) CH2 CH2 CH2 O O O CH CH CH CH2 CH2 CH2 O O O CH2 n CH2 Epoxy Novolac (Epoxidized Phenolic Resin) Example: DOW DEN 438 H CH2 CH CH2 O C O CH2 CH CH2 O O O O CH2 CH2 CH CH2 CH2 CH CH2 CH CH2 O C O CH2 CH CH2 O N CH2 N O O CH2 CH CH2 CH2 CH CH2 H O Tetraglycidylether of Tetrakis (Hydroxyphenyl) Ether Tetraglycidylmethylene Dianiline (TGMDA) Example: SHELL EPON 103 Example: CIBA MY-720 Diluents 1. To reduce the viscosity of the resin 2. To improve shelf or pot life 3. To lower the exotherm 4. Diluents may react with the resin and become an integral part of the cured resin system 5. Butyl glycidyl ether, Cresyl glycidyl ether, Phenyl glycidyl ether. Curing systems for epoxies Amines Anhydrides Tertiary amines and accelerators Amine curing agents AMINE HYDROGEN VISCOSITY @ 25°C AMINE CURING AGENTS EQUIVALENT WEIGHT (77°F), Pa.sec (cP) (g/eq) 0.0055-0.0085 Diethylenetriamine (DETA) 20 (5.5-8.5) H2N CH2 CH2 NH CH2 CH2 NH2 0.020-0.023 Triethylenetetramine (TETA) 24 (20-23) H2N ((CH2)2 NH)2 CH2 CH2 NH2 Diethylaminepropylamine 65 < 5.0 (DEAPA) CH3 CH2 N CH2 NH2 3 CH3 CH2 Amine curing agents OH NH2 NH2 + CH2 CH NH2 NH CH2 CH O OH OH NH2 N CH2 CH NH2 NH CH2 CH + CH2 CH O CH2 CH OH Stoichiometric ratio Molecular weight of amine Parts by weight of amine to be Number of available amine hydrogens per molecule used with 100 parts by weight = X 100 Epoxy equivalent weight of epoxy resin (phr) Epoxy equivalent weight = (Molecular weight of epoxy molecule)/Number of epoxy groups in the molecule) Amine hydrogen: Hydrogen atom attached to Nitrogen Amine hydrogen equivalent weight = (Molecular weight of amine molecule)/(Number of amine hydrogen atoms in the amine molecule) Anhydride curing agent O C Phthalic anhydride (PA) O C O O O R R CH C R R CH... C N + R O. R CH2 CH CH2 O + R N R R CH C R R CH C O O O O R R CH C... N + R R R CH C O CH2 CH2 CH R O O Tertiary amines and accelerators N C CH3 C CH Boron Trifluoride Methyl CH3 CH2 N H EMI accelerator Amine (BF3-MEA) F F B NH2 CH2 CH3 F Homopolymerization Etherification Nature of the reactions and heat generated Primary amine: RNH2 Secondary amine: RNH Tertiary amine: RN Energy associated with primary amine: 83 kJ/mole Energy associated with secondary amine: 131 kJ/mole Energy associated with tertiary amine: higher Example: 1. Assume that there are 100 primary amines to begin with. 2. In the first stage, 20 primary amines participate into the reaction. Heat comes out and raises the temperature of the material. 3. When the temperature is high enough, the 20 secondary amines can participate into the reaction, along with the remaining 80 primary amines. 4. As the temperature rises more, some of the tertiary amines may also participate into the reaction. From then on, at any instant of time, there can be three types of amines that participate into the reaction, giving rise to more heat coming out. 5. When most of the amines are consumed, there are less and less amines remaining for the reaction, heat generated becomes less and less. 6. The heat generated is there complex. 7. The rate of cure is also complex. Example on relative concentration Question: It is desired to cross link a DGEBPA epoxy resin using an amine curing agent called DETA. The formula for the two materials are as shown in Figure 2.8 and Table 2.5. How many grams of DETA should be used if 100g of the epoxy resin are used? Solution: One repeat unit of the DGEBPA epoxy is shown at the bottom of Figure 2.7. Note that the symbol H H Diethylenetriamine (DETA) C C H2N CH2 CH2 NH CH2 CH2 NH2 means C C EPOXY PRODUCT C C CH3 CH2 CH CH2 O C O CH2 CH CH2 H H O CH3 O Counting the number of atoms, each unit of DGEBPA has 21 carbon atoms, 24 Hydrogen atoms, and 4 oxygen atoms. The mass of the epoxy unit is therefore: m1 = 21 (12) + 24 (1) + 4 (16) = 340 g/mole The DETA molecule (Table 2.5) has 4 carbon atoms, 3 Nitrogen atoms and 13 Hydrogen atoms. The mass of DETA is: m2 = 4 (12) + 3 (14) + 13 (1) =103 g/mole. In the epoxy molecule, there are two epoxy groups, the epoxy equivalent weight is therefore: m3 = 340/2 = 170 g/mole. There are 5 available hydrogens in the amine molecule. The value of molecular weight of amine over the number of available hydrogens per molecule is: m4 = 103/5 = 20.6 g/mole Parts by weight of amine to be used with 100 parts by weight of epoxy resin are: Relative concentration 1. Large excess of epoxy 2. One reactive epoxy site for one reactive hardener site 3. One epoxy molecule for one hardener molecule 4. High excess of hardener Cured epoxy resin systems 1. Aromatic rings 2. Cross link density 1. Number of cross links per volume of material 2. Lower cross link density improves toughness 3. Lower cross link density reduces shrinkage 4. Higher cross link density improves resistance to chemical attack 5. Higher cross link density increases heat distortion temperature Pot life Prepregs- Shelf life Quality control At the liquid stage: Measure viscosity At the solid stage: degree of cure Chemical principle FTIR Acetone wipe Quality control At the solid stage: degree of cure Electrical principle Quality control At the solid stage: degree of cure Mechanical principle Barcol hardness test DMA Ultrasonic Shrinkage measurement Quality control At the solid stage: degree of cure Thermal principle -DSC test Cycle 2 Cycle 3 Degree of cure and rate of cure Degree of cure α: 0< α < 1 dα Rate of cure dt dα = f (α , T ) dt dα  ∆E  m = A exp − α (1 − α ) n dt  RT  Vinyl ester resin O R R' O C C C Vinyl ester resin O R' Heat O O catalyst C C C R C C C + 2HO C C C Resin formation R' O OH OH O R' C C C O C C C R C C C O C C C + coreactant + inhibitors C where R = O C O and R' = H or -CH3 C Addition of ethylenically unsaturated carboxylic acid molecules and epoxy molecules Properties: between epoxy and polyester Cost: between epoxy and polyester Vinyl ester resin R' O OH OH O R' C C C O C C C R C C C O C C C + R'' C C * * * * * R (Free- Radical initiator) OH O R' Curing R C C C O C C C R'' C C C where R = O C O C R' = H or -CH3 R'' = * = Reactive sites Polyimide O C N C O Temperature stability up to 350oC Epoxies stable up to 177oC Bismalimide (BMI) o Cured at 177 C o Post cured at 246 C to obtain full cure and properties significantly higher than epoxies Phenolic matrix High temperature applications Made by reaction between phenol & formaldehyde Brittle and high shrinkage Fillers are used to reduce brittleness Used for electrical switches, auto molded parts, billiard balls Used as liner for rocket nozzles. Material ablate at high temperature. Carbon matrix Made from carbon fibers reinforced phenolics Pyrolysis (charring) Porous material is re-impregnated with pitch, phenolics or carbon by vapor deposition Process may take up to 6 months. Higher temperature applications than phenolic Liners for rocket nozzles Tiles for space shuttle nose cones Aircraft, race car and truck brakes Carbon/carbon composites have the highests energy absorption (specific heat) than any known materials Thermoplastic matrix No shelf life Short processing cycle Higher ductility than thermosets Repairable Recyclable Higher viscosity than thermosets Requires high temperature for processing Tapes are stiff and boardy Two types of thermoplastic resins o Industrial thermoplastics (up to about 80 C) Polyethylene (PE) Polyvinyl chloride (PVC) Polymethymethacrylate (PMMA) Polypropylene (PP) Polystyrene (PS) Acrylonitrile Butadiene Styrene (ABS) o High performance thermoplastics (up to ~ 300 C) Poly-ether-ether-ketone (PEEK) Poly-ether-ketone-ketone (PEKK) Poly-ether-imide (PEI) Poly-phenylene sulfone (PPS) Poly sulfone Thermoplastic matrix Thermoplastic matrix Thermoplastic matrix-Fabrication Difficult due to high viscosity- 106 centi-poise as compared to 1000 centi-poise for liquid epoxy Shear thinning Comingled fibers Power impregnation Fillers Cost reduction Shrinkage reduction Improvement of mechanical properties Improvement of flame resistance Colorants, pigments Metal matrix Aluminum, titanium, magnesium, copper Short fibers such as SiC- particles, whiskers, fibers Piston ring inserts, pistons, connecting rods High temperature applications Difficult to make Ceramic matrix Oxides, carbides, borides, nitrides Short fibers such as SiC- particles, whiskers Made by chemical vapor deposition High temperature applications Difficult to make Polymer nanocomposites a = 1, R = 6 a = 0.5, R = 12 a = 0.1, R = 60 R = aspect ratio Structure of clay sheets Clay sheets with intercalating ions Different levels of clay structure Replacing positive ions with long omium ions Dispersion techniques High pressure mixing technique X Ray pattern for samples mixed using different methods Minimum distance 0.3 microns, Maximum distance 3.2 microns, Mean distance 0.9 microns Distribution of sized of particles in epoxy samples mixed using High Pressure method- 2 wt% I 30E in TGDDM-DDS TEM of samples mixed using high pressure mixing method- Clay particle containing about 6 clay sheets 800 DMM HPM-A 600 G1C , J/m2 400 200 0 0 3 6 9 12 Clay Loading, phr Variation of strain energy release rate as a function of percent clays- Max increase: 5.8 times compared with no clay. (b) (a) (c) High speed mixing (10,000 rpm) 5000 8.24 nm 3.74 nm 4000 3.84 nm Intensity (counts) 3000 1.85 nm 2000 C30B ENC-D230-6wt%C30B 1000 ENC-D2000-6wt%C30B EPON828-C30B 0 2 4 6 8 10 o 2θ ( ) X Ray Diffraction pattern for samples mixed using high speeds. Strain energy release rates of epoxy/clay samples mixed using high speed method. Procedure to incorporate modified epoxy into long continuous carbon fibers. a) Hand Lay Up carbon fibers with epoxy/clay. b) Bagging. c) Autoclave cure. d) Final sample. 450 0phr 400 350 2phr 300 4phr GIc, J/m 2 250 200 150 100 50 0 40 50 60 70 80 90 100 a, mm Improvement in fracture toughness of composite samples 1 region I QISF region II Direction of 0.8 QISU Normalized stiffness, E/E0 I crack growth 0.6 II region III 0.4 III 0.2 0 0 20000 40000 60000 80000 100000 120000 Number of cycles,N Improvement of fatigue lives of tapered composite sample (glass /epoxy) subjected to tensile-tensile fatigue loading. Broken curve for unfilled epoxy. Solid curve for epoxy with nanoclay. Possible applications 1. Barrier properties: obstruction to diffusion of small particles: CO2, Flammability resistance. 2. Fracture resistance Carbon nanotube configurations Three-roll milling machine 102 101 100 Conductivity, S/m 10-1 Ref. in Ref. in , 10-2 10-3 10-4 NLIG NIKSW 10-5 0 1 2 3 4 5 6 7 8 9 CNT weight fraction, % Variation of electrical conductivity with amount of nanotubes Possible applications 1. Enhance electrical conductivity: Lightning strike resistance, conductive adhesives. 2. Uses for strain measurement. 3. Uses to detect defect occurrence in composite structures. Aggregately conductive materials Electrical resistance between two points in a plate. a) copper plate, b) glass/epoxy containing CNTs. R R ∆R 1  R R   1  Rtotal = Rtotal = =  − = N N −1 R R  N N − 1  N ( N − 1)  Hole 1 (1/16 inch) Hole 3 (3/16 inch) Hole 5 (5/16 inch) Hole 6 (6/16 inch) Hole 4 (4/16inch) Hole 2 (2/16 inch) 22×13 inch2 carbon fiber/epoxy/ composite plate Change in electrical resistance due to impact by projectile on carbon/epoxy with CNTs Thank you