Vascular Physiology Lecture 4 PDF
Document Details
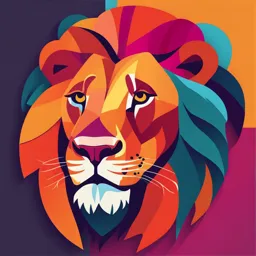
Uploaded by ArticulateSerpent9095
Al-Balqa' Applied University (BAU)
Dr. Asem Alkhalaileh
Tags
Summary
This document is a lecture on vascular physiology, specifically focusing on the mechanisms of capillary exchange, interstitium, and interstitial fluid along with Starling forces. It appears to be a study resource and not an examination paper.
Full Transcript
Vascular physiology Lecture 4 By Dr. Asem Alkhalaileh Overview: Concept 1: Mechanism of capillary exchange Concept 2: Interstitium and interstitial fluid Concept 3 : Starling forces Concept 1: Mechanism of capillary exchange Mechanisms of Capillary Exchange: Microvascular...
Vascular physiology Lecture 4 By Dr. Asem Alkhalaileh Overview: Concept 1: Mechanism of capillary exchange Concept 2: Interstitium and interstitial fluid Concept 3 : Starling forces Concept 1: Mechanism of capillary exchange Mechanisms of Capillary Exchange: Microvascular circulation The microcirculation refers to the highly-distribute smallest blood vessels in the body: smallest arterioles, meta-arterioles, pre-capillary sphincters, the capillaries and the small venules. In addition to these blood vessels, some might also include lymphatic capillaries and even collecting duct. The basic functions of the microcirculation are to provide a source of nutrients and fluid for tissues and carry away metabolic wastes. These exchange processes are design to be selective, thus preventing escape of critical blood elements into the interstitial fluid. Much of these functions are dependent on the unique histological architecture of the microcirculation. Mechanisms of Capillary Exchange: Diffusion Several mechanisms are involved in capillary exchange including: Diffusion, Filtration and reabsorption and Transcytosis (Vesicular Transport) Diffusion is dependent on (Fick’s Law of Diffusion) so all the factors affecting the Fick equation can play a role such as: the length of capillary (area), the thickness of capillary wall and the concentration difference (from higher concentration to lower concentration) on two sides Diffusion is bidirectional along the whole length of the capillary (as movement depend on concentration difference Diffusion does not result in net water movement across the capillary wall, because no concentration difference, this is why (Starling forces) are not involved in diffusion. Mechanisms of Capillary Exchange: Filtration and reabsorption Filtration is considered to occur because of the imbalance of (Starling forces) in pressure (from higher pressure to lower pressure) on two sides Filtration results in net movement of water Filtration is an imbalance between 1. forces promoting outward (Filtration: out of capillary to tissues) 2. forces promoting inward (reabsorption: into capillary from tissues) Mechanisms of Capillary Exchange: Transcytosis (Vesicular transport) Vesicular transport is involved in the translocation of macromolecules across capillary endothelium by endocytosis and exocytosis. Transcytosis, blood substances move into the cell by endocytosis then across the endothelial cells that compose the capillary structure. Finally, these materials exit by exocytosis, process in which vesicles go out from a cell to the interstitial space. Large molecules that are lipid-insoluble, such as the insulin hormone, mainly use Transcytosis. Minimum amounts of substances cross by transcytosis. Once vesicles exit the capillaries, they go to the interstitium, sometimes vesicles can merge يندمجwith other vesicles, so their contents are mixed, or can directly go to a specific tissue. go to a specific tissue. This material intermixed increases the functional capability of the VESICLE. Sometimes versicles fuse with each other, forming vascular channels Mechanisms of Capillary Exchange Mechanisms of Capillary Exchange: Transcytosis (Vesicular transport) Present in the endothelial cells are many minute plasma- lemmal vesicles, also The diameter of the capillaries is what determines Mechanisms of Capillary Exchange: what substances pass through the cleft Effect of molecular size and type Small, non-polar lipid soluble molecules can easily diffuse through endothelial cells and thus directly across the capillary barrier. This is especially true of gases such as O2 and CO2 as well as non-polar small molecules such as steroid hormones. Small, polar, water-soluble molecules can diffuse through intercellular clefts between the endothelial cells as seen in continuous capillaries. Many substances needed by the tissues are soluble in water but cannot pass through the lipid membranes of the endothelial cells; such substances include water molecules, sodium ions, chloride ions, and glucose Large, water-soluble molecules can diffuse through Intercellular “Pores” in the Fenestrated Capillary Membrane (capillaries). Fenestrated capillaries (fenstra: window) have pores in the endothelial cells (60-80 nm in diameter).Pores spanned امتدادby a diaphragm of radially شعاعيoriented fibers pores allow small molecules and limited amounts of protein to diffuse Mechanisms of Capillary Exchange: Intercellular clefts Although only 1/1000 of the surface area of the capillaries is represented by the intercellular clefts between the endothelial cells, the velocity of molecular motion in the clefts is so great that even this small area is sufficient to allow tremendous diffusion of water and water-soluble substances through these cleft-pores. To give one an idea of the rapidity with which these substances diffuse, the rate at which water molecules diffuse through the capillary membrane is about 80 times as great as the rate at which plasma itself flows linearly along the capillary. That is, the water of the plasma is exchanged with the water of the interstitial fluid 80 times before the plasma can flow the entire distance through the capillary. Mechanisms of Capillary Exchange: Intercellular clefts HENECE some substances like the proteins are water soluble, but too large to enter The width of the capillary intercellular cleft-pores, 6 to 7 nanometers, is about 20 times the diameter of the water molecule, which is the smallest molecule that normally passes through the capillary pores. The diameters of plasma protein molecules, however, are slightly greater than the width of the pores. Other substances, such as sodium ions, chloride ions, glucose, and urea, have inter- mediate diameters. Therefore, the permeability of the capillary pores for different substances varies according to their molecular diameters. The permeability for glucose molecules is 0.6 times that for water molecules, The permeability for albumin molecules is very slight—only 1/1000 that for water molecules. Concept 2: Interstitium and interstitial fluid Interstitium and interstitial fluid About 1/6 of the total volume of the body consists of spaces between cells, which collectively are called the interstitium. The fluid in these spaces is called the interstitial fluid. The structure of the interstitium contains two major types of solid structures: 1. Collagen fiber bundles The collagen fiber bundles extend long distances in the interstitium and are extremely strong and therefore provide most of the tensional strength of the tissues. 2. Proteoglycan filaments. The proteoglycan filaments, however, are extremely thin coiled or twisted molecules and composed of about 98% hyaluronic acid and 2% protein. The proteoglycan filaments are so thin that they cannot be seen with a light microscope, and they are difficult to demonstrate even with the electron microscope. The proteoglycan filaments form a mat of very fine reticular filaments aptly described as a “brush pile”. Interstitium and interstitial fluid Gel in the interstitium The fluid in the interstitium is derived by filtration and diffusion from the capillaries. The fluid in the interstitium contains almost the same constituents as plasma except for much lower concentrations of proteins because proteins do not easily pass outward through the pores of the capillaries. The fluid in the interstitium is entrapped mainly in the minute spaces among the proteoglycan filaments. This combination of proteoglycan filaments and fluid entrapped within them has the characteristics of a gel and therefore is called tissue gel. BULK flow Interstitium and interstitial fluid Free fluid in the interstitium Although almost all the fluid in the interstitium normally is entrapped within the tissue gel, occasionally small rivulets انهار صغيرةof “free” fluid and small free fluid vesicles are also present, which means fluid that is free of the proteoglycan molecules and therefore can flow freely. When a dye is injected into the circulating blood, it often can be seen to flow through the interstitium in the small rivulets, usually coursing along the surfaces of collagen fibers or surfaces of cells. The amount of “free” fluid present in normal tissues is slight—usually less than 1 percent. Conversely, when the tissues develop edema, these small pockets and rivulets of free fluid expand tremendously until one half or more of the edema fluid becomes freely flowing fluid independent of the proteoglycan filaments. Interstitium and interstitial fluid Importance of the proteoglycan filaments The proteoglycan filaments have many functions: “Spacer” for the cells Prevents rapid flow of fluid in the tissues 1) “Spacer” for the cells The proteoglycan filaments, along with much larger collagen fibrils in the interstitial spaces, act as a “spacer” between the cells. Nutrients and ions do not diffuse readily through cell membranes without adequate spacing between the cells, so that these nutrients, electrolytes, and cell waste products could be rapidly exchanged between the blood capillaries and cells located at a distance from one another. Interstitium and interstitial fluid Importance of the proteoglycan filaments 2) Prevents rapid flow of fluid in the tissues A. The interstitial fluid is difficult to flow easily through the tissue gel because of the large number of proteoglycan filaments. B. The interstitial fluid mainly diffuses through the gel. Diffusion through the gel occurs about 95 to 99 percent as rapidly as it does through free fluid. For the short distances between the capillaries and the tissue cells, this diffusion allows rapid transport through the interstitium not only of water molecules but also of electrolytes, small molecular weight nutrients, cellular excreta, oxygen, carbon dioxide, and so forth. Interstitium and interstitial fluid Importance of the proteoglycan filaments C. The interstitial fluid molecules move molecule by molecule from one place to another by kinetic, thermal motion rather than by large numbers of molecules moving together. D. If it were not for the proteoglycan filaments, the simple act of a person standing up would cause large amounts of interstitial fluid to flow from the upper body to the lower body. When too much fluid accumulates in the interstitium, as occurs in edema, this extra fluid creates large channels that allow the fluid to flow readily through the interstitium. Therefore, when severe edema occurs in the legs, the edema fluid often can be decreased by simply elevating the legs. Interstitium and interstitial fluid What controls the movements of fluids? Selective perfusion of capillaries Not all capillaries are perfused with blood at all times. Rather, there is a selective perfusion of capillary beds (Vaso-motion) depending on the metabolic needs of the tissues. Selective perfusion of capillary beds is determined by the degree of dilation or constriction of the arterioles and precapillary sphincters. The degree of dilation or constriction of the arterioles and precapillary sphincters is controlled by: 1)The sympathetic innervation of vascular smooth muscle and 2) Vasoactive metabolites produced in the tissues. Interstitium and interstitial fluid Bulk flow (Convection) The mass movement of fluids into and out of capillary beds requires a transport mechanism far more efficient than diffusion. This movement, often referred to as bulk flow, involves two pressure-driven mechanisms: Volumes of fluid move from an area of higher pressure in a capillary bed to an area of lower pressure in the tissues via filtration. In contrast, the movement of fluid from an area of higher pressure in the tissues into an area of lower pressure in the capillaries is reabsorption. Interstitium and interstitial fluid Bulk flow (Convection) Two types of pressure interact to drive each of these movements: hydrostatic pressure and osmotic pressure. Bulk flow of fluid and electrolytes occurs through "pores" and intercellular clefts. Bulk flow mechanism of exchange is particularly important in renal glomerular capillaries; however, it occurs to variable extent in nearly all tissues. Concept 3: Starling forces ❑ Starling Forces. Starling Forces govern the passive exchange of water between the capillary microcirculation and the interstitial fluid. These forces not only determine the directionality of net water movement between two different compartments but also determines the rate at which water exchange occurs. Starling forces maintains the balance between filtration and re-absorption of H2O depends both on the difference in hydrostatic and oncotic pressure between the blood and the tissue and on the permeability of the vessel. Qw = K. [(Pc – Pi) –σ (πc – πi)] where Qw : bulk flow of water , K: permeability-surface area coefficient, Pc: capillary pressure, Pi: interstitial fluid pressure, σ: reflection coefficient, πc: capillary(or plasma) colloid osmotic pressure, πi: interstitial fluid colloid osmotic ❑ The permeability-surface area coefficient (K) Sometimes called capillary filtration coefficient Means : how many ml of fluid is filtered in the unit of time (min) , through the unit mass of membrane (g) when the pressure difference is one unit (mmHg) ? So its unit is ml/min/mm Hg/100 g Depends on two factors : 1. surface area.. the length and diameter of the vessel, and the number of capillaries 2. Permeability.. the number and size and thickness of the pores The capillary filtration coefficient of the average tissue is about 0.01 ml/min/mm Hg/100 g However because of extreme differences in permeability of the capillary systems in different tissues, this coefficient varies more than 100-fold among the different tissues Examples 1. very small in brain and muscle, 2. moderately large in subcutaneous tissue, 3. large in the intestine, 4. extremely large in the liver and glomerulus of the kidney We also can study protein permeation, since the permeation of proteins through the capillary membranes varies greatly as well The concentration expressed as g of protein /dl of the interstitial fluid, this means : how many gram of proteins does permeate with the filtrated fluid ? Examples 1. In brain is close to zero, 2. In muscles is about, 1.5 g/dl 3. In subcutaneous tissue, 2 g/dl 4. In intestine 4 g/dl 5. In liver, 6 g/dl Fenestrated capillaries have a higher filtration of potassium than continuous capillaries. Substances such as histamine, increase potassium filtration ❑ The reflection coefficient (σ: sigma) Is the inverse of the permeability of the vessel wall to protein and is a measure of the degree to which molecules retained or reflected by the membrane So that, a molecule in the blood that reflects from the capillary wall and does not cross the capillary wall into the interstitium has permeability coefficient of zero, but a reflection coefficient of 1 and the osmotic pressure is fully exerted. And, a molecule in the blood that cross the capillary wall into the interstitium and does not reflects from the capillary wall has permeability coefficient of 1, but a reflection coefficient of 0 and no osmotic pressure is exerted. That’s why the continuous capillaries have a high σ (>0.9), but discontinuous and fenestrated capillaries have a low σ Moreover, larger proteins have a high σ (>0.9), but smaller proteins (e.g. albumin) have a low σ When the value for σ is very low, plasma and tissue oncotic pressures may have a negligible influence on the net driving force, notice the equation : Qw = K. [(Pc – Pi) –σ (πc – πi)] The actual colloidal osmotic pressure exerted by For example, when we whisk eggs with water, it turns ①Protein concentrations white why? ②Reflection coefficient (σ) -this white substance is called colloid (as the eggs are not dissolved and not accumulated at the bottom due to their weight) ❑ Capillary colloid osmotic pressure (πc) Colloids: is large molecular weight (MW > 30,000, particle size 10-1000 A) particles present in a solution. is derived from the fact that a protein solution resembles a colloidal solution Osmotic pressure: is exerted by molecules or ions that fail to pass through the pores of a semipermeable membrane. is generate by all the dissolved ①solutes different ions (Na, K, Ca ….) ②small non-dissociated molecule (glucose, amino acids) and ③proteins (albumin, globulin ….) is the hydraulic pressure required to prevent the migration of solvent from the area of low solute concentration to an area of high solute concentration Oncotic pressure or Colloid Osmotic Pressure is the pressure exerted by colloidal plasma proteins to reabsorb water back into the blood system, because the plasma proteins are the major colloids present in the plasma and interstitial fluids (i.e., on the two sides of the capillary membrane) that do not readily, pass through the capillary pores Oncotic pressure or Colloid Osmotic Pressure is the part of the osmotic pressure created by proteins. In plasma, the oncotic pressure is only about 0.5% of the total osmotic pressure The total osmotic pressure of the human plasma is 5535mmHg Colloid Osmotic Pressure is 25-30mmHg). This may be a small percent but because colloids cannot cross the capillary membrane easily, oncotic pressure is extremely important in trans-capillary fluid dynamics Because the capillary barrier is readily permeable to ions, the osmotic pressure within the capillary is principally determined by plasma proteins that are relatively impermeable. Therefore, instead of speaking of "osmotic" pressure, this pressure is referred to as the "oncotic" pressure or "colloid osmotic" pressure because it is generated by colloids. The oncotic pressure increases along the length of the capillary, particularly in capillaries having high net filtration (e.g., in renal glomerular capillaries), because the filtering fluid leaves behind proteins leading to an increase in protein concentration ◼ In arteries : the water will move towards the interstitial space from the capillary and the concentration of water in it will decrease BUT The proteins will stay inside Hence, as we move forward towards the venules concentration of water will decrease where the concentration of proteins will increase and colloid osmotic pressure will increase the water will move out of the blood vessels in artery side and into the blood vessels in the vein side Normally, when oncotic pressure is measured, it is measured across a semipermeable membrane that is permeable to fluid and electrolytes but not to large protein molecules. In most capillaries, however, the wall (primarily endothelium) does have a finite ﻣﺤﺪدpermeability to proteins. Because of this finite permeability, the actual oncotic pressure generated across the capillary membrane is less than that calculated from the protein concentration. The actual permeability to protein depends upon ①the type of capillary ②the nature of the protein (size, shape, charge). Plasma colloid osmotic pressure or oncotic pressure tends to cause osmosis of fluid inward through the capillary membrane. The normal value of the capillary plasma oncotic pressure is about 28 mmHg (19mmHg + 9mmHg) o 19 mmHg are caused by molecular effect of dissolved plasma proteins … related to the next slide o 9 mmHg are caused by the cations held in the plasma by proteins … related to : Gibbs donnan effect ❖ Effect of the Different Plasma Proteins on Colloid Osmotic Pressure The plasma proteins are a mixture that contains albumin, globulins, and fibrinogen, with an average molecular weight of 69,000, 140,000, and 400,000, respectively. Thus, 1 gram of globulin contains only half as many molecules as 1 gram of albumin, and 1 gram of fibrinogen contains only one sixth as many molecules as 1 gram of albumin. Osmotic pressure is determined by the number of molecules dissolved in a fluid rather than by the mass of these molecules. Thus, about 80 percent of the total colloid osmotic pressure of the plasma results from the albumin, 20 percent from the globulins, and almost none from fibrinogen. Therefore, from the point of view of capillary and tissue fluid dynamics, it is mainly albumin that is important The charges in the plasma and the charges in the interstitium are the same The difference is only in the concentration ❖ The Gibbs-Donnan effect This occurs because negative charges of proteins exert attraction of Na and other positive charged ions Steps : 1. Cl- diffuses from solution B to A down its concentration gradient. 2. This causes solution A to become electrically negative with respect to solution B. 3. This membrane voltage then drives the diffusion of Na+ from solution B to A (electrical gradient) 4. The accumulation of additional Na+ and Cl- in solution A increases its osmolality and causes water to flow from B to A (osmotic gradient) The Gibbs–Donnan effect is a name for the behavior of charged particles near a semi-permeable membrane that sometimes fail to distribute evenly across the two sides of the membrane It’s caused the presence of a different charged substance that is unable to pass through the membrane and thus creates an uneven electrical charge It is related to cations (Na+ and K+) attached to dissolved plasma proteins & the plasma has a HIGH colloid oncotic pressure ❑ Tissue (interstitial) oncotic pressure (πi) Tends to cause osmosis of fluid outward through the capillary membrane to interstitium Depends on : 1. The interstitial protein concentration 2. The reflection coefficient of the capillary wall … The more permeable the capillary barrier is to proteins, the higher the interstitial oncotic pressure 3. the amount of fluid filtrated into the interstitium “ ﻋﻜﺴﯿﺔ.. protein will be diluted with more fluid” Increased capillary filtration will cause a decrease in interstitial protein concentration (because protein will be diluted with more fluid) This reduces the interstitial oncotic pressure, and this will increase the net oncotic pressure across the capillary endothelium (πC - πi) which opposes filtration and promotes reabsorption thereby serving as a mechanism to limit capillary filtration. In a "typical" tissue, tissue oncotic pressure is about 5 mmHg (much lower than capillary plasma oncotic pressure) because only small amount of plasma protein do leak through the pores into the interstitial fluid so protein concentration in interstitium is 3gm/dL (about 40% of plasma) There is no Gibbs-Donnan effect The concentration of proteins in the interstitium is low as not all proteins can cross to it (some are too large) So the interstitium has a low colloid oncotic pressure ❑ The capillary hydrostatic pressure (Pc) The total hydrostatic pressure from the arterial and Venus side Tends to force fluid outward through the capillary membrane to the interstitium Capillary hydrostatic pressure depending upon the organ 1. Capillary hydrostatic pressure is high in kidney (45mmHg) 2. Capillary hydrostatic pressure low in intestine (10mHg). 3. Capillary hydrostatic pressure in skeletal muscle is nearly 40 mmHg at arterial end and 12 mmHg at venous end. Capillary hydrostatic pressure may drop along the length of the capillary by 15-30 mmHg (axial or longitudinal pressure gradient) The axial gradient favors filtration at the arteriolar end (where PC is greatest) and reabsorption at the venular end of the capillary (where PC is the lowest) Using this equation , we can develop the factors that affect the capillary hydrostatic pressure : 1. Arterial and venous pressures (PA and PV) An increase in either arterial or venous pressure will increase capillary pressure When (RV/RA)=0.2, a given change in arterial pressure is only about one-fifth as effective in changing capillary hydrostatic pressure as a comparable change in venous pressure Therefore, PC is much more influenced by changes in PV than by changes in PA 2. the ratio of postcapillary-to-precapillary resistances (Venous resistance /Arterial resistance) When (RV/RA)=0.2; this means that the precapillary resistance (arteriolar) is about 5-times greater than post-capillary (venular) resistance. Because venous resistance is relatively low, changes in PV are readily transmitted back to the capillary. Conversely, because arterial resistance is relatively high, changes in P A are poorly transmitted downstream to the capillary Radius is inversely proportion to resistance Increase ratio cause increase Capillary hydrostatic pressure Arteriolar constriction ►↓radius ►↑ resistance ► ↓ ratio ►↓ Capillary hydrostatic pressure. Venous constriction ►↓ radius ►↑ resistance ► ↑ ratio ►↑ Capillary hydrostatic pressure. ❑ The tissue (interstitial) hydrostatic pressure (Pi) It depends on two factors : 1. The interstitial fluid volume ↑ fluid that filters into the interstitium >> ↑the volume of the interstitial space (Vi) and ↑ hydrostatic pressure within that space (Pi). 2. The compliance of the tissue interstitium Compliance of the tissue interstitium is defined as the change in volume divided by the change in pressure, and there are two cases : a) In some organs, the interstitial compliance is low Which means that small increases in interstitial volume lead to large increases in pressure. Examples of this include the brain and kidney b) In some organs, the interstitial compliance is high Which means that large increases in interstitial volume lead to small increases in pressure Examples of this include the soft tissues such as skin, muscle and lung ↑ interstitial volume ►↑interstitial pressure ►↓filtration into the interstitium because this pressure opposes the capillary hydrostatic pressure. Large increases in tissue interstitial pressure can lead to tissue damage and cellular death. 1. Normally, interstitial fluid hydrostatic pressure (Pi) is near zero. 2. In some tissues interstitial fluid hydrostatic pressure (Pi) is slightly sub-atmospheric (negative) 3. In some tissues interstitial fluid hydrostatic pressure (Pi) is slightly positive. Interstitial fluid hydrostatic pressure (Pi) tends to force fluid inward when it is positive Interstitial fluid hydrostatic pressure (Pi) tends to force fluid outward when it is negative. Qw = K. [(Pc – Pi) –σ (πc – πi)] The normal interstitial fluid pressure is usually several mmHg negative with respect to the pressure of the surrounds each tissue; for example, in the kidney the capsular pressure surround the kidney average about +13mmHg, whereas the interstitial fluid pressure is +6mmHg. The pressure exerted on the skin is atmospheric pressure, which is considered to be zero pressure, while the interstitial fluid pressure is sub-atmospheric. In most natural cavities of the body where there is free fluid in dynamic equilibrium with the surrounding interstitial fluids, the pressures that have been measured have been negative. Some of these cavities and pressure measurements are as follows: Intra-pleural space: −8 mm Hg Joint synovial spaces: −4 to −6 mm Hg Epidural space: −4 to −6 mm Hg ❖ Significance of negative interstitial fluid pressure as a means for holding the body tissues together Traditionally, it has been assumed that the different tissues of the body are held together entirely by connective tissue fibers. However, connective tissue fibers are very weak or even absent at many places in the body, particularly at points where tissues slide over one another (e.g., skin sliding over the back of the hand or over the face). Yet, even at these places, the tissues are held together by the negative interstitial fluid pressure, which is actually a partial vacuum. When the tissues lose their negative pressure, fluid accumulates in the spaces and the condition known as edema occurs. All of the above forces are seen at the arterial and venous side, but it tends to push fluid toward interstitium at the arterial side and toward the capillary at the venous side. The best example is the skin: Thus, the summation of forces at the arterial end of the capillary shows a net filtration pressure of 13 mmHg, tending to move in the outward direction through the capillary pores. Thus, the summation of forces at the venous end of the capillary shows a net filtration pressure of 7 mmHg, is the (net re-absorption pressure) tending to move in the inward direction through the capillary pores. The re-absorption pressure is considerably less than the filtration pressure at the capillary arterial ends, but remember that ❶ the venous capillary are more numerous and ❷the venous capillary more permeable than the arterial capillaries, so that less pressure is required to cause the inward movement of fluid. The re-absorption pressure causes about (9/10) of the fluid that has filtered out of the arterial of the capillaries to re-absorbed at the venous end. The remainder flows into the lymph vessels.