Viral Replication PowerPoint PDF
Document Details
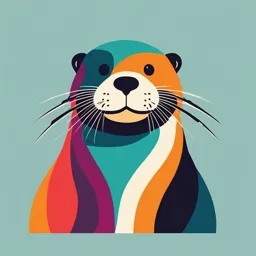
Uploaded by DauntlessSynergy8913
Tags
Summary
This document is a presentation on viral replication, covering the basics of how viruses infect cells and reproduce. It touches on the one-step growth curve, and the processes of adsorption, penetration and uncoating. The presentation also features the mechanisms for RNA and DNA virus replication.
Full Transcript
Viral replication Overview A virus is an infectious agent that is minimally constructed of two components: 1) a genome consisting of either ribonucleic acid (RNA) or deoxyribonucleic acid (DNA), but not both, and 2) a protein-containing structure (capsid) designed to protect the genome (Figure 23....
Viral replication Overview A virus is an infectious agent that is minimally constructed of two components: 1) a genome consisting of either ribonucleic acid (RNA) or deoxyribonucleic acid (DNA), but not both, and 2) a protein-containing structure (capsid) designed to protect the genome (Figure 23.1A). Many viruses have additional structural features, for example, an envelope composed of a protein-containing lipid bilayer, whose presence or absence further distinguishes one virus group from another (Figure 23.1B). A complete virus particle combining these structural elements is called a virion. In functional terms, a virion can be envisioned as a delivery system that surrounds a nucleic acid payload. The delivery system is designed to protect the genome and enable the virus to bind to host cells. The payload is the viral genome and may also include enzymes required for the initial steps in viral replication, a process that is obligately intracellular. The pathogenicity of a virus depends on a great variety of structural and functional characteristics. Therefore, even within a closely related group of viruses, different species may produce significantly distinct clinical pathologies. Characteristics Used to Define Virus Families, Genera, and Species Viruses are divided into related groups, or families, and, sometimes into subfamilies based on: 1) type and structure of the viral nucleic acid, 2) the strategy used in its replication, 3) type of symmetry of the virus capsid (helical versus icosahedral), and 4) presence or absence of a lipid envelope. Within a virus family, differences in additional specific properties, such as host range serologic reactions amino acid sequences of viral proteins, degree of nucleic acid homology, among others, form the basis for division into genera (singular = genus) and species (Figure 23.2). Species of the same virus isolated from different geographic locations may differ from each other in nucleotide sequence. In this case, they are referred to as strains of the same species. Viral Replication: The One-Step Growth Curve The one-step growth curve is a representation of the overall change, with time, in the amount of infectious virus in a single cell that has been infected by a single virus particle. In practice, this is determined by following events in a large population of infected cells in which the infection is proceeding as nearly synchronously as can be achieved by manipulating the experimental conditions. Whereas the time scale and yield of progeny virus vary greatly among virus families, the basic features of the infectious cycle are similar for all viruses. The one-step growth curve begins with the eclipse period, which is followed by a period of exponential growth (Figure 23.7). Eclipse period Following initial attachment of a virus to the host cell, the ability of that virus to infect other cells disappears. This is the eclipse period, and it represents the time elapsed from initial entry and disassembly of the parental virus to the assembly of the first progeny virion During this period, active synthesis of virus components is occurring. The eclipse period for most human viruses falls within a range of one to twenty hours. Exponential growth The number of progeny virus produced within the infected cell increases exponentially for a period of time, then reaches a plateau, after which no additional increase in virus yield occurs. The maximum yield per cell is characteristic for each virus-cell system, and reflects the balance between the rate at which virus components continue to be synthesized and assembled into virions, and the rate at which the cell loses the synthetic capacity and structural integrity needed to produce new virus particles. This may be from 8 to 72 hours or longer, with yields of 100 to 10,000 virions per cell. Steps in the Replication Cycles of Viruses The individual steps in the virus replication cycle are presented below in sequence, virus attachment to the host cell leading to penetration uncoating of the viral genome. Gene expression and replication are followed by assembly and release of viral progeny. Adsorption The initial attachment of a virus particle to a host cell involves an interaction between specific molecular structures on the virion surface and receptor molecules in the host cell membrane that recognize these viral structures (Figure 23.8A). Attachment sites on the viral surface: Some viruses have specialized attachment structures, such as the glycoprotein spikes found in viral envelopes (for example, rhabdoviruses, see p. 310); whereas for others, the unique folding of the capsid proteins forms the attachment sites (for example, picornaviruses, see p 284). In both cases, multiple copies of these molecular attachment structures are distributed around the surface of the virion. [Note: In some cases, the mechanism by which antibodies neutralize viral infectivity is through antibody binding to the viral structures that are required for adsorption (Figure 23.8B).] Host cell receptor molecules: The receptor molecules on the host cell membrane are specific for each virus family. Not surprisingly, these receptors have been found to be molecular structures that usually carry out normal cell functions. For example, cellular membrane receptors for compounds such as growth factors may also inadvertently serve as receptors for a particular virus. Many of the compounds that serve as virus receptors are present only on specifically differentiated cells or are unique for one animal species. Therefore, the presence or absence of host cell receptors is one important determinant of tissue specificity within a susceptible host species, and also for the susceptibility or resistance of a species to a given virus. Penetration Penetration is the passage of the virion from the surface of the cell, across the cell membrane and into the cytoplasm. There are two principal mechanisms by which viruses enter animal cells: receptor-mediated endocytosis direct membrane fusion. Receptor-mediated endocytosis: This is basically the same process by which the cell internalizes compounds such as growth regulatory molecules and serum lipoproteins, except the infecting virus particle is bound to the host cell surface receptor in place of the normal ligand (Figure 23.9). The cell membrane invaginates, enclosing the virion in an endocytotic vesicle (endosome). Release of the virion into the cytoplasm occurs by various routes, depending on the virus but, in general, it is facilitated by one or more viral molecules. In the case of an enveloped virus, its membrane may fuse with the membrane of the endosome, resulting in the release of the nucleocapsid into the cytoplasm. Membrane fusion Some enveloped viruses (for example, human immunodeficiency virus, see p. 297) enter a host cell by fusion of their envelope with the plasma membrane of the cell (Figure 23.10). One or more of the glycoproteins in the envelope of these viruses promotes the fusion. The end result of this process is that the nucleocapsid is free in the cytoplasm, whereas the viral membrane remains associated with the plasma membrane of the host cell. Uncoating refers to the stepwise process of disassembly of the virion that enables the expression of the viral genes that carry out replication. For enveloped viruses, the penetration process itself is the first step in uncoating. In general, most steps of the uncoating process occur within the cell and depend on cellular enzymes; however in some of the more complex viruses, newly synthesized viral proteins are required to complete the process. The loss of one or more structural components of the virion during uncoating predictably leads to a loss of the ability of that particle to infect other cells, which is the basis for the eclipse period of the growth curve (see Figure 23.7). It is during this phase in the replication cycle that viral gene expression begins. Mechanisms of DNA virus genome replication Each virus family differs in significant ways from all others in terms of the details of the macromolecular events comprising the replication cycle. The wide range of viral genome sizes gives rise to great differences in the number of proteins for which the virus can code. In general, the smaller the viral genome, the more the virus must depend on the host cell to provide the functions needed for viral replication For example, some small DNA viruses, such as polyomavirus (see p. 249), produce only one or two replication-related gene products, which function to divert host cell processes to those of viral replication. Other larger DNA viruses, such as poxvirus (see p. 270) provide virtually all enzymatic and regulatory molecules needed for a complete replication cycle. Figure 23.11 outlines the essential features of gene expression and replication of DNA viruses Mechanisms of RNA virus genome replication Viruses with RNA genomes must overcome two specific problems that arise from the need to replicate the viral genome, and to produce a number of viral proteins in eukaryotic host cells. First, there is no host cell RNA polymerase that can use the viral parental RNA as a template for synthesis of complementary RNA strands. Second, translation of eukaryotic mRNAs begins at only a single initiation site, and they are, therefore, translated into only a single polypeptide. However, RNA viruses, which frequently contain only a single molecule of RNA, must express the genetic information for at least two proteins: an RNA-dependent RNA polymerase and a minimum of one type of capsid protein. Although the replication of each RNA virus family has unique features, the mechanisms evolved to surmount these restrictions can be grouped into four broad patterns of replication. Type I: RNA viruses with a single-stranded genome (ssRNA) of (+) polarity that replicates via a complementary (-) strand intermediate: In Type I viral replication, the infecting parental RNA molecule serves both as mRNA and later as a template for synthesis of the complementary (-) strand (Figure 23.12). Role of (+) ssRNA as mRNA: Because the parental RNA genome is of (+), or messenger, polarity, it can be translated directly upon uncoating and associating with cellular ribosomes. The product is usually a single polyprotein from which individual polypeptides, such as RNA-dependent RNA polymerase and various proteins of the virion, are cleaved by a series of proteolytic processing events carried out by a protease domain of the polyprotein (see Figure 23.12). Role of (+) ssRNA as the template for complementary (-) strand synthesis: The viral (+) ssRNA functions early in infection, not only as mRNA for translation of polyproteins but also as a template for virus- encoded RNA-dependent RNA polymerase to synthesize complementary (-) ssRNA (see Figure 23.12). The progeny (-) strands, in turn, serve as templates for synthesis of progeny (+) strands, which can serve as additional mRNAs, amplifying the capacity to produce virion proteins for progeny virus. When a sufficient quantity of capsid proteins has accumulated later in the infection, progeny (+) ssRNAs begin to be assembled into newly formed nucleocapsids. Type II: Viruses with a ssRNA genome of (-) polarity that replicate via a complementary (+) strand intermediate: Viral genomes with (-) polarity, such as the (+) strand genomes, also have two functions: 1) to provide information for protein synthesis, and 2) to serve as templates for replication. Unlike (+) strand genomes, however, the (-) strand genomes cannot accomplish these goals without prior construction of a complementary (+) strand intermediate (Figure 23.13). A) Mechanism of replication of viral ssRNA with (-) polarity: The replication problems for these viruses are two-fold. First, the (-) strand genome cannot be translated and, therefore, the required viral RNA polymerase cannot be synthesized immediately following infection. Second, the host cell has no enzyme capable of transcribing the (-) strand RNA genome into (+) strand RNAs capable of being translated. The solution to these problems is for the infecting virus particle to contain viral RNA-dependent RNA polymerase, and to bring this enzyme into the host cell along with the viral genome. As a consequence, the first synthetic event after infection is transcription of (+) strand mRNAs from the parental viral (-) strand RNA template. B) Mechanisms for multiple viral protein synthesis in Type II viruses: The synthesis of multiple proteins is achieved in one of two ways among the (-) strand virus families: 1) the viral genome may be a polycistronic molecule, from which transcription produces a number of mRNAs, each specifying a single polypeptide; 2) alternatively, the (-) strand viral genome may be segmented (that is, composed of a number of different RNA molecules, most of which code for a single polypeptide). Production of infectious virus particles: Although the details differ, the flow of information in both segmented and unsegmented genome viruses is basically the same. In the Type II replication scheme, an important control element is the shift from synthesis of (+) strand mRNAs to production of progeny (-) strand RNA molecules that can be packaged in the virions. This shift is not a result of different polymerase, but rather to the sequestering of (+) strand RNA molecules by interaction with one or more of the newly synthesized proteins. This makes the (+) strands available as templates for the synthesis of genomic (-) strands. Further, segmented genome viruses have the additional problem of assuring that all segments are incorporated into the progeny virions. The mechanism by which this occurs is not clear. Type III: Viruses with a dsRNA genome. The dsRNA genome is segmented, with each segment coding for one polypeptide. However, eukaryotic cells do not have an enzyme capable of transcribing dsRNA. Type III viral mRNA transcripts are, therefore, produced by virus-coded, RNA-dependent RNA polymerase (transcriptase) located in a subviral core particle. This particle consists of the dsRNA genome and associated virion proteins, including the transcriptase. The mechanism of replication of the dsRNA is unique, in that the (+) RNA transcripts are not only used for translation, but also as templates for complementary (-) strand synthesis, resulting in the formation of dsRNA progeny. Type IV: Viruses with a genome of ssRNA of (+) polarity that is replicated via a DNA intermediate. The conversion of a (+) strand RNA to a double-stranded DNA is accomplished by an RNA-dependent DNA polymerase, commonly referred to as a reverse transcriptase, that is contained in the virion. The resulting dsDNA becomes integrated into the cell genome by the action of a viral integrase. Viral mRNAs and progeny (+) strand RNA genomes are transcribed from this integrated DNA by the host cell RNA polymerase. Assembly and release of progeny viruses. Assembly of nucleocapsids generally takes place in the host cell compartment where the viral nucleic acid replication occurs (that is, in the cytoplasm for most RNA viruses and in the nucleus for most DNA viruses). For DNA viruses, this requires that capsid proteins be transported from their site of synthesis (cytoplasm) to the nucleus. The various capsid components begin to self- assemble, eventually associating with the nucleic acid to complete the nucleocapsid. Naked viruses: In naked (unenveloped) viruses, the virion is complete at this point. Release of progeny is usually a passive event resulting from the disintegration of the dying cell and, therefore, may be at a relatively late time after infection. Enveloped viruses: In enveloped viruses, virus-specific glycoproteins are synthesized and transported to the host cell membrane in the same manner as cellular membrane proteins. When inserted into the membrane, they displace the cellular glycoproteins, resulting in patches on the cell surface that have viral antigenic specificity. The cytoplasmic domains of these proteins associate specifically with one or more additional viral proteins (matrix proteins) to which the nucleocapsids bind. Final maturation then involves envelopment of the nucleocapsid by a process of budding (Figure 23.16). A consequence of this mechanism of viral replication is that progeny virus are released continuously while replication is proceeding within the cell and ends when the cell loses its ability to maintain the integrity of the plasma membrane. A second consequence is that with most enveloped viruses, all infectious progeny are extracellular. The exceptions are those viruses that acquire their envelopes by budding through internal cell membranes, such as those of the endoplasmic reticulum or nucleus. Viruses containing lipid envelopes are sensitive to damage by harsh environments and, therefore, tend to be transmitted by the respiratory, parenteral, and sexual routes. Nonenveloped viruses are more stable to hostile environmental conditions and often transmitted by the fecal-oral route. Effects of viral infection on the host cell The response of a host cell to infection by a virus ranges from: 1) little or no detectable effect; 2) alteration of the antigenic specificity of the cell surface due to presence of virus glycoproteins; 3) latent infections that, in some cases, cause cell transformation; or, ultimately, 4) cell death due to expression of viral genes that shut off essential host cell functions (Figure 23.17). Viral infections in which no progeny virus are produced: In this case, the infection is referred to as abortive. An abortive response to infection is commonly caused by: 1) a normal virus infecting cells that are lacking in enzymes, promoters, transcription factors, or other compounds required for complete viral replication, in which case the cells are referred to as nonpermissive; 2) infection by a defective virus of a cell that normally supports viral replication (that is, by a virus that itself has genetically lost the ability to replicate in that cell type); or 3) death of the cell as a consequence of the infection, before viral replication has been completed. Viral infections in which the host cell may be altered antigenically but is not killed, although progeny virus are released: In this case, the host cell is permissive, and the infection is productive (progeny virus are released from the cell), but viral replication and release neither kills the host cell nor interferes with its ability to multiply and carry out differentiated functions. The infection is therefore said to be persistent. The antigenic specificity of the cell surface may be altered as a result of the insertion of viral glycoproteins. Viral infections that result in a latent viral state in the host cell: Some viral infections result in the persistence of the viral genome inside a host cell with no production of progeny virus. Such latent viruses can be reactivated months or years in the future, leading to a productive infection. Some latently infected cells contain viral genomes that are stably integrated into a host cell chromosome. This can cause alterations in the host cell surface, cellular metabolic functions, and, significantly, cell growth and replication patterns. Such viruses may induce tumors in animals; in this case, they are said to be tumor viruses, and the cells they infect are transformed. Viral infections resulting in host cell death and production of progeny virus Eliminating host cell competition for synthetic enzymes and precursor molecules increases the efficiency with which virus constituents can be synthesized. Therefore, the typical result of a productive (progeny-yielding) infection by a cytocidal virus is the shut-off of much of the cell's macromolecular syntheses by one or more of the virus gene products, causing the death of the cell. Such an infection is said to be lytic. The mechanism of the shut-off varies among the viral families. Viral infections Chickenpox (Varicella) and Shingles (Zoster) Chickenpox (varicella) is a highly contagious skin disease primarily of children 2 to 7 years of age. It is estimated that about 4 million cases occur annually in the United States. The causative agent is the varicella-zoster virus, a member of the family Herpesviridae, which is acquired by droplet inhalation into the respiratory system. The virus produces at least six glycoproteins that play a role in viral attachment to specific receptors on respiratory epithelial cells, and their recognition by the human immune system results in humoral and cellular immunity. Following an incubation period of from 10 to 23 days, small vesicles erupt on the face or upper trunk, fill with pus, rupture, and become covered by scabs (figure 38.1). Healing of the vesicles occurs in about 10 days. During this time intense itching often occurs. Chickenpox can be prevented or the infection shortened with an attenuated varicella vaccine (Varivax; see table 33.1) or the drug acyclovir (Zovirax or Valtrex). Healing of the vesicles occurs in about 10 days. During this time intense itching often occurs. Chickenpox can be prevented or the infection shortened with an attenuated varicella vaccine (Varivax; see table 33.1) or the drug acyclovir (Zovirax or Valtrex). Individuals who recover from chickenpox are subsequently immune to this disease; however, they are not free of the virus, as viral DNA resides in a dormant (latent) state within the nuclei of cranial nerves and sensory neurons in the dorsal root ganglia. This viral DNA is maintained in infected cells but virions cannot be detected (figure 38.2a). When the infected person becomes immunocompromised by such factors as age, AIDS, or psychological or physiological stress, the viruses may become activated (figure 38.2b). They migrate down sensory nerves, initiate viral replication, and produce painful vesicles (figure 38.2c) because of sensory nerve damage. This syndrome is called postherpetic neuralgia. This reactivated form of chickenpox is termed shingles (zoster). Most cases occur in people over 50 years of age. Shingles does not require specific therapy; however, in immunocompromised individuals, acyclovir (Zovirax or Valtrex; see figure 35.14), vidarabine (Vira-A), or famciclovir (Famvir) are recommended. More than 300,000 cases of zoster occur annually in the United States. Influenza (Flu) Influenza [Italian, to be influenced by the stars—un influenza di freddo], or the flu, is a respiratory system disease caused by orthomyxoviruses. Influenza viruses (see figures 16.10i, 16.17a, b) are classified into A, B, and C groups based on the antigens (H and N) of their protein coats. One unique feature of the influenza viruses is the frequency with which changes in antigenicity occur. These changes are called antigenic variation. If the variation is small, it is called antigenic drift; if it is large, it is called antigenic shift. Antigenic variation occurs almost yearly with the influenza A virus, less frequently with the B virus, and has not been demonstrated with the C virus. Animal reservoirs are critical to the epidemiology of human influenza. For example, rural China is one region of the world where chickens, pigs, and humans live in close, crowded conditions. Animal reservoirs are critical to the epidemiology of human influenza. For example, rural China is one region of the world where chickens, pigs, and humans live in close, crowded conditions. Influenza is widespread in chickens; although chickens can’t transmit the virus to humans, they can transfer it to pigs. Pigs can transfer it to humans and humans, back to pigs. Recombination between human and avian strains thus occurs in pigs, leading to major antigenic shifts This explains why influenza continues to be a major epidemic disease and frequently produces worldwide pandemics. The worst pandemic on record occurred in 1918 and killed more than 20 million people. This disaster, traced to the Spanish influenza virus (see figure 37.7), was followed by pandemics of Asian flu (1957), Hong Kong flu (1968), and Russian flu (1977). (The names reflect popular impressions of where the episodes began, although all are now thought to have originated in China.) The virus (see figures 16.17a, 18.7, 18.9) is acquired by inhalation or ingestion of virus-contaminated respiratory secretions. During an incubation period of 1 to 2 days, the virus adheres to the epithelium of the respiratory system (the neuraminidase present in envelope spikes may hydrolyze the mucus that covers the epithelium). The virus attaches to the epithelial cell by its hemagglutinin spike protein, causing part of the cell’s plasma membrane to bulge inward, seal off, and form a vesicle (receptor-mediated endocytosis). This encloses the virus in an endosome. The hemagglutinin molecule in the virus envelope undergoes a dramatic conformational change when the endosomal pH decreases. The hydrophobic ends of the hemagglutinin spring outward and extend toward the endosomal membrane. After they contact the membrane, fusion occurs and the RNA nucleocapsid is released into the cytoplasmic matrix. Influenza is characterized by chills, fever, headache, malaise, and general muscular aches and pains. These symptoms arise from the death of respiratory epithelial cells, probably due to attacks by activated T cells. Recovery usually occurs in 3 to 7 days, during which coldlike symptoms appear as the fever subsides. Influenza alone usually is not fatal. However, death may result from pneumonia caused by secondary bacterial invaders such as Staphylococcus aureus, Streptococcus pneumoniae, and Haemophilus influenzae. A commercially available identification technique is Directigen FLU-A (an enzyme immunoassay [EIA] rapid test). This test can detect influenza A virus in clinical specimens in less than 15 minutes As with many other viral diseases, only the symptoms of influenza usually are treated. However, the antiviral drugs amantadine (Symmetrel) (see figure 35.14), rimantadine (Flumadine), zanamivir (Relenza), and oseltamivir (Tamiflu) have been shown to reduce the duration and symptoms of type A influenza if administered during the first two days of illness. All four of these drugs attack the virus directly by plugging the catalytic site of the enzyme neuramidase. With the enzyme inactivated, viral particles can’t travel from cell to cell. Aspirin (salicylic acid) should b avoided in children younger than 14 years to reduce the risk of Reye’s syndrome (Box 38.1). The mainstay for prevention of influenza since the late 1940s has been inactivated virus vaccines, (see table 33.1), especially for the chronically ill, individuals over age 65, residents of nursing homes, and health-care workers in close contact with people at risk. Currently three influenza A virus subtypes are epidemic in humans: those with H1N1, H2N1, and H3N2 hemagglutinin and neuraminidase surface glycoproteins. Influenza A infections usually peak in the winter and involve 10% or more of the population, with rates of 50 to 75% in school-age children. Influenza B usually accounts for only 3% of all flu cases in the United States.