Semiconductor Devices and Rectifiers PDF
Document Details
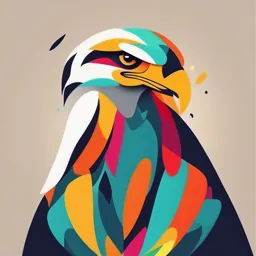
Uploaded by WellConnectedPlumTree9039
Tags
Summary
These lecture notes cover the fundamentals of semiconductor devices, including diodes, transistors, and operational amplifiers. They also detail different types of rectifiers, such as half-wave and full-wave rectifiers, along with the characteristics and applications of these devices.
Full Transcript
Fundamentals of semiconductor devices : introduction to semiconductor devices, basic operation and V-I characteristics of semiconductor diode, basic operation and V-I characteristics of Zener diode, type of transistor, transistor action, input- output characteristics of CE - configuration,...
Fundamentals of semiconductor devices : introduction to semiconductor devices, basic operation and V-I characteristics of semiconductor diode, basic operation and V-I characteristics of Zener diode, type of transistor, transistor action, input- output characteristics of CE - configuration, MOSFET representation and characteristics, characteristics of an ideal and practical operational amplifier (IC 741), open and closed loop configuration and concept of virtual ground Holes and Electrons in Semiconductors Holes and electrons are the types of charge carriers accountable for the flow of current in semiconductors. Holes (valence electrons) are the positively charged electric charge carrier, whereas electrons are the negatively charged particles. Both electrons and holes are equal in magnitude but opposite in polarity. Electrons travel in the conduction band, whereas holes travel in the valence band. When an electric field is applied, holes cannot move as freely as electrons due to their restricted movement. Acceptor Germanium (32) and silicon (14) are the most common types of intrinsic semiconductor elements. They have four valence electrons (tetravalent). They are bound to the atom by a covalent bond at absolute zero temperature. When the temperature rises due to collisions, few electrons are unbounded and become free to move through the lattice, thus creating an absence in its original position (hole). These free electrons and holes contribute to the conduction of electricity in the semiconductor. The negative and positive charge carriers are equal in number. The thermal energy is capable of ionising a few atoms in the lattice, and hence, their conductivity is less. Forward Biased Built-in Potential Si=0.7 V Injection/Diffusion Recombination Ge=0.3 V KT/q=26 mV at room temperature Q1. When is the pn junction said to be forward biased? Q2. What type of biasing gives a semiconductor diode low resistance? Q3. What happens to the depletion region in forward bias? Q4. Why is biasing needed? https://www.slideshare.net/ piyush210987/unit-1-numerical-problems- on-pn-junction-diode Peak inverse voltage (PIV) or peak reverse voltage (PRV) maximum voltage a diode can withstand in the reverse-biased direction before breakdown. Less PIV means less pressure on the diode. Small PIV diodes also are less costly in comparison to larger PIV diodes. PIV= Vm for the HWR. PIV= 2Vm for the Center Tap rectifier. PIV= Vm for the bridge rectifier. The transformer utilization factor (TUF) of a rectifier circuit is defined as the ratio of the DC power available at the load resistor to the AC rating of the secondary coil of a transformer. TUF= 0.27 for the HWR. TUF= 0.67 for the Center Tap rectifier. TUF= 0.810 for the bridge rectifier. The more the value of TUF, the more will be the utilization. Construction of a full wave bridge rectifier The construction of a bridge rectifier is shown in the figure below. The bridge rectifier circuit is made of four diodes D1, D2, D3, D4, and a load resistor RL. The four diodes are connected in a closed-loop configuration to efficiently convert the alternating current (AC) into Direct Current (DC). The main advantage of this configuration is the absence of the expensive centre-tapped transformer. Therefore, the size and cost are reduced. The input signal is applied across terminals A and B, and the output DC signal is obtained across the load resistor RL connected between terminals C and D. The four diodes are arranged in such a way that only two diodes conduct electricity during each half cycle. D1 and D3 are pairs that conduct electric current during the positive half cycle/. Likewise, diodes D2 and D4 conduct electric current during a negative half cycle. Working When an AC signal is applied across the bridge rectifier, terminal A becomes positive during the positive half cycle while terminal B becomes negative. This results in diodes D1 and D3 becoming forward biased while D2 and D4 becoming reverse biased. The current flow during the positive half-cycle is shown in the figure. During the negative half- cycle, terminal B becomes positive while terminal A becomes negative. This causes diodes D2 and D4 to become forward biased and diode D1 and D3 to be reverse biased. The current flow during the negative half cycle is shown in the figure. Characteristics of Bridge Rectifier Ripple Factor The smoothness of the output DC signal is measured by a factor known as the ripple factor. The output DC signal with fewer ripples is considered a smooth DC signal while the output with high ripples is considered a high pulsating DC signal. Mathematically, the ripple factor is defined as the ratio of ripple voltage to pure DC voltage. The ripple factor for a bridge rectifier is given by Peak Inverse Voltage The maximum voltage that a diode can withstand in the reverse bias condition is known as a peak inverse voltage. During the positive half cycle, the diodes D1 and D3 are in the conducting state while D2 and D4 are in the non-conducting state. Similarly, during the negative half cycle, diodes D2 and D4 are in the conducting state, and diodes D1 and D3 are in the non- conducting state. PIV=Vm Efficiency The rectifier efficiency determines how efficiently the rectifier converts Alternating Current (AC) into Direct Current (DC). Rectifier efficiency is defined as the ratio of the DC output power to the AC input power. The maximum efficiency of a bridge rectifier is 81.2%. Advantages The efficiency of the bridge rectifier is higher than the efficiency of a half-wave rectifier. However, the rectifier efficiency of the bridge rectifier and the centre-tapped full-wave rectifier is the same. The DC output signal of the bridge rectifier is smoother than the output DC signal of a half-wave rectifier. In a half-wave rectifier, only half of the input AC signal is used, and the other half is blocked. Half of the input signal is wasted in a half-wave rectifier. However, in a bridge rectifier, the electric current is allowed during both positive and negative half cycles of the input AC signal. Hence, the output DC signal is almost equal to the input AC signal. Disadvantages The circuit of a bridge rectifier is complex when compared to a half-wave rectifier and centre-tapped full- wave rectifier. Bridge rectifiers use 4 diodes while half- wave rectifiers and centre-tapped full wave rectifiers use only two diodes. When more diodes are used more power loss occurs. In a centre-tapped full-wave rectifier, only one diode conducts during each half cycle. But in a bridge rectifier, two diodes connected in series conduct during each half cycle. Hence, the voltage drop is higher in a bridge rectifier. Zener Diode A Zener diode is a highly doped semiconductor device specifically designed to function in the reverse direction. It is engineered with a wide range of Zener voltages (Vz), and certain types are even adjustable to achieve variable voltage regulation. No collision as depletion region is thin Extra free electrons will be generated as E=V/d E is high that result in covalent bond breakage and free e- will be part of current conduction The V-I characteristics of a Zener diode can be divided into two parts as follows: (i) Forward Characteristics (ii) Reverse Characteristics Forward Characteristics of Zener Diode The first quadrant in the graph represents the forward characteristics of a Zener diode. From the graph, we understand that it is almost identical to the forward characteristics of P-N junction diode. Reverse Characteristics of Zener Diode When a reverse voltage is applied to a Zener voltage, a small reverse saturation current Io flows across the diode. This current is due to thermally generated minority carriers. As the reverse voltage increases, at a certain value of reverse voltage, the reverse current increases drastically and sharply. This is an indication that the breakdown has occurred. We call this voltage breakdown voltage or Zener voltage, and Vz denotes it. Zener diode as a Voltage Regulator Q1. For the circuit shown in Fig.1 (i), Find: (i) the output voltage (ii) the voltage drop across series resistance (iii) the current through zener diode. For the circuit shown in Fig. 2 (i),find the maximum and minimum values of zener diode current. A 7.2 V zener is used in the circuit shown in Fig. 3 and the load current is to vary from 12 to 100 mA. Find the value of series resistance R to maintain a voltage of 7.2 V across the load. The input voltage is constant at 12V and the minimum zener current is 10 mA. Transistor Transistors are one of the key components in most of the electronic devices that are present today. Developed in the year 1947 by three American physicists, John Bardeen, Walter Brattain and William Shockley, the transistor is considered one of the most important inventions in the history of science. A transistor is a type of semiconductor device that can be used to conduct and insulate electric current or voltage. A transistor basically acts as a switch and an amplifier. Parts of a Transistor A typical transistor is composed of three layers of semiconductor materials or, more specifically, terminals which help to make a connection to an external circuit and carry the current. A voltage or current that is applied to any one pair of the terminals of a transistor controls the current through the other pair of terminals. There are three terminals for a transistor. They are listed below: Base: This is used to activate the transistor. Collector: It is the positive lead of the transistor. Emitter: It is the negative lead of the transistor. BJT-Bipolar Junction Transistor The three terminals of BJT are the base, emitter and collector. A very small current flowing between the base and emitter can control a larger flow of current between the collector and emitter terminal. Furthermore, there are two types of BJT, and they include: P-N-P Transistor: It is a type of BJT where one n-type material is introduced or placed between two p-type materials. In such a configuration, the device will control the flow of current. PNP transistor consists of 2 crystal diodes which are connected in series. The right side and left side of the diodes are known as the collector-base diode and emitter-base diode, respectively. N-P-N Transistor: In this transistor, we will find one p- type material that is present between two n-type materials. N-P-N transistor is basically used to amplify weak signals to strong signals. In an NPN transistor, the electrons move from the emitter to the collector region, resulting in the formation of current in the transistor. This transistor is widely used in the circuit. There are three types of configuration, which are common base (CB), common collector (CC) and common emitter (CE). In a common base connection, IE = 1mA, IC = 0.95mA. Calculate the value of IB. A common base transistor amplifier has an input resistance of 20 Ω and output resistance of 100 kΩ. The collector load is 1 kΩ. If a signal of 500 mV is applied between emitter and base, find the voltage amplification. Assume αac to be nearly one. Common Collector Configuration CE Configuration Two terminals are needed for input and two terminals for output. Transistors have three terminals, so one terminal have to be taken as common terminal for both input and output. In CE configuration, emitter terminal is taken as common for both input and output. Input is given between base-emitter terminals Output is taken between collector-emitter terminals. This is the most commonly used configuration. The input side is forward biased and the output side is reverse biased. Input voltage-VBE Output voltage-VCE The output current IC is taken across the EC terminals. Emitter base region acts like forward biased diode and so the depletion region is very small. Emitter collector region acts like reverse biased diode and the depletion region is large. The input current IB is measured in µA because the base region is very lightly doped. The input and output impedance are moderate in common emitter configuration and thus the current and voltage gain is moderate and the power gain is high. So this configuration is widely used for amplification. Input characteristics are the relationship between the input current and the input voltage keeping output voltage constant. Here the input current is the base current IB, input voltage is base emitter voltage VBE and the output voltage is collector emitter voltage VCE. First the output voltage VCE is kept at zero and the input voltage VBE is gradually increased and the input current IB is noted. Then again the output voltage VCE is increased like 10V, 20V and kept constant and by increasing the input voltage VBE, the input current IB is noted. From the results it is observed that when the input voltage VBE is increased initially there is no current produced, further when it is increased the input current IB increases steeply. When the output voltage VCE is further increased the curve shifts right side. It has a value between 20 to 500 Output characteristics is the relationship between the output current and the output voltage keeping input current constant. Here the values of output current IC and the output voltage VCE is noted keeping input current IB constant. In active region when the output voltage is increased there is very slight change in the output voltage. The curve looks almost flat in the active region. Cut off region is the region where the input current is below zero. When both the junctions are forward biased, it is in saturation region. In a common base connection, current amplification factor is 0.9. If the emitter current is 1mA, determine the value of base current. In a common base connection, IC = 0.95 mA and IB = 0.05 mA. Find the value of α. In a common base connection, the emitter current is 1mA. If the emitter circuit is open, the collector current is 50 μA. Find the total collector current. Given that α = 0.92. In a common base connection, the emitter current is 5mA. If the emitter circuit is open, the collector current is 70 μA. Find the total collector current. Given that α = 0.96. Transistor as Amplifier A transistor is a three terminal semiconductor device, and the terminals are E(Emitter), B (Base) & C (Collector). The transistor can work in three different regions like active region, cutoff region & saturation region. Transistors are turned off while working in the cut-off region and turned on while working in the saturation region. Transistors work as an amplifier while they work in the active region. The main function of a transistor as an amplifier is to enhance the input signal without changing much. The transistor configurations are classified into three types such as CB (common base), CC (common collector), and CE (common emitter). But common emitter configuration is frequently used in the applications like an audio amplifier. Because in CB configuration, the gain is VGS1, IDSS3 is greater than IDSS2 as VGS3 > VGS2, so on and so forth. Further, Figure 1b also shows the locus of pinch-off voltage (black discontinuous curve), from which VP is seen to increase with an n-channel Depletion-type MOSFET The transfer characteristics of n-channel depletion MOSFET shown by Figure 3a indicate that the device has a current flowing through it even when VGS is 0V. This indicates that these devices conduct even when the gate terminal is left unbiased, which is further emphasized by the VGS0 curve of Figure 3b. Under this condition, the current through the MOSFET is seen to increase with an increase in the value of VDS (Ohmic region) untill VDS becomes equal to pinch-off voltage VP. After this, IDS will get saturated to a particular level IDSS (saturation region of operation) which increases with an increase in VGS i.e. IDSS3 > IDSS2 > IDSS1, as VGS3 > VGS2 > VGS1. Further, the locus of the pinch-off voltage also shows that VP increases with an increase in VGS. However it is to be noted that, if one needs to operate these devices in cut-off state, then it is required to make VGS negative and once it becomes equal to -VT, the conduction through the device stops (IDS = 0) as it gets deprived of its n-type channel (Figure 3a). Threshold Voltage The value of the gate-to-source voltage VGS needed to create (induced) the conducting channel (to cause surface inversion) is called the threshold voltage and denoted with Vth or Vt. The value of the threshold voltage is dependent from some physical parameters which characterize the MOSFET structure such as: the gate material, the thickness of oxide layer tox, substrate doping concentrations (density) NA, oxide –interface fixed charge concentrations (density) Nox, channel length L, channel width W and the bias voltage VSB. Varactor Diode Varactor diode is a type of diode whose internal capacitance varies with respect to the reverse voltage. It always works in reverse bias conditions and is a voltage-dependent semiconductor device. Several names know varactor diode as Varicap, Voltcap, Voltage variable capacitance, or Tunning diode. Operation of Varactor Diode The junction capacitance of a p-n junction diode is inversely proportional to the width of the depletion layer. In other words, if the width of the depletion layer is less, then the capacitance is more, and vice versa. So if we need to increase the capacitance of a varactor diode, the reverse bias voltage should be decreased. It causes the width of the depletion layer to decrease, resulting in higher capacitance. Similarly, increasing the reverse bias voltage should decrease the capacitance. This ability to get different values of capacitances just by changing the voltage applied is the biggest advantage of a varactor diode compared to a normal variable capacitor.