Unit Notes - Ocean Science PDF
Document Details
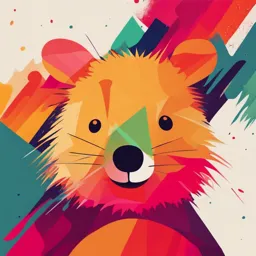
Uploaded by MightyMossAgate1509
Tags
Summary
These unit notes cover a range of topics related to ocean science, including marine life, ocean systems, climate change, and ocean stewardship. The modular structure provides a framework for understanding the complex connections within ocean ecosystems and human impact. The notes also include images/diagrams, relevant assessment tasks, and discussion questions.
Full Transcript
**Unit description:** Seventy percent of the planet is covered by ocean. In this unit, we discover ocean connections and iconic and 'weird' marine life, give insight into 'a day in the life' of an oceanographer, and explore several topics highly relevant to modern society. These include technologies...
**Unit description:** Seventy percent of the planet is covered by ocean. In this unit, we discover ocean connections and iconic and 'weird' marine life, give insight into 'a day in the life' of an oceanographer, and explore several topics highly relevant to modern society. These include technologies for ocean science, responding to climate change, conserving threatened species, and sustainable management of marine resources including fisheries. **Module 1: Marine life**\ Topics of marine life and the ecology of temperate and high latitude marine ecosystems. 1. Iconic sea life 2. Sharks 3. Origin of life 4. Marine food webs 5. Antarctic food webs 6. Temperate kelp forests 7. Deep sea and fascinating factoids **Module 2:** **The ocean system**\ The Ocean system The interactions between physical, chemical, geological, and ecological features. 1. Ocean system 2. Primary production 3. Pumping carbon: the oceans role in climate 4. The iron age of oceanography 5. Ocean observations with satellites, robots and animals 6. Ocean circulation 7. Ocean eddies, waves and mixing 8. Plate tectonics: shaping the oceans 9. Antarctica and the southern ocean 10. Climate and weather **Module 3: Climate Change and multiple stressors**\ The role of the oceans in climate change and how climate change affects ocean life. 1. Climate change and multiple stressors 2. Ice age: how has our climate changed before? 3. Do you live near a hot spot? 4. Who cares about a few degrees? 5. Consequences of alters circulation 6. Ocean acidification 7. Cumulative environmental stress 8. The East Australian Current (EAC) 9. Acclimation and adaption: what's the difference? 10. How does all of this affect us? **Module 4: Stewardship of the oceans**\ How human activity impacts the oceans and the responsibilities of humans in ocean stewardship. 1. Stewardship of the oceans: pressures on ocean systems 2. Balancing extractive use and conservation 3. Threatened species and systems 4. Where does our seafood come from? 5. Sustainable fishing and the future 6. Patterns in nature: extinctions, populations trends and biodiversity 7. How we count fish and other tails 8. Highly migratory species: significance, management, and challenges 9. Antarctic recourse management **Task \#** **Assessment task** **Due Date** **Weight** ------------- ------------------------------ -------------- ------------ **1** Module quizzes 13-Jan-2025 6% **2** Module tweets 17-Jan-2025 10% **3** Fake-news rebuttal 20-Jan-2025 24% **4** Tutorial/Forum participation 31-Jan-2025 5% **5** Essay 31-Jan-2025 25% **6** Final exam 3&4-Feb-2025 30% **Communication \> Discussions \> Student Forum** **! for topics !** - Watch vids - Join discussion forums (bc im at work/in school for most of the tuts join forums for task 4) - Complete quizzes by 13^th^ Jan 1. **Iconic sea life** [What is 'iconic'?] Iconic sea life includes unique marine organisms that captivate human fascination, like massive whales and delicate seaweeds, showcasing remarkable adaptations to ocean life. [Extraordinary and unique sea life] Many taxa (organisms which are grouped/classified in units arranged from kingdom to subspecies in the Linnean classification system -- refer to image 1.1.3) can only be found in the ocean; some of these are very well known to the public while others are almost never seen. For example, starfish are a common\ symbol of seashores and are easily recognisable to everyone, but how many realise that there are 1,500 different species that range from the seashores to abyssal depths? Another unique group is the Nudibranchs (sea slugs) a soft-bodied marine gastropod. Again another remarkable, colourful and well known group are the corals. Corals are tiny Cnidarians (jellyfish) which typically live in dense colonies. Despite their tiny individual size, the colonies can be massive,\ forming shallow water reefs that can extends over hundreds of kilometres, which are themselves crucially important habitats for a vast diversity of marine life. [Sealife that can kill you!] Some marine animals kill through toxins, such as the box jellyfish, cone shells, blue-ringed octopuses and stonefish. Others are top predators such as killer whales and sharks. Of course, not all sharks are predators of humans; in fact the majority of species are quite harmless. However other\ species such as the great white shark are\ deeply embedded in out psyches. [Seabirds] Several groups of marine animals have evolutionary histories that have seen them evolve on land but have consequently become adapted to life in the marine environment. A good example are the seabirds, of which there are 4 families and over 400 species. Most seabirds are easily recognisable as birds, having many morphological traits in common with their terrestrial counterparts. The penguins, however, have become so specialised to living in a marine environment that they have a number of very un-birdlike traits, such as dense bones and a reduction in the size and shape of their wings, so much so that they have lost the power of flight. These changes however make them expert swimmers, the largest of the penguins (emperor penguins) can dive to depth in excess of 400m and hold their breath for 11 minutes. Penguins are a very successful group, with 16 species, all of which are restricted to the southern hemisphere. 5 species breed on the Antarctic continent, one of which, the emperor penguin, has the remarkable strategy of rearing their chicks during the bitter winter. [Marine mammals] Marine mammals are another groups which has terrestrial ancestors, but unlike the seabirds, some groups of marine mammals (cetacean and sirenians) have become completely independent of land, while others like seals, sea lions, and walruses and sea otters still need to leave the water to breed. Being tied to land can restrict where fur seals can go to feed, as the need to return every few days to suckle their pups (income breeding), which can deplete recourses around breeding colonies. Other pinniped species have an alternate approach requiring them to stay with the pup continuously during lactation (capitol breeding). These species far free to range widey outside the breeding season. However they need to be able to store enough energy to sustain themselves during a month long fast. Whales spend their entire lives at sea, including giving birth\ and suckling young. And their morphology reflects this being\ completely streamlined and possess no limbs other than kind flukes for propulsion and pectoral fins for stability. This means that when they become stranded onshore (which is rare) they are completely helpless and will die without human intervention. [Discussion question] List 5 iconic species of the GSR and 5 iconic Antarctic species along with 2 interesting facts/characteristics for each. Great southern reef : **Weedy Sea dragon (*Phyllopteryx taeniolatus*)\ **The weedy sea dragon is a leaf-like fish that grows up to 45cm in length and are native to the temperature waters of the southern coast of Australia. Their leaf-like appendages provide effective camouflage among seaweed and kelp.\ **Blue Groper (*Achoerodus viridis*)\ **Blue gropers can reach up to 1.2 meters in length and weigh up to 40 kilograms. They are protogynous hermaphrodites, meaning juveniles are born female and may transition to male as the mature. They inhabit rocky reefs along the southeast coast of Australia.\ **Giant Kelp (*Macrocystis pyrifera*)\ **Giant kelp is a fast-growing algae which can grow up to 60cm per day, forming underwater forests that reach up to 45 meters in height. They provide habitat and food for a diverse range of marine species, including fish, invertebrates and marine mammals.\ **Eastern Rock Lobster (*Sagmariasus verreauxi*)\ **The eastern rock lobster can grow up to 60cm in length and weight over 8 kilograms, making it one of the largest spiny lobsters. Because the eastern rock lobster is both a predator and prey, they help control populations of other marine organisms.\ **Australian Fur Seal (*Arctocephalus pusillus doriferus*)\ **The Australian fur seal can grow up to 2.3 meters in length and weight up to 360 kilograms. They are excellent swimmers capable of diving to depths of 200 meters to hunt fish and squid.\ Antarctic: **Emperor Penguin (*Aptenodytes forsteri*)\ **The emperor penguin is the largest penguin species, standing between 101 to 132cm tall. They breed during the winter with males incubating eggs on their feet for about 65 without feeding.\ **Weddell Seal (*Leptonychotes weddellii*)\ **Weddell seals are capable of diving to depths of 600 meters and remaining underwater for up to 70 minutes. They use their teeth to maintain breathing holes in sea ice, allowing easy access to the ocean for hunting.\ **Antarctic Krill (*Euphausia superba*)\ **Antarctic krill have a total biomass estimated at over 300 million tones. The krill swarm can cover vast areas, serving as a crucial food source for many Antarctic predators.\ **Antarctic Toothfish (*Dissostichus mawsoni*)\ **The Antarctic toothfish can produce antifreeze glycoproteins to prevent its blood for freezing in sub-zero waters. They can grow up to 2 meters and weigh over 100 kilograms.\. **Adélie Penguin (*Pygoscelis adeliae*)\ **There are and estimated 4 million breeding pairs of Adélie Penguin distributed across numerous colonies along the Antarctic coast. Male are known to collect and arrange small stones to form nests, which elevate and protect eggs.\ **1.2 Sharks** [The ecological role of sharks] Sharks, as a group, contain many species at or near the top of the marine food chain and as such play an important role in the ecosystem. These predators are known as 'apex predators. These large shark feed of prey from large fish to marine mammals and even sea birds. Large predators keep the populations of the prey in check and also maintain the 'fitness' of\ the prey populations by weeding out sick or weak individuals. The balance of marine ecosystems is very complex, and it is very hard to predict or measure t he effect of removing apex predators such as sharks form the ocean. However, there is some suggestion, although it is still very controversial and hotly debated, that some ecosystems are now very badly out of balance due to the removal of large shark species owing to overfishing. This is them believed to 'release' their prey populations such that their numbers increase out of control and then they impact the populations of their prey. One example of this is: once the large sharks were removed, this led to an increase in ray population size, this then results in the loss of shellfish populations that the rays feed on. [Sharks are particularly vulnerable to overfishing] Sharks are important fisheries targets throughout the world and catches are ever increasing with record catches of more than 800,00 tonnes per year, which is likely to be significantly underestimated due to non-reporting. Some 50% of the estimated global catch of sharks is takes as by-catch, which does not appear in official fishery statistics, and is almost totally unmanaged. Sharks require special resource management as they are more susceptible to overfishing than other fishes which can produce millions of eggs, most of which don't survive. Once overfished, many shark populations would take\ several decades to recover. World-wide there is a poor record of sustainability of target shark fisheries, partly due to their life history, but also due to a general lack on management strategies. [Management and research] Many sharks are known to use shallow coastal waters as shark nursery areas especially during their early life history. Identifying and understanding how these areas are used is becoming increasingly important in the global effort to rebuild declining shark populations by stablishing measures to protect these areas. In their simplest form shark nurseries are areas where young sharks are born, and juveniles spend the first years of their lives. They are often characterised as being shallow, coastal areas where young sharks have access to abundant food resources and are less vulnerable to predation from adult sharks. Shark nurseries are typically occupied by multiple shark species which would naturally suggest these species compete for habitat and food resources. Therefore, understanding how multiple sharks interact and the mechanisms that enable this to occur will ensure the ecosystem-based management decisions achieve protection for multiple shark species. [Discussion Question] What are the key characteristics of the NSW Shark management program, include a list of the technologies used? The New South Wales Shark Management Program is an initiative aimed at enhancing beachgoer safety while minimizing harm to marine life. The program was established in 2015 with a 16-million-dollar commitment and focuses on trailing and implementing innovative technologies and strategies to reduce shark interactions. The program emphasizes non-lethal methods to protect humans and marine life, moving away from traditional shark nets due to their impact on non-target species. Additionally, educational initiatives are in place to raise public awareness about shark safety and promote the use of person deterrent devices. The technologies being used include: - SMART Drumlines which capture and tag sharks, which are then relocated offshore. They have proven effective in reducing shark attacks and have been expanded to various NSW regions. - VR4G Shark Listening Stations detect tagged sharks and provide real-time alerts to authorities and the public when a shark is nearby. - Drone Surveillance monitor coastal waters for shark activity, providing real-time data to lifeguards. As of 2021, drone surveillance was conducted at 50 beaches. - Shark Nets are still used at some beaches, however, their effectiveness and impact on marine life are under review. **1.3 Origin of life** [What is life? How did it start and how do we recognise it elsewhere in the universe? ] Properties of life: - A capacity for growth and reproduction - Having metabolism - A capacity to adapt to environment [From dead molecules to living cells] In 1953, Stanley Miller's experiment simulated early Earth's conditions by combining gases (ancient atmosphere), a spark plug (lightning) and a simulated "ocean". This process produced amino acids, the building blocks of life. Subsequent experiments demonstrated the formation of proteins, and by 1993, scientist created self-replicating RNA in a lab. This supports the idea of an 'RNA world', where RNA acted as the primary genetic material before DNA emerged. For the first cells, a protective cell wall was necessary, forming a prokaryote cell template. These primitive cells lacked mitochondria, chloroplasts, and a nucleus. Early life thrived without oxygen, using gases like ammonia and hydrogen\ to generate energy and convert CO₂ into organic compounds. [The rise of cyanobacteria and photosynthesis] There are archaea (ancient; sometimes wrongly called archaebacteria -- although they are 'bacteria-like') existing today similar to those original primitive organisms -- super thermophilic (heat loving) cells that can produce methane from CO₂ in environments without oxygen. It needs only a small chemical transformation to turn the metabolic activities of\ the archaea into the photosynthetic machinery of the cyanobacteria, which use carbon dioxide, water, and energy from light toe produce organic molecules and release oxygen. Fossil cyanobacteria are known from western Australian sediments where they produce structures called stromatolites, some 3.5 billion years old. [Eukaryotic radiation] Eukaryote cell types, represented by all plants, animals, fungi, algae, and single celled protists are much more complex and evolved than 2.1 billion years ago. Through molecular detective work on individual cell components, we now know that they in fact represents permanent symbiotic association of different creatures living together. A primitive amoeba-like predator ingested a sulphur bacterium that became a mitochondrion, ingested a cyanobacterium that became a chloroplast, and so on. It has now been fully substantiated by sophisticated molecular technologies. Many primitive plant-like algae and animal-like protozoa actively practice this way of living still today, as in image 1.3.4. [The tree of life] Being large beings ourselves, we tend to focus on macroscopic creatures, the one we see from the naked eye, and so we lose sight of the crucial importance of microbes. Most of the genetic diversity on our planet still resides in the kingdoms of the true bacteria, archaebacteria and protists that dominate the world oceans. Genetic diversity of life in the water which started to evolve 3.5 billion\ years ago remain much larger than the land animals and plants.  [The microbial engine] If you take all the microscopic algal cells in the world oceans you can pack them together in a 7cm deep and 30cm wide plank stretching from the earth to the moon. This is impressive considering the algae clean the atmosphere from the greenhouse gas CO₂ and deliver humans with oxygen. The oxygen in every second breath we take comes from the phytoplankton. More importantly, microscopic algae grow much faster than land plants, on average they divide once per day. That means they deliver us and extra plank to the moon every day. The reason that the oceans are not getting greener every day is because this plank in its entirety is consumed by zooplankton, the free-floating animals and larval fish. Small as these early life forms are, they represent the microbial engine that drives our planet. [Discussion Question] Discuss the evidence supporting and challenging the notion that life evolved in the seas. Debate rages between biologists and chemists over whether life began on land or under the sea. The question 'How did life begin?' is closely linked to the question 'Where did life begin?' Most experts agree over 'when': 3.8--4 billion years ago. However, there is still no agreement about which environment started this event. Since their discovery, deep sea hydrothermal vents have been suggested as the birthplace of life, particularly alkaline vents. But not everyone is convinced that life started in the sea -- many say the chemistry just won't work and are looking for a land-based birthplace. In 1993, before alkaline vents were actually discovered, geochemist Michael Russell from Nasa's Jet Propulsion Laboratory (JPL) in California, US, suggested a mechanism by which life could have started at such vents. His ideas, updated in 2003, suggest life came from harnessing the energy gradients that exist when alkaline vent water mixes with more acidic seawater. This mirrors the way that cells harness energy. Russell's theory suggests that pores in the hydrothermal vent chimneys provided templates for cells. This energy, along with catalytic iron nickel sulphide minerals, allowed the reduction of carbon dioxide and production of organic molecules, then self-replicating molecules, and eventually true cells with their own membranes. But not everyone agrees that life began in deep sea hydrothermal systems. Armen Mulkidjanian at the University of Osnabruck in Germany says there are several big problems with the idea, one being the relative sodium and potassium ion concentrations found in seawater compared to cells. He says therefore it makes no sense for cells that contain 10 times more potassium than sodium to have their origins in seawater, which has 40 times more sodium than potassium. His assumption is that protocells must have evolved in an environment with more potassium than sodium, only developing ion pumps to remove unwanted sodium when their environment changed. **1.4 Food webs** [Definition] Most ecosystems on earth are powered by the sun, with solar energy enabling phytoplankton to grow by combining nutrients under the right conditions. These microscopic plants form the base of the marine food web, supporting like at higher trophic levels. Zooplankton, the primary consumers, feed on phytoplankton, while larger animals consume plants or plant-eating animals. Energy transfer through the food web is inefficient, with only about 10% of consumed energy contributing to growth at each trophic level, resulting in a food pyramid structure. This inefficiency emphasizes the importance of primary producers. Food webs can range from simple to complex, often relying on key organisms that are critical for energy flow. In some ecosystems, these pivotal organisms create a 'wasp's waist', where energy flow narrows significantly. [Alteration to food webs] Food webs have evolved with the evolution of their organisms. Animals have adapted to the availability of new food sources and to the loss of their existing food sources. Changes like this can occur over very long-time scales which allow animals to slowly adapt. Sometimes, changes can be abrupt. They can be natural or manmade changes. Animals often migrate long distances to maintain their food supplies. All changes to the marine environment that impacts the availability of food though altering the distribution, abundance or availability of organisms will alter marine food webs. These changes can result from pollution, fishing, climate change or other means. [Fishing the marine food webs] Fishing is a controversial factor in altering marine food webs. It directly removes marine organisms, some of which are discarded if not valuable, while others are highly sought after. Fishing also indirectly impacts ecosystems by altering habitats, such as damaging the seafloor through trawling. The concept of \"fishing down the marine food web\" describes a process where humans first target apex predators (e.g., tuna and sharks) at the top of the food chain. These predators are especially vulnerable due to their longer lifespans and lower reproductive rates. As their populations decline, two effects occur: prey species of these predators may thrive and increase in number, and fishing efforts may shift to these now-abundant species. Over time, this process could deplete the ecosystem to a point where only plant eaters remain. However, there are exceptions to this pattern. Fishing does not always target apex predators first; instead, it often focuses on species that are more valuable or preferred for consumption, such as herring, which are lower on the food web. Additionally, modern fishing practices increasingly\ target a wider range of species, a process called \"fishing through the food web.\" Understanding how marine food webs function, respond to stress, and adapt to human impacts is critical. Changes to one species can cascade through the web, affecting numerous others, emphasizing the interconnected nature of marine ecosystems and the importance of sustainable fishing practices. [Discussion Question] List the trophic level of the 10 species you listed in Module 1.1. Weedy Sea Dragon (*Phyllopteryx taeniolatus*) -- **secondary consumer**: small crustaceans e.g. mysids\ Blue Groper (*Achoerodus viridis*) -- **secondary/tertiary consumer**: crustaceans, molluscs, echinoderms\ Giant Kelp (*Macrocystis pyrifera*) -- **primary producer**: photosynthesizes to produce energy\ Eastern Rock Lobster (*Sagmariasus verreauxi*) -- **secondary consumer**: small invertebrates\ Australian Fur Seal (*Arctocephalus pusillus doriferus*) -- **tertiary consumer**: fish, squid, ect. Emperor Penguin (*Aptenodytes forsteri*) -- **secondary/tertiary consumer**: fish, krill, squid\ Weddell Seal (*Leptonychotes weddellii*) -- **tertiary consumer**: squid, fish, ect.\ Antarctic Krill (*Euphausia superba*) -- **primary consumer**: phytoplankton, algae\ Antarctic Toothfish (*Dissostichus mawsoni*) -- **tertiary consumer**: fish, squid, ect.\ Adélie Penguin (*Pygoscelis adeliae*) -- **secondary/tertiary consumer**: krill, fish, small squid **1.5 Antarctic ecosystems** [Life in antarctica] Living outside the ideal temperature range for life requires very specialised adaptations, and life in the regimes is restricted largely to bacteria. AT the poles, due to the tilt of the earth and low angle of the sun, temperatures are much colder than the rest of the planet and are often below zero -- the coldest place on earth is Vostok station in antarctica. This has profound implications for the types of plants and animals that can live there. The lack of liquid water means that, with the exception of a few highly adapted mosses and fungi, bacteria and invertebrates, there is no life in Antarctica that does not rely on the ocean. These organisms need to contend with water changing form its liquid phase to its solid phase ona. Regular basis, and this is one of the reasons that polar regions are particularly sensitive to climate change. [The Arctic is an ocean surrounded by land and Antarctica is land surrounded by the ocean] One of the key differences between the arctic and the Antarctic likes in the geological history, which has resulted in the arctic being and ocean largely surrounded by land, while antarctica can be regarded as a land mass surrounded by ocean. Antarctica is therefore the only\ continental land mass on earth which has a completely uninterrupted series of currents circulation around it, ultimately influencing the environment and the ability of organisms to migrate in and out of the Antarctic ocean. In addition to this there is a massive sheet of frozen ocean that expands and contracts annually, which at its peak in the winter occupies and area of 15 million square kilometres -- twice the area of Australia. [Cold climate = low biodiversity] The combination of the harsh climate and geographical isolation means that the Antarctic has much lower diversity than most other marine ecosystems, as the only organisms that can live there need to be highly specialised and there are relatively few ecological niches available. This is due to the fact that all but 1% of the continent is covered by ice, and permanent and seasonal sea-ice covers much of the ocean. The ecosystem of the southern ocean is correspondingly one of the simplest of all marine ecosystems. However, those species that can live there often prosper and can be spectacularly abundant. For example, crab-eater seals are the most abundant of all seal species in the world. Despite the relative simplicity, the ecosystem is still poorly understood by scientists -- due, in a large part, to how difficult it is to get to antarctica. [Productivity highly varies between seasons] One important feature of the ecosystem is the highly seasonal nature of its productivity. The high latitude of antarctica means that, not only is it extremely cold, but it also experiences many months of complete darkness in the winter months where there is little or no production of phytoplankton (the dominant plants in the southern ocean). Conversely, in summer months the region has 24 hours of daylight, which when combined with increasing summer temperatures and the retreat of the winter sea-ice, results in massive blooms of phytoplankton, so that, at least for a few months, the southern ocean is the most productive on earth. This productivity forms the base of the food web, which is geared around exploiting this abundant, but seasonal resource. [The Antarctic krill is the most important species in the Southern Ocean Ecosystem ] Another important feature of the ecosystem is the importance of a single species, the Antarctic krill (Euphausia superba). Krill are sometimes said to be the most abundant organisms on earth with a standing stock of 500 million tonnes. While the veracity of that statement can be debated, it is indisputable that this one species plays a central role in the southern ocean ecosystem. In oceanic regions krill are a major consumer of primary production\ (phytoplankton), and themselves are food for fish, squid, seabirds, and marine animals. [Antarctica has become a hunting ground affecting the whole ecosystem ] Despite commonly being described as the "last pristine wilderness of earth", from an ecological point of view nothing could be further from the truth. After its discovery in the 17^th^ century, the region became that target of intensive commercial hunting which firstly took millions of fur seals, resulting in their near extinction, and then whaling which removed a significant proportion of the whales from the southern ocean. The removal of fur seals and whales over a hundred years ago has had enormous repercussions for this simple ecosystem, the effects of which still\ need to be considered by scientist and policy makers today. [Discussion Question] Describe the habitat and environmental conditions experienced by the organisms covered in the links (plants/microbes, benthic invertebrates, Adelie penguins). What environmental changes might be occurring across these habitats and how might scientists assess any impacts on the associated species? The cold habitats of Antarctica host diverse organisms, from tiny microbes to large marine mammals, each uniquely adapted to withstand harsh condition. However, environmental changes are posing serious challenges. **Plants and microbes** such as mosses and lichens, thrive in nutrient-poor soils and extreme cold. However, rising temperatures and melting ice are altering their habitats. Scientists study soils samples and DNA analysis to track the impacts on plants and microbes. **Benthic invertebrates** such as sea stars and molluscs live on the seas floor and rely on stable oxygen levels and cold waters. Unfortunately, ocean acidification and warming are changing these ecosystems, which are monitored through sediment analysis. **Adelie penguins** depend on sea ice for breeding and krill for food. Climate change is depleting sea ice and disrupting their habitat and food supply. Scientists use satellite tracking and population studies to assess the impacts. **1.6 Temperate kelp forests** [What is kelp] Kelps belong to the class Phaeophyceae (brown algae) and are strictly defined as algae from the order Laminariales. However, the term "kelp" is increasingly used for all large, canopy-forming brown seaweeds. Most true kelps belong to the genus Laminaria, with their greatest diversity in the northern hemisphere, where they evolved 15-35 million years ago. Only two species of Laminaria are found in the southern hemisphere, with limited\ distribution. In contrast the southern hemisphere has a high diversity of canopy-forming fucoids (order Fucales), which form prominent intertidal and subtidal forests, such as durvillaea in Tasmania. In the northern hemisphere, fucoids primarily form intertidal canopies and are less significant subtidally. Kelp beds generally develop on shallow rock reeds in warm and cool temperate regions, typically between 35° and 60° latitude, though there are some exceptions. [Structure of kelp forests] Like their terrestrial counterparts, kelp forests usually have a complex structure, often with several layers of sub-canopy and understorey species beneath the top canopy. When disturbances occur to open up holes in the canopy, the species that colonises that gap usually depends on which species are reproductive -- that is, which species is producing spores -- and close by at the time the gap appears. Because different seaweeds are reproductive at different times, and tend to be patchily distributed in space, many kelp forest exist as a dynamic mosaic of patches reflecting the space-time patchiness of disturbances. [Productivity] Kelp beds are highly productive in optimal conditions with abundant light and nutrients, often provided by upwelling. Giant kelp, the fastest-growing species, can grow 35-45cm per day under these conditions, reaching lengths of over 30m. Kelp beds can produce over 2kg of carbon per square meter annually, surpassing even the most intensively managed crops. Unlike coral reefs, where most algae are consumed by grazers, only a small portion of kelp production is directly eaten. Instead, most becomes detritus (waste) or dissolved organic matter, supporting bacteria and detritivorous animals like sea squirts, sponges, and mussels. The structural complexity of kelp forest also fosters high secondary production, supporting fisheries for species like finfish, abalone, and lobsters. [Sea urchins] Sea urchin outbreaks can devastate kelp forests by overgrazing seaweed, transforming reefs into barren habitats devoid of macroalgae and many benthic invertebrates. These "urchin barrens" result in significant losses of biodiversity, productivity, and fisheries compared to thriving kelp beds. Urchins however can survive in these barrens by feeding on microalgae, drift seaweed, or resistant calcareous algae, meaning their populations persist even after the\ seaweed is gone. Restoring kelp requires reducing urchin densities to much lower levels than those that initially caused the barren -- virtually eliminating them. This makes urchin barrens a significant challenge for marine management, as seen in eastern Tasmania, where extensive barren areas have replaced once-divers kelp forests. [Why do urchin barrens form?] Evidence indicates that overfishing of urchin predators enables the urchins to build up to the point where destructive grazing can commence. Fishing of the urchin predators sets up a so-called trophic cascade... Human fishing activity **increases\ **Urchin predators **decrease**\ Urchin populations **increase\ **Kelp and other seaweeds **decrease** The classic example is hunting down sea otters (for their fur) as a 'keystone predator' of urchins in the NE pacific rim, leading to urchin outbreaks, destruction of kelp and barrens formation, and the recovery of kelp when otters were reintroduced to areas from which they'd effectively been exterminated. But urchin barrens are known from all temperate waters -- for example, Australia and NZ, Japan, Chile, Scandinavian countries, and both sides of the north Atlantic -- and in these places it tends to be fish and/or lobsters that are overfished to trigger barrens formation. [Discussion Question] What are key threats facing seaweeds on the Great Southern Reef? What is the scale of these stressors? Seaweeds on the Great Southern reef face stressors such as climate change and sea urchin outbreaks. Climate change is causing rising sea temperatures which threated the reef's seaweed populations. For example, a 2011 marine heatwave off southwestern Australia resulted in a large loss of several seaweed species, many of which are still struggling to recover. Prolonged warming stress seaweed, reducing their ability to grow and reproduce. Overfishing of predators, such as lobsters, has allowed sea urchin populations to grow unchecked. Sea urchins are ravenous grazers capable of stripping reefs of their seaweed, transforming them into urchin barrens devoid of macroalgae and biodiversity. Once a barren is established, sea urchins continue inhabiting the area by feeding of microalgae and drift seaweed, making recovery of kelp forests challenging. The combination of ocean warning and urchin barrens creates a positive feedback loop which accelerates the delice of seaweed habitats. **1.7 Deep sea (factoids)** [The coelacanth, the living fossil] The coelacanth has many features of extinct fish species and is more closely related to lungfish and reptiles than modern fish with fins. All of its relatives are extinct; in fact, they all went extinct in the Devonian era (\~400 milling years ago). They have thick armoured scales on their body. For a long time, they were thought to be extinct also, and then a skipper who had been trawling in south Africa reported an unusual fish amongst his catch in 1938. A second species was discovered in 1999 in Sulawesi Indonesia when a marine biologist spotted one at a fish market and photographed it but couldn't convince the fisherman to sell it to them. They are an extraordinary fish for a number of reasons. One has never been brought up to the surface alive. The live in the\ deep ocean at about 100-500m down in caves where they rest\ all day, and drift out at night to feed on fish and squid. The have huge eggs inside their bodies, unlike the modern fish which lay eggs or release them into the water. They are about the size of oranges. The eggs hatch inside the female and have a very large yolk sac attached which is it sources of nutrition (ovoviviparous) and then the female gives live birth. Coelacanths are about the size of a human at 170cm long and 60kg in weight, and live until they are over 100. They do not taste good as their body has a foul-tasing urea and oil. But they are still at risk of extinction because they are accidently caught when fishers\ target oilfish. This combined with their longevity and slow reproduction makes them highly vulnerable.  [The giant squid remains a mystery for scientist] The giant squid was one of the most famous mythical sea monsters until 2004 when it was photographed for the first time, alive and in its natural environment. Then again, in 2006 it was filmed by the same team of scientists. Before 2004 the giant squid was never seen alive but dead and washed ashore. The giant squid still remains a mystery for scientists.  In January 2013, a group of three scientist captured a giant squid on camera using a remotely operated vehicle (ROV). Instead of using bait, they used and optical lure, a battery-powered camera attached to a floating line.\ This "electronic jellyfish" (e-jelly) emits red light visible to deep-sea animals and mimics the bioluminescence of the Atolla jellyfish. The jellyfish produces light when chewed on by a\ predator, hoping to attract a larger predator to attack to\ one that is threating it. [Scientists use information locked in ear bones to figure where giant squid live and some other facts] We still don't know much about the giant squid, such as its life cycle, how long it lives, and how fast it grows. Video footage can only tell us so much. However, by studying the ear bones (statoliths) we can find out how deep giant squid actually live. Just like you can date the time at which tree rings were formed, scientists can determine the age of the squid and the temperature of the ocean water by looking at growth rings in statoliths. Statoliths are tiny and are roughly the size of a pinhead. The temperature\ deduced from the growth rings (1 ring = 1 day) are matched with the ocean depth\ (in general -- the colder the temperature the deeper the corresponding depth). [Discussion Question] Explain how/what resources drive food webs in the deep sea. In the deep sea, food webs are driven by resources the originate from the surface and by unique deep sea processes. A key resource is "marine snow", which consists of organic material such as dead plankton, faecal pellets, and other organic debris that slowly sinks from the upper layers of the ocean to the deep sea. This material serves as a vital food source for deep sea organisms, ranging from bacteria to larger animals like fish and invertebrates. These resources are essential as the deep sea is an environment where sunlight is absent. Another important driver of deep sea food webs is chemosynthesis, which occurs near hydrothermal vents. This is where bacteria use chemicals from the vent emissions to produce energy, rather than relying on sunlight. These bacteria form the base of the food web, supporting a wide range or organisms like tube worms and clams that are adapted to life in these extreme environments. **2.1 Oceanography** [Ocean modelling] Physical oceanographers use mathematical models to describe ocean mixing and circulation, helping to understand both short-term processes (like coastal system responses to wind changes) and larger-scale issues, such as the oceans' role in regulation and responding to global climate change. Advances in computing power and ocean theory have greatly improved these models in recent years. A key focus of current research is enhancing the resolution of these models, such as simulating ocean eddies, and integrating them with biological cycling equation to predict how the ocean biosphere will respond to climate change. However, biological processes are mostly observed through traditional sampling methods, highlighting the importance of combining observations with models. [Networking] Networking with other scientists at nation and international meetings offer and opportunity to get the word out about out science and to develop new and exciting collaborations. [Discussion Question] What are aerosols and what role to they have in climate? Aerosols are tiny particles suspended in the atmosphere. They can come from natural sources like sea spray, volcanic eruption, and wildfires, or from industrial processes and burning fossil fuels. Aerosols have an important role in climate because they have to ability to eighter cool or warm the Earth, depending on their behaviour. Some aerosols, such as sulphate particles, reflect sunlight back into space, leading to a cooling effect on the climate. However, others such as black carbon, also known as soot, absorb sunlight and can contribute to warming.. **2.2 Primary producers and nutrients** [Ocean photosynthesis] Just like photosynthesis on land, photosynthesis in the ocean is performed by organisms that contain chlorophyll. However, the plants of the ocean are mostly single celled and microscopic. They are the phytoplankton which means "drifting plant". While it is true that kelps and seagrasses also photosynthesis, they are really just the bath ring around the vast oceans that cover 70% of the planet. These tiny plants have a huge impact on global climate. They are also the base of the marine food chain, supporting the fisheries that feed us. The phytoplankton are small (\~1-10 microns), they are ubiquitous (present everywhere) through the oceans. The phytoplankton have become and increasingly diverse group, spanning thousands of species. As you can see in image 2.2.2 some have adapted to the extremely low nutrient concentrations of the ocean deserts -- the subtropical gyres -- and others more specialised to regions of high nutrients, like the costal upwelling systems of Peru and elsewhere. [Unique aspects in the marine environment] Just like photosynthesis on land, the phytoplankton require light and nutrients, fertilisers such as nitrogen and phosphorous, and trace elements like iron. The problem for the phytoplankton is that light is abundant near the surface and decreases exponentially with depth, because it is absorbed by water particles including they plankton themselves. Conversely, nutrients are low near the surface but increase in the darker, deeper parts of the ocean. This patter occurs precisely because the phytoplankton consume nutrients near the surface in well-lit conditions, and as they sink out and die, they decompose back into the dissolved nutrients they came from. So, we only see high ocean productivity where these two resources occur together. [Global distributions, seasonal and interannual variability ] Ocean productivity is influenced by both physical processes that bring light and nutrients together. One classic example is the North Atlantic spring bloom, where phytoplankton grow rapidly due to increased sunlight and nutrients after winter mixing. This bloom, one of the largest biological phenomena of Earth, occurs as the ocean warms and less mixing allows the nutrients to rise, fuelling phytoplankton growth. After a period, the bloom declines as nutrients are exhausted or consumed by grazers like copepods, which support fisheries in the region. Another key area of high ocean productivity is coastal upwelling systems, such as those along the west coasts of the Americas and Africa. Winds and Earth's rotation create offshore\ currents, which are replaced by nutrient-rich, cool waters that trigger blooms in the sunlit zone.\ These blooms are essential for sustaining large, ecologically and economically significant fisheries. Variability in mixing and circulation, influenced by factors like El Niño and climate change, affect productivity in both seasonal blooms and upwelling systems. [Conclusion] ! These microscopic plants produce half the oxygen we breath !\ ! They are dependent on light that penetrates into the ocean and on nutrient or food !\ ! They are not distributed evenly, and their concentration vary seasonally! [Discussion Question] How can scientists predict hotspots of seafloor biodiversity? To identify seafloor biodiversity hotspots scientists, use a variety of methods. Studying environmental characteristics such as water temperature, depth, salinity, and nutrient availability is one approach since these elements have a significant impact on the kinds of organisms that can thrive in a location. For example, regions with complex ecosystems, such as seamounts, deep-sea vents, or coral reefs, have higher biodiversity than say deep sea habitats. Data from remote sensing technology is used by scientists to learn more about these environmental conditions and locate possible hotspots for biodiversity. **2.3 Pumping carbon -- ocean's role in climate** [The ocean regulated the amount of CO₂ in the atmosphere] The ocean plays a key role in regulating that amount of carbon dioxide (CO₂) in our atmosphere. As CO₂ is a powerful greenhouse gas, meaning it greatly affects the temperature of the earth, this is one of the ways that the ocean regulates our climate. The reason the ocean is so effective at controlling atmospheric CO₂ is because it contains a lot of carbon, particularly carbon that can exchange rapidly. The ocean contains roughly 60 times more carbon than the atmosphere, and 15 times more carbon than all land plants and soils combined. Geological reservoirs of carbon are large, but exchange rates are very small. They scale of human alteration on the global carbon cycle is significant: for example, fossil fuel emissions are over 10 times greater than the amount of carbon buried in ocean sediments annually. The carbon in the ocean is NOT the whales, fish, penguins or even the shrimp. It's mostly dissolved, inorganic carbon (DIC). This is in contrast to the land carbon, which is mostly living in biomass like trees. The deep ocean contains more DIC than the surface ocean, which can exchange carbon with the atmosphere. Two processes move carbon to the deep sea: the biological pump, where CO₂ is converted into organic matter that sinks, and the solubility pump, where CO₂ is absorbed by cold water and carried to the deep. These pumps help regulate atmospheric CO₂ levels, with the biological pump playing a crucial role in keeping CO₂ levels lower than they would be otherwise. [The ocean has absorbed \~30% of anthropogenic CO2 emissions, and this has acidified the ocean ] Anthropogenic CO₂, primarily from fossil fuel and deforestation, is a significant driver of climate change. The ocean plays a crucial role in mitigating some of the impacts of this CO₂ by absorbing about a quarter of the total emissions produced by human activities. The CO₂ absorbed by the ocean leads to a phenomenon call ocean acidification, where\ the pH of seawater decreases due to chemical reactions between CO₂ and water. As a result, the concentration of hydrogen ions increases, lowering the pH level. Currently, the surface ocean's pH is around 8, but it has already dropped by 0.1 units, and scientists predict thus could increase by 100-150% by\ 2100. The decrease in pH has serious consequences for marine organisms, particularly those that rely on calcium carbonate to build shells and skeletons, such as mussels, oyster, corals, and some types of phytoplankton. The reduced availability of carbonate ions makes it more\ difficult for these organisms to maintain their shells, potentially leading to disruptions in marine ecosystems. In contrast, non-calcifying organisms like seaweed and algae benefit from higher CO₂ levels, similar to land plants. This shift in marine species could disrupt food chains, impacting human food supplies, marine biodiversity, and coastal habitats. Additionally, ocean acidification can weaker the ability of coral reeds to protect coastlines from storms and may have a negative effect of tourism, which often relies on\ health marine environments. [Discussion Question] What are PIPs and how do they affect the movement of carbon in the oceans? PIPs, also known as particle injection pumps, are mechanisms that improve the ocean's capacity to sequester carbon by facilitating the transfer of carbon from the surface of the ocean to the deep ocean. This process is a vital component of the biological carbon pump, which plays a significant role in regulating atmospheric CO₂ levels. PIPs operate through various biological processes, like the sinking of organic matter produced by phytoplankton and the vertical migration of zooplankton. These activities transport carbon rich particles from the ocean's surface to the deeper layers, effectively removing CO₂ from the atmosphere and storing it in the deep ocean. **2.4 The iron age of oceanography** [Iron and its productivity] Over the last 15 years, research has shown that productivity in about a third of the ocean is limited by the availably of iron. Chlorophyll concentration maps indicate that regions with low iron often have low chlorophyll, signalling low productivity. The biological pump's role in controlling atmosphere carbon dioxide\ highlights the importance on iron in marine ecosystems, yet iron is found in very low concentrations in most ocean waters. In addition to iron, nitrate, a common nitrogen that forms in the ocean, also plays a role in marine productivity. However, areas like the North Pacific and Southern Ocean have high nitrate but low chlorophyll, referred to as the high nutrient low chlorophyll (HNLC) regions. This discrepancy was initially unexplained, with early hypotheses focusing on light or predator control. Despite iron being abundant in Earth's crust, its concentration in\ \ seawater is very low -- about 0.1 nano-moles per litre. Early studies dismissed iron as a limiting factor but using clean sample collection methods in the 1970s lead to renewed interest in iron's role. Subsequent experiments confirmed that adding iron to HNLC water significantly boosts phytoplankton growth. [Climate implications of iron limitation] John Martin's iron fertilization experiments led him to propose a link between iron and climate, particularly in relation to atmospheric CO₂. Ice core data from Antarctica showed that CO₂ concentration fluctuated with glacial cycles, being lower during glacial period. Martin suggested that during glaciation, more iron reached the ocean from land, alleviating iron limitation for phytoplankton and stimulating the biological pump, which in turn removed CO₂ from the atmosphere. Scientists continue to explore its effectiveness and ecological impact, while policy experts debate the ethics and legal aspects. Recent research, however, suggests that\ while iron fertilization may have played a role in glacial periods, it alone is not sufficient to explain the significant CO₂ drop during those times. [Discussion Question] How is iron delivered to the ocean around the sub-Antarctic islands? And what is its effect on the biology? Iron is delivered to the ocean around the sub-Antarctic islands through several natural processes, including atmospheric dust deposition, upwelling and melting icebergs. Winds from regions like southern South America blow iron rich dust into the ocean, while upwelling brings iron from deep waters to the surface. Additionally, when icebergs melt, they release trapped iron into the surrounding water. This added iron plays a crucial role in simulation phytoplankton growth, as iron is often a limited nutrient in many parts of the ocean. When iron is introduced, it triggers phytoplankton blooms. This then enhances marine food webs and helps to support ecosystem health. **2.5 Ocean observations with satellites, robots and animals** [Satellites] Recent advancements in technology, such as satellites and ocean robots, have significantly improved our ability to observe and understand the oceans. Observing the oceans is challenging due to its vast, dark, and three-dimensional nature, and instruments must be protected from water, salt, pressure, and temperature extremes. Despite this, marine observations have historically been prone to equipment losses at sea, making it difficult to fully understand the deep sea compared to other environments like Mars. Technologies like satellites, robotic platforms, buoys, and tags are now\ essential for studying ocean variability. Satellites, used since the late 1970s,\ can monitor ocean conditions like surface temperature, seasonal changes, ocean currents, and ecosystems. New satellite sensors can even estimate phytoplankton abundance and species based on ocean colour. These tools measure various parameters, including wind speed, salinity, and sea surface height, with the goal of monitoring long-term climate changes, such as sea level rise and ocean temperature fluctuations. As the time series for ocean chlorophyll data grows, scientists can now begin to detect the impact of physical changes on ocean biology. [Undersea robots (and animals)] Satellites offer real-time observations of the ocean's surface, but much of the ocean's variability occurs beneath the surface. Traditionally, deep ocean observation required expensive ship-based sampling, but advances in robotic platforms have revolutionised this. The Argo program, for example, used over 3,00 autonomous floats that collect vertical profiles of temperature and salinity up to 2,000m deep every 9 days. This system provides real-time data and has significantly increased the amount of data collected, especially in remote regions like the Southern Ocean. In addition to robots, satellite tags are now used to track marine mammals and gather oceanographic data. These tags can measure temperature, salinity, chlorophyll, and light, providing valuable insights into marine ecology and ocean conditions in places where Argo floats can't reach, such as beneath the Antarctic ice. The next frontier in ocean research involves expanding the range of\ chemical and biological sensors deployed on the automated platforms to monitor ocean biogeochemistry with unprecedented precision. [Discussion Question] What are the benefits of using AUVs to collect data around Antarctic ice sheets compared to ship-board monitoring? Summarise key technologies used by IMOS to monitor the marine environment. Using autonomous underwater vehicles (AUVs) to collect data around Antarctic ice sheets offers significant advantages over traditional ship-based monitoring. AUVs are able to access hard to reach areas, such as the underside of ice shelves. They operate autonomously, which reduces risks to human researchers in Antarctica's harsh environment. AUVs also deliver continuous, high resolution data on temperature, salinity, and currents, which is valuable for studying oceanographic processes. Also, they have a smaller environmental footprint than large ships and are more cost-effective in the long term, as they require less fuel. The integrated marine observing system (IMOS) uses a range a technologies to monitor the marine environment around Australia. One key tool is ocean gliders, which are AUVs that collect data on temperature, salinity, and ocean currents. IMOS also used Argo floats, which drift with ocean currents and dive to different depths to measure temperature and salinity profiles. For coastal monitoring, radar systems track surface currents, while acoustic technologies like passive acoustic sensors are used to study marine life and monitor human impacts. **2.6 Ocean circulation** [Ocean currents role in weather and climate] The oceans have an enormous heat capacity and hence can slow the rate of climate change. For instance, the upper 3 meters of the ocean holds as much heat as the entire atmosphere. Ocean currents can transport and redistribute heat to deeper ocean layers where it can reside for centuries. The ocean stores and transports not only heat but also carbon dioxide (CO₂), a potential major source of global warming. About half of the total CO₂ added to the atmosphere during the past century by human activity has been absorbed by the ocean. Surface currents redistribute heat around the world and have a profound effect on the world's climate. It is especially clear in the North Atlantic Ocean where the Gulf Stream carries huge volumes of warm salty tropical water north to the Greenland coast and to the Nordic Seas. Heat radiating off this water helps keep the countries of northwest Europe relatively comfortable places to live. [What are currents?] Ocean currents are present in every ocean basin and vary in size, strength, and importance. Some of the strongest currents are the Gulf Stream in the Atlantic, the Kuroshio and East Australian Current in the Pacific, and the Antarctic Circumpolar Current in the Southern Ocean. [Surface currents] Surface currents are found in the upper few hundred meters of the ocean. They are mostly caused by the wind applying stress to the water as it blows over the surface of the ocean. Because surface currents travel slowly compared to the Earth's rotation and over long distances, the rotation of the Earth plays an important role in their movement. The action of wind and Earth's rotation cause the water to move in a circular pattern, called gyres, with fast currents at the western boundaries of the oceans and slow currents in the interior away from the boundaries. In the northern hemisphere, gyres move clockwise and in the southern hemisphere they spin counterclockwise. These wind-driven gyres dominate the central regions of most ocean basins. [Deep-water currents] Deep-water currents are found in the bottom few kilometre of the ocean and make up about 90% of the ocean. These currents are mainly cause by density differences in the water. Because the density of water in the ocean is determined by its temperature and salinity, this circulation is also called the thermohaline circulation (thermos = temperature; haline = salinity). Cold, salty waters are dense and sink to the bottom of the ocean at high latitudes, while warm waters are less dense and rise to the surface throughout the ocean interior. The circulation of deep, dense waters acts as submarine river and moves water throughout the ocean. The ocean conveyor "starts" in the North Atlantic, where warm waters form the Gulf Stream lose their heat to the cold atmosphere. This loss of heat makes that waters cooler and denser, causing them to sink to the bottom of the ocean. [Global conveyor belt] There is an underlying transport pattern emerging from the complex circulation of both surface and deep-water currents. Water cycles form the surface of the ocean to the abyss then back to the surface again. This global circulation is similar to a giant conveyor belt and is called the meridional overturning circulation. It takes almost 1,00 years for the conveyor belt to complete one "cycle". **2.7 Ocean eddies, waves and mixing** [Eddies] Ocean eddies are everywhere in the ocean. They form when the large-scale currents become unstable. Eddies are one of the ways the ocean can take energy from the large-scale currents and move it to smaller scales where it is used to mix the ocean. Ocean mixing allows the warming and cooling at the sea surface to be distributed throughout the ocean depth. [Waves] Waves are another way energy is transferred to small scales to produce mixing. As a surface wave rolls into the surf zone it becomes steeper as the water becomes shallower. As soon as the water depth is less than half the wavelength of the wave (distance from one crest to the next), the wave starts to feel the ocean bottom, and it steepens, slows down, and the wavelength get smaller. Eventually, the wave becomes so steep that it is unstable and breaks, crashing onto the shore. Waves can also break in deep water when the wind is strong enough to build the height of the wave until the become unstable. Although we see surface waves move, the water itself is going in circles. The water is lifted up and pushed forward a little as the crest passes and falls down and is pulled back a little as the trough passes. The circles are larger at the sea surface and gradually get smaller the deep er you go, until there is no wave motion at all. The Southern Ocean is the place with the highest waves. This is because the strong westerly winds (the roaring forties, and furious fifties) blow continuously around the globe, uninterrupted by land, allowing the waves to grow very high. [Internal waves and mixing] Waves form on an interface or boundary. The interface between the air and the sea is where surface waves form. Although we can't see them, there are also many waves below the sea surface. There are called "internal waves", internal to the ocean. They form on the interface between layers of different density. Internal waves and eddies are important to climate because they remove energy that is added to the ocean by winds. As a wave breaks it is releasing energy and mixing the ocean. The waves grow, become unstable and break. This releases energy (seen in yellow) and mixes the ocean. Note there's less energy (shown in black) released from internal waves as you move towards the surface of the ocean. [Tsunamis] Waves can be formed in several ways: by meteorological forcing such as wind and air pressure changes, by tides and by earthquakes. A tsunami is a wave formed by an earthquake under the ocean. The sea floor is pushed up by the earthquake, which in turn pushes up the water above it. The bump that is formed in the sea surface radiates away from the centre, in the same way that a stone dropped in a pond makes a circle of waves. In the middle of the oceans, the bump in the sea surface is only centimetres high and can't be seen. The trouble comes when the tsunami moves toward the coast. As the water depth gets shallower, the wave feels bottom, slows down, and gets higher and steeper. Eventually the wave crashes onto the shore as a giant wall of water devastating anything in its path [Tides] The gravitational attraction from the sun and the moon both pull on the earth and make the oceans bulge toward them. The earth rotated one full circle every day, but the moon takes a month to circle the earth. This means that the bulge of water stays nearly still, which the Earth rotates beneath is. On Earth is feels like the Earth stays still while the tides come and go once or twice a day. The moon as a much stronger effect on our tides because it is much closer than the sun. When the sun and moon are in line and pulling in the same direction, we get big spring tides. **2.8 Plate tectonics: shaping oceans** [Plate tectonics] Plate tectonic theory describes the formation, destruction, movement and interaction of the Earth's surface. Plate tectonics is the process that moves continents across the Earth over millions of years. As the continents move apart they form ocean basins, and where they collide they form mountain ranges such as the Himalayas and the Andes. Plate tectonic is important because the motions are responsible for the formation of the landscapes we see all around us. [Texture of the seafloor] These motions are also responsible for creating the texture of the seafloor that is important for controlling deep ocean mixing. Oceanic crust is quite different from continental crust. It is denser, thinner and get completely recycled approximately every 200 million years as ocean basins open and close. There are massive mountain chains that extend through all the world's\ major ocean basins. This is where new ocean floor is formed as seen in red in image 2.8.2. As the ocean floor ages it sinks to form broad plains that are about 5km deep. In image 2.8.2 you can see the varied texture of the seafloor. Here we are standing at the edge of Antarctica looking towards Madagascar. You can see the smooth seafloor close up, with a volcanic submerged plateau in the bottom left and the much rougher younger crust around a mid-ocean ridge further north. [Earthquakes] Plate tectonic motions are also responsible for geological phenomena such as earthquakes and volcanoes. Places like the San Andreas Fault in the Western United States, where two plates are sliding past one another and generate large earthquakes but no volcanoes. But commonly concentrations of volcanoes and large earthquakes occur at the same places, known as subduction zones, where one tectonic plate is being forced underneath another. Examples of these include Japan, the Andes, and Indonesia. [Tectonics and climate] Plate tectonics is also important in controlling regional and global climate over time scales of millions of years. We all know that today Antarctica is a very cold place that is located over the South Pole and is covered in thick glaciers, but it wasn't always that way. 100 million years ago, Antarctica was a much warmer place covered in forests, with abundant plant and animal life. One of the reasons that Antarctica is so cold now when it was much warmer before is because Antarctica became isolated at the pole\ roughly 35 million years ago, as Australia and South America broke away and moved northwards. This enabled the establishment of the strong eastward flowing current all the way around Antarctica, known as the Antarctic Circumpolar Current (ACC) that continues today, which keeps Antarctica colder than it otherwise would be. [Discussion Question] Can you think of any reason why plate tectonics might be an important consideration in an economic context? Plate tectonics can have a significant impact on the economy, influencing industries like mining, energy, and infrastructure. The movement of the plates can create geological formations rich in valuable resources such as oil, natural gas, and minerals. For example, many of the world's largest mineral deposits are found near tectonic plate boundaries. However, tectonic activity can also result in natural disasters like earthquakes and tsunamis, which can disrupt economies by damaging infrastructure. **2.9 Antarctic and the Southern Ocean** [ACC] The Southern Ocean is the only place on Earth where waters can transit 360° of longitude, unimpeded by land barriers. The major current that encircles Antarctica is the Antarctic Circumpolar Current (ACC) which is primarily driven by strong westerly winds -- the roaring forties and furious fifties -- and its pathway is also steered by the shape of the sea floor. The current itself is a series of jets, and together they transport a volume of water equivalent to 135 times the outflow of all the rivers in the world. The jest often become unstable, forming abundant eddies that can be seen in satellite images of temperature and chlorophyll. The ACC acts as a barrier between Antarctica and the southern gyres of the Indian, Atlantic and Pacific Oceans, keeping Antarctica cold, with important consequences for global climate. Although it is a barrier for north-south transport, it's an important connector in and east-west sense, and it is central to the global conveyor belt. [Bottom waters] The Southern Ocean plays a critical role in global ocean circulation and nutrient cycling. It features strong vertical currents that bring deep waters, formed in the North Atlantic, to the surface after hundreds of years. Near Antarctica, these upwelled waters either flow south, where cold temperatures and ice formation make them the coldest in the world, or north, where they are warms and eventually sink below lighter subtropical waters, continuing towards the equator. This circulation affects the chemical and biological properties of the Southern Ocean. Upwelled waters heading north carry nutrients that support summer productivity and provide essential nutrients to subtropical and tropical ocean where they sink. The process also facilitates carbon dioxide exchange between the ocean and the atmosphere, though the Southern Ocean remains a major uncertainty in global carbon budgets. Seasonal changes in sea ice cover significantly impact the region. In winter, sea ice doubles Antarctica's size, covering 30% of the Southern Ocean, with most ice forming and melting annually. This ice, typically 1-2m thick, excludes salt during formation and melts into fresh layers that support phytoplankton blooms. [Antarctica is affected by climate change] The Antarctic peninsula is one of the most rapidly warming places on Earth, but unlike the Arctic, it seems that Antarctic sea ice is not in rapid decline. The large-scale winds that drive the ACC are also changing, due to both ozone depletion and greenhouse warming, and this has consequences for upwelling and the delivery of nutrient to subtropical and tropical oceans. [Discussion Question] What is the significance of the Antarctic circumpolar current in climate change? How does melting glacial ice provide a positive feedback for climate change and sea level rise? The Antarctic Circumpolar Current (ACC) plays a crucial role in regulating the Earth's climate. As the world's strongest ocean current, the ACC connects the Atlantic, Pacific, and Indian Oceans, helping to circulate heat, nutrients, and carbon dioxide across the globe. This circulation impacts global weather patterns and the exchange of carbon between the ocean and the atmosphere. Also, the ACC acts as a barrier, keeping Antarctica's water cold, which helps maintain ice sheets. Melting glacial ice contributed to a positive feedback loop that accelerates climate change and sea level rise. As glaciers and ice sheets melt, the expose darker surfaces like ocean water or land, which absorbs more solar radiation instead of reflecting it. This increased absorption leads to further warming and additional ice melt, creating a positive feedback loop. ). **2.10 Climate and weather** [Definition of climate and weather] ! Climate is what we expect, temperature is what we get ! Mathematically, we can think of climate as a probability distribution and weather as a random sample from that distribution. Most of our expectation fall in the central part of the distribution and defines the average climate. There are tails to the distribution too and these represent the extreme events, which may be hot, cold, rainy, or dry depending on what aspect of the climate we are interested in. [Climate variability] Climate variability encompasses the natural fluctuation in weather patterns over time, distinct from long-term global warming. These variations occur across different timescales, from years to centuries, and follow organised patterns known as "modes". Key modes include: - Indian Ocean Dipole (IOD) Characterized by phases affecting sea surface temperatures. A positive phase shows warmer water in the western Indian Ocean and cooler water in the east, while the negative phase reverses this pattern. The IOD influences rainfall and monsoon patterns, particularly in surrounding regions. - Southern Annular Mode (SAM) Involves shifts in atmospheric pressure between Antarctica and the mid-latitudes. A positive SAM phase is linked to stronger westerly winds and colder Antarctic conditions, while the negative phase weakens these winds and raises pressure over Antarctica. SAM affects Southern Hemisphere weather, including rainfall in Australia. - El Niño Southern Oscillation (ENSO) La Niña: Cooler-than-average conditions in the same region, often bringing heavy rains and flooding to Australia while causing drought in parts of South America. El Niño: Warmer-than-average sea surface temperatures in the eastern equatorial Pacific disrupt global weather, often leading to droughts in Australia and floods in South America. ENSO is particularly impactful. The 1997/98 El Niño caused sever global disruption, while the 1988 La Niña brought widespread flooding in eastern Australia. A prolonged La Niña from 2010-2012 resulted in two of Australia's wettest years, massive flooding, and a marine heatwave off Western Australia. [El Niño] Under normal condition, strong westward-blowing trade winds over the tropical Pacific Ocean contain warm surface waters into the Western Pacific, maintaining what is called the "Indo-Pacific warm pool". El Niño develops when these westward blowing trade winds weaken, and the warm surface waters are blown to the east. This warm water, which evaporates easily and warms the atmosphere, can hasten the formation of rainfall and storms in the Eastern Pacific.  [Impact of El Niño] The El Niño variation has been related to human disease and conflict. **3.1 Our changing oceans** [Our changing ocean] The oceans store most of the energy from greenhouse effects, resulting in uneven warming. Since 1971, the top 75 meters of the ocean have warms by about 0.1°C per decade, while deeper waters have warmed more slowly. This surface warming decreases water density, weakening the overturning circulation that transports heat, carbon dioxide, and oxygen into the ocean interior. As the ocean warms, the water column expands, contributing to sea level rise. Since 1900, sea levels have risen by about 0.19m (1.7mm/year), primarily due to thermal expansion (0.8mm/year) and glacier and ice sheet melt. Climate change has also altered ocean salinity patterns. High salinity regions, linked to higher evaporation and desert areas, have become saltier, while low salinity regions, associated with higher rainfall, have become fresher. This reflects changes in the Earth's water cycle, with wet regions becoming wetter and dry regions dryer. These salinity changes are tied to global temperature increase and are expected to intensify as warming continues. [Projected change in our oceans] The oceans are predicted to warm around 2 degrees on average in the coming decades due to the effects of climate change and the oceans are gradually absorbing heat that is building up in the atmosphere as a direct result of human activities. [Discussion Question] How is the nature of marine heat waves changing? What are the drivers and impacts? Marine heatwaves are characterised by unusually high seas surface temperatures and are becoming increasingly frequent. This trend is driven by climate change, which raises ocean temperatures, and natural climate processes like El Niño, which amplify warming. Additionally, ocean current and eddies contribute to localized heatwaves. Over the past decade, these events have become 50% more common, posing a significant risk to marine ecosystems. The impacts of marine heatwaves are severe and widespread, as they disrupt ecosystems by causing coral bleaching, destroying kelp forests, and triggering mass die-offs of marine species, which reduces biodiversity. These changes have a direct impact on tourism and fisheries, leading to economic losses for communities who rely on ocean resources... **3.2 Ice age: how has our climate changed before** [Ice cores] Drilling ice cores is a logistically complex operation requiring large teams and international collaboration. After the cores are drilled they are carefully logged and then samples are returned to the laboratory for detailed analysis. As the ice accumulates the air gets trapped in bubbles and sealed off from the atmosphere. The ice itself can be melted and analyses and can mini particles\ trapped in the ice (aerosols). The cores contain a wealth of climate relevant information, not only temperature and CO₂, but also other greenhouse gases such as methane and nitrous oxide, aerosols, and even human produced contaminants such as lead and mercury. They even trap aerosols produced by phytoplankton in the oceans. An interesting example is methane sulphonic acid (MSA), a product of biological activity in sea ice. The ice core record of MSA can be used to look back at\ \ past trends in Antarctic sea ice extent. [Marine sediment cores] Marine sediment cores tell us how the ocean responded to past climate change, in terms of temperature, productivity, pH, oxygen and sea level. Just as ice cores can tell us about the atmosphere in the past, marine sediments record past changes in ocean properties. Because of its role in the carbon cycle there is a lot of interest in understanding past changes in ocean productivity. One way this can be done is to measure the accumulation in the sediment of the remains of organisms -- either chemical remains or micro-fossils. These layered, or laminated sediments are an indication that oxygen levels at the seafloor are too low to support animals that live in the sediment and stir it up. Some trace metals such as rhenium and uranium also become enriched in sediments when oxygen levels are low. Ocean deoxygenation is a growing concern as the earth warms. Sea levels have been rising at a rate of 3mm/year over the past 20 years and is expected to rise by about 1m about pre-industrial levels by the year 2100. Fossil corals -- which live in shallow water -- can be used to estimate past sea levels. [Discussion Question] How fast is the planet warming and what are some challenges that species will have to contend with in order to adapt? The planet is warming at an alarming rate, with global temperatures having risen by approximately 1.2°C since pre-industrial times. This seems small, but this little increase has already caused noticeable changes in climate patterns, like more intense heatwaves, stronger storms, and rising sea levels. If current trends continue, we could see a temperature increase of up to 3°C by the end of the century. This warming is primarily driven by human activities, such as the burning of fossil fuels and deforestation. As the climate warms, marine species face many challenges. Rising temperatures are causing coral bleaching, which leads to loss of habitat and biodiversity. Species such as polar bears and sea turtles are especially vulnerable, as they depend on specific temperature ranges, which are now shifting due to planted warming. **3.3 Do you live near a hot spot?** [Regional response to climate change] Image 3.3.1 shows the warming over the last century, in degrees Celsius. Regions that are deep red or purple such as Siberia, Canada, and Brazil, are warming faster than the global average while regions that are pale yellow, including many areas over the open ocean, are warming slower than the average rate. We even detect a cooling in some regions, shown with a light blue colouring, such as the southeastern USA and over the North Atlantic Ocean. [South pacific and the Tasman sea ] Ocean hotspots, areas of rapid warming, differ from global warming patterns and are influenced by wind changes. These hotspots often occur on the western sides of ocean basins, such as the Southern Indian Ocean, the Atlantic, and Pacific Oceans. In particular, the Tasman Sea between Australia and New Zealand is warming at nearly 4 times the global average rate, driven by increased westerly wind that push surface currents poleward, creating warm and salty anomalies. Temperature changes in these hotspots affect marine ecosystems by altering species' distributions and life cycle event, such as spawning and moulting. These rapidly warming areas serve as "natural laboratories" for studying climate change impacts and developing adaptation strategies. Ocean temperature extremes, or "marine heatwaves", are expected to increase, potentially disruption local ecosystems. Regions like the Galapagos Islands and waters around Alaska are already experiencing significant biodiversity losses and ecosystem changes due to these warming trends and other factors like overfishing. [Marine species are 'shifting' in time and space] Marine species are 'shifting' (permanently moving from where they live) their ranges in response to climate change, moving away from area that are becoming too warm and moving towards cooler waters, particularly the poles. These shifts are not seasonal migrations but permanent relocations as species track their preferred temperature ranges. However, not all species can shift, and the rate at which they do varies. They complexity of these changes requires extensive observation and funding. **3.4 Who cares about a few degrees?** [What will 2 degrees warming do to marine ecosystems?] Warming is already -- and will continue to -- affect marine ecosystems. In the tropics, corals live near to their upper thermal tolerance. Corals in fact live in a symbiotic relationship with single celled microalgae (dinoflagellates of the genus Symbiodinium) which reside within their tissues. These cells (often called zooxanthellae) photosynthesize to produce energy and a carbon source for corals and are what give coral their colour. When thermally stressed (or when the water is too warm for a certain period of time), corals tend to reject their zooxanthellae -- they effectively expel them from their cells and so they lose their colour, bleach, and turn to white. Bleaching doesn't necessarily kill corals -- in some cases they will take up new zooxanthellae from the water column and continue to grow. But at the very least it stresses them physiologically, and in more extreme bleaching event the corals die. Due to some corals being more heat tolerant than others, reduced coral cover occurs and a shift in community composition happens. The giant kelp (Proboscia inermis) shows responses to temperature over the seasonal range, with higher growth in warmer summer water -- but if there is warming much beyond the top end of the usual seasonal range, this species shuts down and stops growing altogether (tolerance range). Responses by individual species range from changes in: - Growth - Reproduction - Range shifts These kinds of records reveal that some Southern Ocean ecosystems undergo large and often ecosystem-wide changes in structure and function of ecosystems in response to warming. These changes are reversible -- as is observed with a subsequent period of cooling ocean temperatures. In contrast a 2-degree warming driven by human-induced climate change may not be reversible -- unless we change our attitudes towards CO₂ emissions. A 2-degree warming will pose some unique challenges to Southern Ocean ecosystems. Many polar and subpolar plants and animals appear likely to be threatened by warming as is being observed in the Arctic. [Discussion Question] Why do small changes in temp have a large impact on biology/ecology? Provide some examples of ecological impacts. What were the drivers and impact of the 2010/2011 marine heat wave off WA? Due to many species that live within small thermal ranges, slight changes in temperature can significantly affect biological and ecological systems. A small temperature rise can push organisms past their tolerance range, which affects their growth and reproduction. One example is that coral reefs bleach when water temperatures rise by 1-2°C above their normal range, as the zooxanthellae are expelled. Similarly, warming can disrupt seasonal cycles, such as the timing of phytoplankton blooms. The 2010/2011 marine heatwave located off Western Australia was driven by a strong La Niña event combined with unusually warm Leeuwin Current waters. This caused the sea surface temperature to rise up to 5°C above average in some areas. **3.5 Consequences of altered circulation** [Warming and freshening of the ocean surface leads to stratification] What is 'stratification'? The density of seawater is determined by temperature, salinity, and pressure. Seawater is denser or heavier if its colder and/or saltier. The ocean is layered -- or stratified -- according to density, with denser waters at the bottom (deepwater) and the least dense waters at the surface. There are also pronounced vertical gradients in ocean chemical properties: nutrients and carbon are elevated in deep water, and low in surface water. In contrast, the surface ocean has higher oxygen concentration than at depth, as oxygen is produced during photosynthesis and also regularly exchanged between surface waters and the atmosphere. Climate change is warming and freshening (less salty) the ocean surface. That is, there is a growing contrast in density between ocean surface layers and the layers below. This increase in density contrast, or increate in stratification, makes it harder to mix deep waters with surface waters. Ocean stratification has a number of chemical and biological impacts. [Ocean stratification reduces the supply of nutrients to plants] The deep ocean holds a vast reservoir of plant nutrients, while the surface waters are where the light is. So, if the 'normal' rate of mixing between the deep ocean and the surface ocean is decreased due to ocean stratification, there will be less nutrients available to support plant growth. The ocean gyres, sometimes referred to as the deserts of the oceans, give us a glimpse of what this might look like. The centres of the ocean gyres are already highly stratified, due to the prevailing circulation patterns. The vertical supply of nutrients to these regions is therefore very small, and as a consequence that have among the lowest rates of primary production in the oceans. The size of these oceanic deserts might expand in the future if ocean stratification intensifies. [Warming and stratification reduce the supply of oxygen to the ocean interior] Stratification also restricts the supply of oxygen-rich surface waters to the ocean interior. This in known as deoxygenation. While the cause of deoxygenation is still being determined, two factors certainly contribute. The first is that oxygen is less soluble in warm water than in cold. Think spaghetti, as the water warms up oxygen bubbles escape. So, as the ocean warms, it holds less oxygen. The second effect is related to stratification: decreased mixing between surface and deep waters decreases the physical transport of oxygen to the ocean interior. Deoxygenation in the open ocean (far way from the coasts) is caused primarily by large-scale climate change. Deoxygenation in coastal areas is called hypoxia, (red dots in image 3.5.1), meaning low oxygen and is caused by a combination of climate change and local, anthropogenic inputs of nutrients such as agricultural runoff, known as eutrophication (yellow dots). Both forms of deoxygenation (costal and in the open ocean) lead to impacts on ocean biodiversity and can affect the cycling\ of nutrient elements such as iron, phosphorus and nitrogen. Of\ particular concern is the possibility that low oxygen levels might accelerate the release of nitrous oxide from the oceans, as marine microbes produce for nitrous oxide under low oxygen conditions. Nitrous oxide is a potent greenhouse gas. Another more familiar greenhouse gas, carbon dioxide, is also affected by ocean warming and stratification. The 'solubility pump' carries carbon-rich cold surface waters to depth during deepwater formation. Because CO₂, like oxygen, is more soluble (able to be dissolved) in cold water\ than in warm water, and because deepwater formation is reduced in a stratified\ ocean, we might expect the solubility pump to be weakened in the future. This is a concern, because the 25% of anthropogenic CO₂ taken up by the oceans to date had been taken up almost exclusively by the solubility pump. [Alteration of large scale ocean overturning ] If the North Atlantic warms and freshens significantly then even large scale ocean overturing (eg. Thermohaline circulation) could be altered. This deep ocean current links to atmospheric circulations that ultimately deliver warm equatorial air to North America and Europe. The Atlantic deep water mass forms when salty water flowing north from the Mediterranean cools in winter off the coast of Iceland and become dense enough to sink to depths of several thousand meters. As river flow increases in the Arctic, and glaciers melt there, it is a concern that the combination of lower salinity and warmer temperature might actually lead to a decrease in the production of NADW (north Atlantic deep water). **3.6 Ocean acidification** [Why should we care about ocean acidification] Ocean acidification impacts organisms that produce a hard shell (the calcifiers) and those that don't (like marine plants). The excess CO₂ in the atmosphere due to burning fossil fuels is absorbed by seawater, causing pH to decline. So, more CO₂ in the atmosphere = more CO₂ absorbed into the ocean. This is causing the ocean to acidify, which is referred to as ocean acidification. Some calcifiers (eg. shells and corals) are vulnerable to dissolution and will have to expend more energy to build their shells. In comparison, man non-calcifiers (eg. algae and seaweed) thrive under a high CO₂ world. Ocean acidification took off with the industrial revolution (1780). And we have already seen some effects on marine life and on industry. For example, oyster farms in the US suffered a large financial loss when their juvenile oysters died. On these farms, seawater is pumped off the coast to supply the hatcheries. When acidified water were pumped into the hatcheries there was a large mortality of juvenile oysters. In response, and ocean acidification buoy was installed off the coast and the pump can now be turned off when the seawater is too acidic. [Calcifying marine organisms are susceptible to dissolution] A large number of marine organisms form parts of their bodies from calcium carbonate, which they manufacture from the calcium and carbon ions. Organisms obtain the calcium and carbonate ions from seawater. Corals are foundation species in the tropics in that they create coral reef habitats. OA (ocean acidification) is an additional stress for tropical corals given that they are already impacted by thermal and pollution threats in many areas (eg. GBR). Coralline seaweeds are calcifying red seaweeds. They form the 'glue' that stabilises the substrate of coral reefs and temperate reefs, and they also release chemicals that induce the free-swimming larval phases of invertebrates, eg. abalone and urchins. Laboratory experiments have shown that coralline seaweeds are susceptible to OA. Coccolithophores are calcifying phytoplankton that form massive blooms and are of major importance in sediment and rock formation, and in carbon cycling. The White Cliffs of Dover are formed from coccolithophores. There are an extraordinary diversity of species and strains of coccoliths, many of which appear tolerant and able to adapt to OA. Pteropods -- also known as sea butterflies -- are planktonic molluscs. They are very important in polar seas and are believed to be a key link in the food web between primary producers and fish. Finally, shellfish, such as oysters, mussels and abalone play important\ roles in structuring coastal ecosystems. These species synthesis their protective shells from calcium carbonate. Shellfish are arguably the most susceptible group to OA as their calcifying structures are used for protection against predators. Research shows that under acidic condition, shellfish re-allocate energy to\ maintain their protective shells and so have\ lower rates of muscle growth and reproductive output. [Non-calcifiers may benefit from OA] About 99% of marine plants do not calcify. For most of these species, the current levels of CO₂ in seawater are lower than those required for optimal photosynthesis and growth. Indeed, many have developed mechanisms called 'carbon concentrating mechanisms' (CCM) which allow them to increase their access to CO₂. Phytoplanktonic diatoms and benthic seaweeds might grow faster in a future low pH ocean. [Ecosystem shift] Changes to one (or more) tropic level can have flow-on effect throughout the food web. One potential food web consequence of OA could stem from a change to the nutritional quality of seaweeds. If there is more CO₂ available to seaweeds, but the level of nitrogen remains the same, then those seaweeds will contain less protein, and so animals will have to consume greater quantities to acquire sufficient energy for growth. Ocean acidification also appears to affect fish behaviour. Clown fish like nemo lose their sense of smell and hearing under high CO₂ conditions, which makes them more susceptible to predators. **3.7 Cumulative environmental stress** [It's not just about a warming ocean] Changes to the ocean environment are not just to do with temperature. Instead of talking about global warming we now talk about climate change, which better reflects that a whole range of oceanic conditions will be altered by a changing climate. Ocean properties that will be altered by climate change include temperature, oxygen concentration, pH (acidification), light levels, and nutrient supply. This alteration even includes the strength and direction of flow of coastal boundary currents, and the locations where current systems meet. Alteration of these properties will influence all levels within ecosystems, with phytoplankton (microscopic plants, macroalgae (seaweeds) and even sea grass productivity being set by temperature, pH , carbon dioxide concentrations, light levels, and nutrient supply. Animals in food webs will also be altered by these properties. Not only that but changing patterns in ocean currents will alter the spatial availability of optimal environment for planktonic larval phases, and indeed a wide array of pelagic species that track regions of optimal opportunity. So, the flows of energy (from photosynthesis) into ecosystems may be altered which will influence the performance of ecosystems. [Environmental stressors can magnify each other] Changes to the aforementioned properties will each influence plants and animals. However, such stressors can also interact with each other to magnify the effect of individual environmental properties, or in other cases the joint effects may cancel each other out. For example, as warmer ocean will have a decreased capacity for dissolved carbon dioxide (as it will be less soluble) which might offset the rates of acidification in the future. Understanding these kinds of interactions between multiple stressors is very challenging and requires the design of complex experiments. Sometimes these 'experiments' can occur naturally and was can then deduce some of the interactions. One example of a situation where multiple stressors can influence marine community structure can be found from monitoring benthic invertebrate communities (those living on the sea floor) is Bathurst Channel. In this system the rivers that flow into the estuary carry a high load of tannins derived from terrestrial vegetation in the region. This 'red' tannin stained freshwater sits over the top of the marine waters below, providing a natural light filter that blocks almost all light at depths below 3m, and allows invertebrates like soft corals and sea whips to flourish on rock reef systems where algae would normally grow and exclude such invertebrate life. [Topping up environmental stress with pollution and harvesting] The widespread global effects of changing oceanic conditions on marine plants and animals can also be topped up by local effects -- such as fishing pressure and/or pollution -- on ecosystems. The combination of global and local effects can therefore increase the build-up of such stresses (cumulative stress) that can plants and animals 'over the edge'. Due to coral reefs being situated close to land they are often subjected to sediment and nutrient runoff form agricultural activities. These stressor act to reduce coral growth rates and increase algae growth rates. At the same time, overfishing of herbivorous fish such as parrotfish can allow algae to grow unchecked. This combination of pollution, fishing and increased temperatures can spell disaster for coral reef systems that can tip to a different stat with lots of algae and very little coral. Marine protected areas such as the GBR can be important tools to understand the interactions between multiple stressors and to help mitigate their effects. **3.8 East Australian current** [EAC] Remember 'Finding Nemo'? Marlin (that's Nemo's Dad), Dory and a group of turtles hitch a ride on a powerful ocean superhighway which sweeps them from the Great Barrier Reef to Sydney Harbour in their search for Nemo. That ocean superhighway is the East Australian Current, or EAC, and it is the western boundary current of the huge oceanic gyre circulating right across the South Pacific Ocean -- the South Pacific Gyre. The gyre is a surface current that runs eastwards across the Southern Ocean to become the Humboldt current\ at its eastern margin (running south to north up the west coast of South America) and then back across the Pacific in a westward direction as the South Equatorial Current until it reaches Australia, where it becomes the EAC moving southwards down the east coast. The EAC flows at speed of 2-3 knots, occasionally reaching 5-7 knots near the continental shelf. Around Port Macquarie, it splits into 2 branches: one forms the Tasman Front, moving across the Tasman Sea and around New Zealand, while the other\ extends southwards along Australia's east coast, generating large eddies. Over the past 5 decades, the EAC's behaviour has shifted due to increased wind stress in the Southern Ocean, initially caused by the Antarctic ozone hole and now exacerbated by climate change. More EAC water now flows southward, creating l