Basidiomycota: General Account - Unit II PDF
Document Details
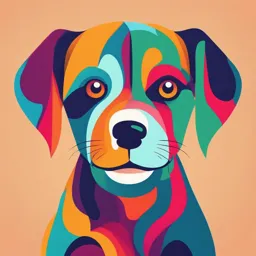
Uploaded by ThankfulRational
University of Kashmir
Tags
Summary
This document provides a general account of Basidiomycota, a phylum of fungi including mushrooms, polypores, and plant pathogens. It discusses the characteristic structures like basidia and basidiospores, along with details on mycelium structure, reproduction methods, and various types of basidia. Key topics covered include fungal classification, the formation of hyphae, and spore release mechanisms.
Full Transcript
“Unit II” Basidiomycota_ General Account The phylum Basidiomycota includes such well known macrofungi like mushrooms, polypores, puff balls, earth stars, bird‟s nest fungi and others (Fig. 2.1, 2.2). The group also includes important plant pathogenic fungi which cause rust and smut diseases of highe...
“Unit II” Basidiomycota_ General Account The phylum Basidiomycota includes such well known macrofungi like mushrooms, polypores, puff balls, earth stars, bird‟s nest fungi and others (Fig. 2.1, 2.2). The group also includes important plant pathogenic fungi which cause rust and smut diseases of higher plants. About 1100 genera and 16,000 species are described in this group. The most important character of the group is production of sexual spores called basidiospores on specialized structure described as basidium (pl. basidia). The basidia in macrofungi are formed in fruit bodies called basidiocarps, but basidiocarps are not formed in pathogenic fungi. Over a period of time, some of the common macrofungi came to be known by their common names (Table 2.1). Some of the macrofungi and their common names are: Technical name of Macrofungus Common name Family Agaricus campestris Field mushroom Agaricaceae Agaricus bisporus Button mushroom Agaricaceae Amanita muscaria Fly agaric Amanitaceae Amanita caesaria Caesar‟s mushroom Amanitaceae Amanita phalloide Death cup Amanitaceae Amanita virosa Destroying angel Amanitaceae Coprinus atramentariu Inky cap mushroom Coprinaceae Termitomyces heimii Termite nest fungus Coprinaceae Strobilomyces Old man of the woods Boletaceae Psilocybe Mexicana Sacred mushroom Stophariaceae Lactarius deliciosus Saffron milk-cap Russulaceae Pholiota samino Viscid mushroom Cortinariaceae Volvariella volvacea Paddy straw mushroom Pluteaceae Pluteus cervinus Saw dust mushroom Pluteaceae Pleurotus spp. Oyster mushroom Tricholomataceae Lentinus edode Shiitake mushroom Tricholomataceae Marasmius oreades Fairy ring fungus Tricholomataceae Omphalotus oleariu Jack-o-lantern Tricholomataceae Armillariella mellea Honey mushroom Tricholomataceae Flammulina velutipes Enokitake mushroom Tricholomataceae Cyathus spp. Bird‟s nest fungus Cyathaceae Phallus spp. Stink horn Phallaceae Lycoperdon spp. Common puff ball Lycoperdaceae Clavatia gigantean Giant puff ball Lycoperdaceae Tulostoma spp. Stalked puff ball Tulostomataceae Geastrum spp. Earth star Geastraceae Clavarius spp. Coral fungi Clavariaceae Polyporus, Ganoderma, Fomes, etc. Bracket fungi Polyporaceae Hydnum sp. Tooth fungi Hydnaceae Auricularia polytricha Wood-ear fungus Auriculariaceae Fig. 2.1. Basidiomycota. 1. Polyporus sp. 2. Dictyospora sp. 3. Urediniospores of Puccinia. 4. Coprinus sp. Fig. 2.2. Basidiomycota. 1. Russula sp. 2. Boletus sp. 3. Phallus sp. 4. Laccaria sp. 5. Scleroderma sp. 6. Psilocybe sp. Mycelium: The mycelium of basidiomycetous fungi consists of well developed, septate hyphae. The cell wall is made of chitin and glucans, cellulose is absent. In free living, saprophytic macrofungi, extensive vegetative mycelium develops in soil or in other substrata while in plant pathogenic forms (rusts and smuts) mycelium is limited in the host tissue, and the hyphae sends in haustoria into the host cells to draw nourishment. The hyphae of basidiomycetous fungi are septate and the septa possess a pore. Because of the septal pores the protoplasm is continuous throughout the mycelium. The septal pore in basidiomycetes is barrel shaped and is described as dolipore septum (Fig. 2.3). Endoplasmic reticulum forms a layer of covering on either side of the pore and it is described as parenthosome. The parenthosome is not continuous but has a number of pores and hence there is no obstruction to cytoplasmic streaming. However, in rust fungi and smut fungi dolipore septum is absent. They contain a simple pore in their septal walls. Fig. 2.3. Structure of dolipore septum. 1. Layers of cell wall. 2. Endoplasmic reticulum. 3. Middle lamella. 4. Barrel shaped structure. 5. Septal pore. 6. Parenthosome The mycelium of these fungi passes through three stages called primary mycelium, secondary mycelium and tertiary mycelium. Primary mycelium: This is formed when basidiospores germinate and produce fungal mycelium comprising of uninucleate hyphal cells. This uninucleate stage is called primary mycelium. It is usually very much limited. Secondary mycelium: When two compatible primary mycelia are growing side by side, somatogamy occurs between them forming dikaryotic stage. The nuclei divide mitotically and spread throughout the mycelium, thus the entire mycelium becomes dikaryotic. This dikaryotic stage of the mycelium is described as secondary mycelium. The dikaryotic mycelium may also form when germ tubes from two compatible basidiospores fuse, or by spermatization of primary mycelium. Tertiary mycelium: The mycelium that forms basidiocarps is called tertiary mycelium. Like in secondary mycelium, all the hyphal cells of tertiary mycelium are also dikaryotic. Fig. 2.4. Development of clamp connection in secondary and tertiary mycelium. A. tip of a dikaryotic hypha. B-E. Method of formation of clamp connection. In secondary and tertiary mycelium, during cell divisions specialized structures called clamp connections are formed, and help to maintain dikaryotic condition of the daughter cells with two compatible nuclei (Fig. 2.4). Asexual reproduction: In Basidiomycotina fungi, asexual reproduction is not generally found. A few species produce conidia. In some species the vegetative cells separate as individual cells and disperse. Such individual cells which disperse are described as arthrospores. In rust fungi urediniospores are considered as conidia. In smut fungi basidiospores germinate to produce more cells which grow by budding as yeasts until the dikaryon is established. Sexual reproduction: In Basidiomycetes no specialized sexual reproductive structures are formed. Plasmogamy occurs either by somatogamy or spermatization to form dikaryotic mycelium, called as secondary mycelium which grows extensively. When conditions are favourable, the fruit bodies called basdiocarps are formed by mycelium termed as tertiary mycelium. Even though each dikaryotic cell is potentially capable of forming basidiospores, karyogamy occurs only in specialized cells called basidia. The basidia are formed at the tip of fertile hyphae. The fertile hyphae form a layer called hymenium. In agarics the gills are lined with hymenium, in polypores hymenium lines the pores. Hymenium formed on gills is shown in Fig. 2.5. Fig. 2.5. Hymenium in basidiomycetous fungi. 1. Cystidium. 2. Basidiospores. 3. Basidium. In the hymenium, some sterile structures are also present interspersing basidia. These are called cystidia which usually have a narrow stalk and upper globose structure which may be bigger than basidia. Basidium: Basidium may be defined as a structure bearing on its surface a definite number of basidiospores (usually four). The two nuclei in the basidium fuse and meiosis follows immediately after karyogamy producing four haploid nuclei. As meiosis is occurring, four sterigmata develop on the top of the basidium. One haploid nucleus enters each sterigma and one basidiospore is formed at the tip of the sterigmata. Based on the structure, the basidium is described as of two type viz. holobasidium and phragmobasidium. Holobasidium is a single celled, typically club shaped structure with four small sterigmata at the top. It is mainly found in Gasteromycetes and majority of Hymenomycetes. It is often described as a typical basidium. However, in some small groups like the members of Tulsanellales and Dacrymycetales, even though the basidium is single celled, it is not typical, and shows some structural variations. In the order Tulsanellales, the sterigmata are very big and prominent structures. In the order Dacrymycetales, the basidium is a curved structure with U-shaped appearance. Phragmobasidium is a multicellular structure usually with four cells. This type of basidium develops due to formation of transverse or longitudinal septa after meiosis. It is found in the members of the subclass Phragmobasidiomycetidae and members of the class Teliomycetes. Up to the stage of meiosis, the basidium is single celled, but as meiosis occurs and four nuclei are formed, basidium becomes septate to form a four celled structure. The septa are formed longitudinally in the order Tremellales, while the septa are formed transversly in Auriculariales, Septobasidiales and the members of Teliomycetes. Different types of basidia are shown in Fig. 2.6. Fig. 2.6. Basidium types in different genera Depending on the stage of development, the basidium is described as of two type‟s viz. Probasidium and Metabasidium. The stage or part of the basidium in which karyogamy occurs is called probasidium, while the stage or part of the basidium in which meiosis occurs is described as metabasidium. In the members of Phragmobasidiomycetidae, the single celled stage in which karyogamy occurs is probasidium and septate structure with four cells formed after meiotic division is metabasidium. In rust fungi and smut fungi, the teliospores represent probasidium and promycelium formed due to germination of teliospore represent the metabasidium. Basidiospores: Basidiospores are typically unicellular, and may be globose, oval, elongated or variously shaped. They may be colourless or pigmented. In many cases, the pigments are very dilute and visible only in spore mass but not in individual spores. The spore prints may be of different colours like white, green, red, 152 pink, different shades of brown etc. The basidiospore wall is usually thick and made up of two layers. The outer layer is called epispore and the inner layer is called endospore. In most of the species the spore wall is smooth, but in some the outer layer may be having different types of thickenings. In the genus Ganoderma, the outer layer is smooth, while conspicuous spiny thickenings are seen on the inner layer. The outer layer appears as a hyaline covering over the spines. Different types of basidiospores are shown in the Fig. 2.7. Fig. 2.7. Different types of basidiospores Basidiospore release: In majority of Hymenomycetes, basidiospore is formed on the tip of sterigma in an inclined position, and is actively discharged. Very close to the point of its attachment to the sterigma, the spore has a minute projection referred to as hilar appendix. A small bubble or drop forms at the hilar appendix as the spore matures. It is thought to be involved in active release of basidiospore. It was first described by A.H.R. Buller and hence is described as Buller‟s drop. At the time of spore release, the drop bursts and the spore is thrown out with force (Fig. 2.8). Various other theories such as electrostatic repulsion, jet propulsion, surface tension, rounding off of turgid cells etc. have also been proposed to explain the active release of basidiospores. Fig. 2.8. Liberation of basidiospore. 1. Sterigma. 2. Basidiospore. 3. Buller‟s drop. 4. Liberated basidiospores. Morphology, Reproduction and Life cycle of 1. Ustilago Kingdom: Fungi Division: Basidiomycota Class: Ustilaginomycetes Order: Ustilaginales Family: Ustilaginaceae Genus: Ustilago. The genus Ustilago comprises of about 300 species, and all of them cause smut disease on higher plants, especially, graminaceous hosts. The term smut refers to the mass of dark powdery spore mass formed in the sori formed by the pathogen on the infected host. Various parts of the plant are infected, but most commonly infected part is inflorescence forming smut sori instead of grains. Generally no symptoms appear on the infected host until the smut balls are formed. However, on maize, the infection results in formation of galls on various infected parts including stalks, leaves and ears. In sugarcane, the infected inflorescence is transformed into a long, tapering, whip like structure. Some important species of Ustilago and the diseases they cause are given below (Table 2.2). Table 2.2. Some important diseases caused by Ustilago spp. Pathogen Disease Ustilago tritici Loose smut of wheat U. avenae Loose smut of oats U. nuda Loose smut of barley U. hordei Covered smut of barley U. kolleri Covered smut of oats U. maydis Smut of maize U. scitaminea Whip smut of sugarcane The life cycle of the smut fungus starts with the germination of the resting spores. The smut spores are functionally equivalent to the teliospores of rust fungi. These teliospores are binucleate, globose in shape, smooth, light brown in colour, measure 6-10 μm in diameter. During the process of maturation or just before the germination the two nuclei fuse forming diploid nucleus. Germination of resting spores: The teliospore germinates to produce a germ tube or promycelium, into which the diploid nucleus passes and undergoes meiosis. Then the promycelium develops three transverse septa to cut off four cells, each containing a single haploid nucleus. Within each cell the nucleus divides and a daughter nucleus passes into a sporidium budded off from the cell. The four celled promycelium is metabasidium and the sporidia are considered as basidiospores. In Ustilago longissima, the cause of leaf stripe of Glyceria spp. the teliospores on germination do not produce obvious promycelium but merely a short tube from which sporidia are budded off successively. In Ustilago violacea, causing smut of Caryophyllaceae members, the teliospores germinate by producing three celled promycelium and from each cell a sporidium is formed by budding. They detach from the teliospore and may continue to develop sporidia even after separation. The bipolar nature of incompatibility in smuts was first demonstrated in this fungus. In Ustilago nuda, the cause of loose smut of wheat and barley, there are no sporidia. A septate promycelium develops on germination and becomes primary mycelium. Dikaryotization: Most species of Ustilago are heterothallic and show bipolar heterothallism controlled by a single gene and two alleles. Of the four cells of promycelium two contain one mating type and other two the opposite mating type. The sporidia produced are thus of two mating types. In some species two sporidia (basidiospores) of opposite mating type may copulate, in other species a basidiospore of one mating type may unite with a hypha issuing from a basidiospore of the opposite type. In Ustilago nuda, the dikaryotic phase is established by fusion of germ tubes derived from the individual uninucleate cells of the promycelium. Regardless of how dikaryotization takes place, it is the binucleate mycelium that carries on the life history of the fungus. Uninucleate mycelium seems to be of short duration and seldom grows very much. Normally it bears no clamp connections and forms no teliospores. Sorus development: During sorus development, dikaryotic hyphae proliferate and mass together in the intercellular spaces, often destroying the softer internal host tissues, but remain enclosed in the host epidermis. Eventually all hyphae are composed of binucleate cells. After the nuclear fusion, the walls thicken and gelatinize. Meanwhile the cells enlarge to become globose. The spore initials are at first enclosed in a gelatinous matrix, which disappear at maturity. The ripe, smooth or ornamented by spines or reticulations. Dissemination of smut spores: The teliospores are commonly dispersed by wind and germinate by a variety of methods. The secondary mycelium in the host may produce branches that reach the surface of the host and give rise to several crops of binucleate conidia that are disseminated by wind and initiate new infections. Later binucleate mycelium forms smut ball (sorus) in which teliospores develop. Over wintering: The smut fungi over winter in the teliospore stage or as mycelium in the host. Ustilago maydis over winters as teliospores that may survive in the soil or in the corn debris. Life cycle: The important stages in the life cycle of Ustilago maydis are shown in Fig. 2.9. Fig. 2.9: Life cycle of Ustilago sp. 2. Puccinia Kingdom: Fungi Division: Basidiomycota Class: Uredinomycetes Order: Uredinales Family: Pucciniaceae Genus: Puccinia. The species of Puccinia are obligate parasites on higher plants causing rust disease. About 700 species have been described in the genus so far, and of them about 125 species are recorded in India. They attack a number of crop plants, weeds and trees. Important crop plants attacked by rust fungi include wheat, barley, sorghum, maize, bajra, groundnut, sunflower and others. Of all these, the black stem rust of wheat caused by Puccinia graminis tritici is the most serious disease causing severe yield losses. Some important rust diseases of crop plants caused by Puccinia species are given below (Table 2.3). Table 2.3. Some important diseases caused by Puccinia species. Crop Common name of the disease Puccinia species Wheat Black stem rust Puccinia graminis tritici Wheat Yellow stripe rust P. striiformis Wheat Orange leaf rust P. recondita Maize Maize rust P. maydis, P. sorghi Sorghum Sorghum rust P. purpurea Bajra Bajra leaf rust P. penniseti Groundnut Peanut rust P. arachidis Sunflower Sunflower rust P. helianthi In the life cycle of Puccinia species five types of spores are formed. They are 1. Pycniospores or spermatia, 2. Aeciospores, 3. Urediniospores, 4. Teliospores (also described as teleutospores) and 5. Basidiospores. These spores are formed sequentially in a cyclic fashion in the life cycle, and are designated as 0, I, II, III and IV spore types or stages. All these five types may or may not be formed in same species of Puccinia. One or two spore types may be missing in the life cycle. When all the five types of spores are formed, the life cycle is described as macrocylic. If one or two spore stages are missing in the life cycle, it is described as microcyclic. The species of Puccinia which complete the life cycle producing all the spore types on a single host are described as autoecious species (Puccinia asparagi, P. helianthi). The species which requires two different hosts to complete the life cycle are described as heteroecious (Puccinia graminis). The host on which perfect stage (sexual stage) occurs is considered as the primary host and the other as alternate or secondary host. Life cycle of Puccinia: Among all the species of Puccinia, Puccinia graminis tritici causing black stem rust of wheat was most thoroughly studied, and hence, it is taken as a good example for the life cycle of Puccinia species. Mycelium is well developed and consists of hyphae which are septate, branched moderately. There are two types of hyphae. Primary hyphae are those which form after the germination of basidiospores. These primary hyphae are unicellular and multinucleate in the beginning but due to formation of septa they become multicellular and uninucleate. These are haploid and are of two strains. One is represented by + ve sign and other by – ve sign. These hyphae form monokaryotic mycelium. Secondary hyphae are multicellular and binucleate, form dikaryotic mycelium. The monokaryotic mycelium grows on barberry (Berberis vulgaris, secondary host) and secondary mycelium on wheat (primary host), thus the fungus is described being macrocylic, and heteroecious (Fig. 2.10). Both primary and secondary mycelia are not very deep seated but they grow close to epidermis of host. Fig.2.10 Symptom of Rust on Wheat stem (i & ii) and Berberry leaf (iii). Stages on wheat: Due to infection by Puccinia graminis tritici two stages are formed on wheat. They are uredinial stage and telial stage. Uredinial stage: When aeciospores released from infected barberry bushes are deposited on wheat leaves, they germinate under favourable conditions and germ tubes enter the leaves through stomata. The stems may also be infected. After an incubation period of about one week, the mycelium aggregates below the epidermis and produces large number of urediniospores. As the mass of urediniospores increase, the epidermis ruptures due to internal pressure, and the urediniospores are exposed. The spots formed by the rupture of epidermis are called pustules. They are also called uredinia or urediniosori. The pustules on wheat leaves and structure of uredinial sorus are shown in the Fig. 2.11. Fig. 2.11. Uredinia and uredinial sorus in Puccinia. A. Uredinial pustules on wheat leaf. B. Structure of uredinial sorus. The urediniospores become easily airborne and when they are deposited on wheat leaves or other plants they germinate and readily infect the leaf, and after an incubation period of about one week, new pustules are formed. Since the uredinial pustules are formed repeatedly in the same crop season, it is called „repeating stage‟, and the urediniospores are described as „conidia of rust fungi‟. When the urediniospores are deposited on wheat leaves, they germinate under favourable conditions. High relative humidity, near saturation point, or free water is essential for germination of urediniospores, and optimum range of temperature is 20 – 25oC. The germ tubes grow and form a round thin globose structure called appressorium. The appressorium helps to keep the germ tube attached to the leaf surface. From the underside of the appressorium, an infection peg develops and enters the leaf through the stomata. The tip of the infection peg expands in the substomatal cavity, and it is described as substomatal vesicle. Hyphae develop from substomatal vesicle and spread in the intercellular spaces. These hyphae send in small, globose haustoria into the host cells to draw nourishment. Up to this stage the reserve food material in the urediniospore itself provides energy for the growth. Once the organic connection is established between the pathogen and the host, the mycelium develops rapidly and aggregates below the epidermis in about four or five days after infection. From this aggregated mycelium urediniospores are produced on small, evanescent stalks. As the spore mass increases, pressure builds up on the epidermis covering the developing pustule, and it eventually ruptures exposing the spore mass. This spot formed by the rupture of epidermis is called pustule. In each pustule thousands of spores are produced. These urediniospores are round to oval in shape, thick walled and measure 20–25 μm in size. Small spines or thickenings may be present on the spore wall. These are called echinulations. Each spore has two nuclei, and there are four or more germ pores in the wall. The urediniospore germinates and germ tubes come out through these germ pores. More than one germ tube may come from a single spore but only one shows further growth. The urediniospores are small, dry, round, thick walled, coloured spores produced in large numbers on infected leaves, and hence, easily come into the air by mechanical disturbances or turbulence of air, and can spread to long distances on wind currents. The long distance dispersal of urediniospores of wheat rust fungi was first reported by Stakman and his coworkers in USA during 1920s and by Prof. K.C. Mehta and his coworkers in India during 1930s and 1940s. Telial stage: By the time the wheat crop reaches harvesting stage, teliospores begin to form in the same pustule in which urediniospores formed earlier. Later formed pustules produce only teliospores. They are formed both on leaves as well as stems. They appear flat dark brown or black. By the time the telial sori or pustules are formed, the crop matures and the leaves dry up, and drop off. Hence they are seen prominently on stems. Therefore, the disease is described as black stem rust. By the time of the telial stage, the crop is in seed filling stage called „dough stage‟. If the telial stage is very severe, seed filling is very poor. The teliospores are smooth, thick walled, two celled and the tip of the upper cell is pointed. Each cell contains two nuclei. They measure 30 – 40 μm in length and 15 – 20 μm in width. They have long persistent stalks to which the spores are firmly attached. Hence, they do not become airborne easily. The telial sorus is shown in Fig. 2.12. Fig. 2.12. Telia and telial sorus in Puccinia. A. Black telial pustule (1) on stem and leaf of wheat. B. Structure of telial sorus. Teliospore germination and formation of basidiospores: After harvesting of wheat, the teliospores remain dormant in dried leaves for a long time. When favourable conditions return the teliospores germinate giving rise to two germ tubes, one from each of the two cells of the spore. The upper cell of the spore possesses a germ pore at the tip and the lower cell has germpore at one side just below the septum. Hence, the germ tube comes from the tip of the upper cell, and laterally from lower cell. As the cells begin to germinate, the two nuclei in the cell fuse and meiosis follows karyogamy producing four haploid nuclei. These haploid nuclei enter into the germ tube. Then transverse septa develop in the germ tube resulting in formation of four uninucleate cells. This structure is described as promycelium. From each cell of the promycelium a small sterigma develops and one basidiospore is formed at the tip of each sterigma. Thus four basidiospores are formed. Of the four basidiospores thus formed, two are of (+) strain and two are of (-) strain. They are released passively and aerially dispersed. They cannot infect wheat plant, but can infect alternate host, the barberry bush. The germination of the teliospore and formation of basidiospores is shown in the Fig. 2.13. Fig. 2.13. Germination of teliospore and formation of basidiospores. A. Formation of promycelium through germination of upper cell of teliospore. B. Formation of promycelium through germination of lower cell of teliospore. 1. Teliospore. 2. Promycelium. 3. Basidiospores. In basidiomycetes, the cell in which karyogamy occurs is described as probasidium and the structure producing basidiospores is described as metabasidium. Hence, the teliospore may be considered as probasidium and promycelium as metabasidium. Stages on barberry: In the life cycle of Puccinia graminis tritici 0 and I stages are formed on barberry plants. Stage „0‟ is called spermagonium stage, and stage „I‟ is called aecial stage. Spermagonium stage: When the basidiospores are deposited on barberry leaves, they germinate under favourable conditions by giving rise to germ tubes which enters the leaf either through stomata or by penetrating the epidermal cells directly. In the leaf the hyphae, initially multinucleate, become multicellular and uninucleate through septa formation. These grow in the intercellular spaces and send in small globose haustoria into the host cells. Some of the basidiospores are of (+) strain and others are of (-) strain. When mycelia of these strains are growing both the strains produce spermagonia on the upper epidermis. The spermagonium, also called pycnium, is a flask shaped structures with round basal part and narrow beak shaped upper part, also called neck. The neck part projects to the outside by piercing through epidermis. The neck part is lined by large number of periphyses. When the spermagonia are fully formed, they appear like yellow or orange coloured pustules on the upper surface of barberry leaves. From the inner wall of the spermagonium long, erect, pointed cells develop and these are called spermatiophores. At the tip of the spermatiophores small, globose, smooth, hyaline unicellular spermatia are formed in chains with basipetal succession. They are also called pycniospores. These spores mixed with honey coloured fluid formed in the spermagonium ooze out through the ostiole. From the entrance of spermagonium, a number of erect hyphae, which are longer than periphyses, develop and project out prominently. These are called receptive hyphae. Spermogonia on barberry leaf and their structure is shown in Fig. 2.14. Fig. 2.14. Spermagonia on barberry leaf and structure of spermagonia. A. Groups of spermagonia on barberry leaf. B. Structure of spermagonium. 1. Spermatia. 2. Receptive hyphae. The rust fungi are heterothallic, and the spermatia and receptive hyphae of the spermagonium are not compatible with each other because they are of the same strain. Hence, the receptive hyphae can receive the nucleus only from a compatible strain formed either on the same leaf or on other leaves or plants. When a compatible spermatium or pycniospore is deposited on receptive hypha, the nucleus from the spermatium enters into receptive hypha. The compatible nucleus that entered the receptive hypha repeatedly undergoes mitotic divisions, and the nuclei spread throughout the mycelium. The two compatible nuclei in each cell of the mycelium pair together. Thus, the dikaryotization occurs. In the life cycle of Puccinia, the occurrence of spermagonium was reported in early 19th century by Persoon (1801), Unger (1833) and others, but its role in dikaryotization was explained for the first time by Craige in 1927. Till then the role of this stage was not known. Hence it is described as „0‟ stage. Hiratsuka and Cummins (1963) reported that about 11 types of spermagonia are formed by Puccinia species. Of these, flask shaped spermagonium is the most common type. Aecial stage: The mycelium formed in barberry leaves due to infection by basidiospores form spermagonia on the upper surface, and the same mycelium form aecial primordia below the lower epidermis. However, until dikaryotization takes place the aecial primordia are inactive. When the entire mycelium becomes dikaryotic, the aecial primordial are stimulated to form aecial cups on the lower surface of the leaf. The aecial cups are surrounded by a clear peridium, and hence they have a clear shape. When the aecial cups rupture the epidermis to expose the aeciospores, the aecial pustules are formed. The aecial pustules always form on the lower surface of the leaf, and occur in concentric rings (Fig. 2.15). Fig. 2.15. Aecial sori on barberry leaf. A. Aecial cups on barberry leaf. B. Structure of aecial sorus. 1. Peridium. 2. Aeciospores. The aeciospores are formed on generative cells arranged in a layer at the base of the aecial cup. The spores are formed in chains with basipetal succession. They are single celled, globose and measure 20-25 μm in diameter. The aecial chains are closely packed in the aecial cups, and due to this the spores may appear angular or polygonal. They disperse through the air. They can infect only wheat leaves, but not barberry leaves. On wheat leaves, the infection due to aeciospores results in the formation of uredinial pustules. Life cycle: In the genus Puccinia, basidiospores are uninucleate and haploid. The cells of mycelium formed in barberry leaves are also at first uninucleate. When the receptive hyphae receive compatible nucleus from spermatium, dikaryotization occurs. The aeciospores formed on dikaryotic mycelium are also dikaryotic. The infection of wheat leaves by aeciospores result in uredinial stage producing dikaryotic urediniospores. The teliospores produced on wheat leaves are also dikaryotic. When the teliospores germinate, karyogamy occurs immediately followed by meiosis. The basidiospores formed on the promycelium are uninucleate and haploid. Thus in the life cycle of Puccinia the diploid stage is confined to the zygotic nucleus only, and dikaryotic stage is the predominant one. The life cycle of Puccinia graminis tritici is shown in the Figs. 2.16 and 2.17. Fig. 2.16. Important Stages in the life cycle of Puccinia graminis tritici. A. + and – strains of basidiospores. B. Spermagonia on barberry leaf. C. Plasmogamy between receptive hypha and pycniospore. D. Aecial cups on barberry leaves. E. Uredinia on wheat leaf. F. Telia on wheat leaf. G. Teliospore. H. Karyogamy and meiosis forming + and – strains of basidiospore. Fig. 2.17. Life cycle of Puccinia. 3. Pleurotus Systematic Position Kingdom: Fungi Division: Basidiomycota Class: Agaricomycetes Order: Agaricales Family: Pleurotaceae Genus: Pleurotus The word “Pleurotus” comes from the Greek word Pleura means side or lateral. The English name of Pleurotus is “oyster mushroom”. It is commonly called as “Dhingri” in Hindi. There are 20 edible species found in both tropical and temperate climatic regions throughout the world. It is a gilled mushroom. All species of Pleurotus are nematophagous (catching nematode and paralysing them with the help of toxin). Pleurotus species are used in mycoremediation of pollutants such as petroleum and polycyclic aromatic compounds. It naturally grows on dead and decaying wood logs or sometime on dying trunks of deciduous and coniferous woods. It may also grow on decaying organic matter. Fig. 2.18: Growth habit. Pleurotus has ability to convert lignocellulosic material (lignin, cellulose, hemicellulose) in to high quality food material. It is one of the most suitable mushrooms which grows on various agriculture, forest and food processing wastes without composting. Spore prints are whitish to grayish. It is very easy to cultivate. The crop cycle completes in 45- 60 days. Less prone to insect, pest and disease attack. Shelf –life of harvested mushrooms is 24-48 hrs. The commonly cultivated species of Pleurotus are P. Sajor-caju, P. ostreatus, P. cornucopiae, P. eryngii, P. fabellatus, P. citrinopileatus, P. djamor, P. cystidiosus, P. columbinus and P. tuber- regium etc. Fig. 2.19: Common species. General Morphology: Mycelium: Mycelia of Pleurotus are pure white in color. Cap: The cap is tongue, shell or spatula shaped, 50-150 mm in diameter. It is white, cream, grey, yellow, pink or light brown in colour. Depending on the species, the surface is smooth and moist with the edge bent downwards. Fig. 2.20: General appearance. Stem: The stipe is short, lateral or sometimes absent. Annulus: absent. Gills: Gills are decurrent type i. e., they run down parallel to the stalk. Fig. 2.21: Fruiting body (Basidiocarp) Basidiospores: Hyaline, 4 haploid spores per basidium, cylindrical, oblong, thin walled, smooth and measures about 12-16 x 4-6 μm. Life cycle: Asexual reproduction in Pleurotus occurs through conidiospores (arthroconidia) produced on conidiophores called coremia. Life cycle of Pleurotus start with haploid spores which are released from the basidium. Spores germinate to form the haploid monokaryotic mycelium termed as the primary mycelium. Normal vegetative cells of this haploid mycelium fuse with another vegetative cell of compatible mating type and they undergo plasmogamy. After plasmogamy create a dikaryotic cell. The repeated nuclear divisions within which along with formation of the clamp connections lead to the establishment of the dikaryotic condition. Dikaryotic mycelium represents the dominant stage of life cycle. Dikaryotic mycelium produces fruiting body of the mushroom. The gills develop below surface of the cap then basidia are formed on gills. In the basidium, both nuclei fuse together and form diploid nuclei (karyogamy) that quickly undergoes meiosis. Each Diploid nucleus yields 4 haploid nuclei of two mating types (+ and -) that develop into basidiospores & repeat the cycle. Fig. 2.22: Life cycle of Pleurotus. Deuteromycota (Anamorphic Fungi)_ General Account The fungi in which sexual stage is absent or not observed so far are included in this group known as anamorphic fungi (Deuteromycotina or Fungi Imperfecti or Mitosporic fungi) (Figs. 2.23, 2.24). These are also called imperfect fungi. It is a large group with more than 1680 genera and 17000 species. Among these fungi 95% were considered as conidial stages belonging to Ascomycetes group. The mycelium of these fungi is similar to that of Ascomycotina fungi. Perfect stages of some fungi are found in Basidiomycetes. Hence, we may consider the Deuteromycetes as ‘conidial stages of Ascomycetes and rarely of Basidiomycetes, whose sexual stages have not been discovered or do not exist.’ Fig. 2.23. Anamorphic fungi. 1. Conidia of Alternaria alternata. 2. Synnemata of Graphium sp. 3. Aspergillus sp. 4. Soil dilution plate with fungal colonies. Fig. 2.24. Anamorphic fungi. 1. Curvularia sp. 2. Nigrospora sp. 3. Drechslera sp. 4. Penicillium sp. However, there is no one to one relation between perfect and imperfect stages. Different conidial fungi may have perfect stages in same genus of Ascomycetes, and species of same form genus may have perfect stages in different genera. Some common examples are shown in Table 2.4. It is clear from the above that the identification and classification of fungi included in Deuteromycetes is based on convenience rather than phylogenetic relationships. Mycelium: The mycelium in most of the imperfect fungi consists of septate, branched hyphae, and is similar to that in ascomycetous fungi. Asexual reproduction mainly occurs through formation of conidia. The hyphae having conidiogenous cells are present on conidiophores. The conidia and conidiophores, their morphology, structure and other characters are considered for identification and classification of these fungi. Table 2.4. Imperfect and perfect stages of some anamorphic fungi. Imperfect stage Perfect stage Aspergillus fumigatus Sartorya fumigata A. nidulans Emericella nidulans Septoria avenae Leptosphaeria avenae Septoria rubi Mycosphaerella rubi Cercospora arachidicola Mycosphaerella arachidis Ramularia fragariae Mycospaerella fragariae Monilia fructigena Sclerotinia fructigena Monilia sitophila Neurospora sitophila Alternaria species Pleospsora species Stemphylium species Pleospora species Drechslera sativa Cochliobolus sativa Monilia sitophila Neurospora sitophila Alternaria species Pleospsora species Stemphylium species Pleospora species Drechslera sativa Cochliobolus sativa Drechslera teres Pyrenophora teres Drechslera turcica Trichometasphaeria turcica Conidia: The conidia formed in this group are highly variable in their morphology structure, size, shape and other characters. Basing on the characters of conidia, Saccardo (1809) divided the conidial fungi into seven sections. The sections of Saccardo have no taxonomic status, but are useful for description of conidia. The sections proposed by Saccardo are as follows. 1. Amerosporae: The single celled conidia which are round, oval or elongated. These spores are called amerospores. Three subsections are recognized. The hyaline conidia are described as Hyalosporae, the coloured spores are called Phaeosporae, and slightly curved spores are called Allantosporae. 2. Didymosporae: Two celled spores are included in this section. These conidia are called didymospores. They are mostly oval in shape. Two subsections are recognized in this section as Hyalodidymae - the hyaline conidia and Phaeodidymae – the coloured conidia. 3. Phragmosporae: Long conidia with transverse septa are included in this section. The conidia in this section are called phragmospores. Two subsections are recognized in this section. They are Hyalophragmiae – with hyaline conidia, and Phaeophragmiae – with coloured conidia. 4. Dictyosporae: The conidia with both transverse and longitudinal septa are included in this section. The two subsections recognized in this section are hyalodictyae – with hyaline conidia, and phaeodictyae – with coloured conidia. The conidia of this group are called dictyospores. 5. Scolecosporae: The conidia which are thin, long and thread like are included in this section. The conidia may be hyaline or coloured. The conidia of this group are called scolecospores. 6. Helicosporae: Helically coiled spores either hyaline or coloured are included in this section. They are described as helicospores. 7. Staurosporae: The conidia with centrally radiating branches, irrespective of the colour are included in this section. They are called staurospores. Different types of conidia observed in Anamorphic fungi are shown in Fig. 2.25. Fig. 2.25. Different types of conidia found in Deuteromycetes. A–D. Amerospores. E-F. Didymospores. G-H. Phragmospores. I-J. Dictyospores. K-L. Scolecospores. M-N. Helicospores. O-P. Staurospores. Conidiophores: Conidiophores are highly variable in their structure. They may occur individually or organized into specialized asexual fruit bodies. The various types are: Micronematous: Conidiophores are similar to vegetative hyphae and not distinct. Macronematous: Conidiophores are distinct from vegetative hyphae. Mononematous: Conidiophores are free, may occur singly or in fascicles. Synnemata: A large number of conidiophores are aggregated along their longitudinal axis, and the structure appears like a broom stick. Sporodochium: It is a cushion shaped structure in which short conidiophores arise from central region. Acervulus: It is typically a flat or saucer shaped bed of generally short conidiophores growing side by side and arise from stromatic mass of hyphae. Long, Sterile, thick walled structures called setae may be present in the stroma interspersing the conidiophores. Pycnidium: They are globose or flask shaped bodies that are lined on the inside with conidiophores. They usually possess an ostiole through which conidia formed on conidiophores ooze out. Different type of asexual fruit bodies found in Anamorphic fungi are shown in Fig. 2.26. Fig. 2.26. Asexual fruit bodies in Deuteromycetes. A. Pycnidium. B. Acervulus. C. Sporodochium. D. Synnema. Conidiogenesis (Conidial ontogeny): The pattern of conidial development is called conidiogenesis. The conidia may be formed singly or in groups. The conidia produced in chains are described as catenulate. The conidia are formed from a conidiogenous cell which may be distinct or integrated with conidiophore. There are different types of specialized conidiogenous cells, and among them phialide and annelid are important. The process of conidiogenesis is mainly of two types, thallic mode, and blastic mode. Of these two types, blastic mode of conidiogenesis is very common and occurs in majority of fungi. Out of 285 dematiaceous hyphomycete genera 275 fungi show blastic mode of conidiogenesis and only 12 show thallic mode of conidiogenesis. Thallic mode of conidiogenesis: In this method, an entire preexisting hyphal cell is transformed into a conidium. The cell may be either terminal or intercalary. The thallic conidia are often described as arthroconidia. Thallic mode is of two types viz., holothallic and enterothallic. 1. Holothallic mode: If all the layers of the wall of conidiogenous cell are involved in the formation of the conidium wall, the conidium is said to be holothallic. 2. Enterothallic mode: If the outer layer of the wall of conidiogenous cell does not become part of the conidium wall, the conidium is described as enterothallic mode. Of the two types, holothallic conidia are more common than enterothallic conidia. Blastic mode of conidiogenesis: Marked enlargement of the recognizable conidial initial takes place before it is delimited from the conidiogenous cell by a septum. Blastic mode is also mainly of two types viz. holoblastic and enteroblastic. 1. Holoblastic method: Both outer and inner walls of blastic conidiogenous cell contribute towards the formation of conidia. In this mode two types are recognised viz. monoblastic and polyblastic. i. Monoblastic mode: If the conidiogenous cell blows out at a single point it is called monoblastic mode. ii. Polyblastic mode: If the conidiogenous cell blows out at several points it is called polyblastic mode. 2. Enteroblastic method: Only inner wall of the conidiogenous cell or no wall layer of conidiogenous cell contributes to the formation of new conidium it is called enteroblastic method of conidiogenesis. In this method three types are recognized viz. tretic, phialidic and annelidic. i. Tretic mode: When conidium develops by protrusion of inner wall through a channel or pore in the outer wall, it is described as tretic mode of conidiogenesis. This type of conidia are also called porospores. e.g. Helminthosporium. ii. Annelidic mode: Conidiogenous cell produces blastic conidia in a basipetal succession, and typically elongates with the production of each conidium. As the first conidium detaches from the conidiogenous cell it leaves a ring like scar on its surface near conidiogenous locus. The numbers of scars indicate the number of conidia produced. Example Scopulariopsis. iii. Phialidic mode: The conidiogenous cell is a flask shaped structure called a phialide. The conidia formed are described as phialospores. Even if a large number of conidia are produced, the phialidic cell retains its shape and size, and does not enlarge. The best examples for this mode are Aspergillus and Penicillium. The types of conidiogenesis methods are shown in Fig. 2.27. Fig. 2.27. Methods of conidiogenesis. A-B. Holoblastic method. C-D. Enteroblastic methods. Morphology and Reproduction of: 1. Alternaria Kingdom: Fungi Division: Deuteromycota Class: Hyphomycetes Order: Moniliales Family: Dematiaceae Genus: Alternaria. Alternaria is one of the important genera of Anamorphic fungi (Deuteromycotina). About 150 species are described in the genus. They are worldwide in distribution. They disperse through air, and produce colonies on various substrata. Most of the species are free living saprophytes, and a few cause important plant diseases. The conidia deposited in house dust cause allergy. Some species cause rotting of stored fruits, vegetables etc. The most important character of the fungus useful for identification is the structure of the conidia which are light to dark coloured, dictyospores with a short or long prominent beak. Alternaria tenuis is the type species of the genus. Various species like A. tenuis, A. tenuissima, A. humicola, etc. are saprophytes. They mainly grow on fallen plant parts. A number of species cause plant diseases mainly leaf spots and blight. They are physiologically weak parasites, causing serious infection on senescent leaves and other plant parts. Some important plant disease causing species of Alternaria, their hosts and disease they cause are given below in the Table 2.5. Table 2.5. Alternaria species pathogenic to crop plants Alternaria species Host crop Disease Alternaria triticina Wheat Leaf spot, leaf blight A. solani Potato, Leaf spot and blight, Brinjal Leaf spot and blight, fruit rot A. brassicae, A. brassicicola Cabbage, Cauliflower, Leaf spot and other cruciferous crops A. ricini Castor Leaf spot A. sesame Sesame Leaf spot A. macrospora Cotton Leaf spot A. longipes Tobacco Leaf spot A. alternata Onion Leaf blight A. mali Apple Leaf blight, Post-harvest fruit rot The mycelium of Alternaria species is composed of light brown coloured septate much branched hyphae. In saprophytic species it grows extensively on the substratum. In plant pathogens, the conidia germinate on host leaf surface and the germ tube enters the leaf either through stomata or by direct penetration. In the host tissue the mycelium grows in the intercellular spaces and sends in haustoria into the host tissue to draw nourishment. Some species, especially those causing blight diseases, produce pathotoxins, kill the host cells and feed on the dead cells. Hence, the pathogenic species are described as nectrotrophs. The toxin produced by Alternaria species is identified as alternaric acid. Alternaria species can be cultured easily on common laboratory media. Potato dextrose agar (PDA) and, Czapek-dox agar are most widely used for culturing of Alternaria species. The colonies develop very quickly on the medium. At first the colonies are white but gradually change into various shades of dark colors such as dark brown, black, grey etc. Colonies of some species are characteristically black with greenish tinge. The colonies are round and slightly raised. Colonies of some species show characteristic zonations also. Numerous conidia are formed in the colonies. Asexual reproduction: After the vegetative mycelium is well developed, branches from some hyphae transform into specialized conidiophores and produce conidia. In pathogenic species the conidiophores penetrate through stomata or epidermis and produce conidia on the leaf surface. The conidiophores are distinct from vegetative hyphae, and they remain as individual structures but not organized into any special asexual fruit bodies. The conidia develop from the tip of the conidiophore by blastic method in acropetal chains. Conidia: The conidia of Alternaria species are multicellular with two clear parts, conidial body and beak. The conidial body is usually club shaped, with relatively big and thick cells. The septa are formed both horizontally and vertically. Hence, these are called dictyospores. At the anterior part there is a beak with uniseriate smaller cells. The beak may be very short or very long (Fig. 2.28). Fig. 2.28. Alternaria symptoms on leaves and conidia. A. Alternaria leaf spot on potato. B. Alternaria blight on wheat leaf. C. Chain of conidia on conidiophore. From the apical cell of the beak, another conidium is formed by budding. The process repeats forming a chain of cells. The length of the beak varies. The arrangement of conidia in chains is described as catenulate. In the conidial chain of Alternaria, the first formed mature conidium is at the base and youngest developing conidium is at the tip. Such type of arrangement of the conidia is described as acropetal. Depending on the length of the conidial chain, the species of Alternaria are divided into three groups. 1. Non catenate species: The species with very long conidia measuring up to 100 to 200 μm, usually do not form conidial chains, and hence are described as non-catenate species. In these species the beak is usually very long compared to the body of the conidium, e.g. A. solani, A. brassicae, A. porri, A. crassa, etc. 2. Brevi catenate species: The length of the conidium in this group is medium, measuring 50- 100 μm, and beak is also medium in length. The conidia are formed in short chains having less than 10 conidia in a chain, e.g. A. longipes, A. sesami, A. amaranthi, etc. 3. Longi catenate species: These species produce short conidia with a short beak of a few cells. They form very long chains with 10 to 15 conidia or more, e.g. A. tenuis, A. brassicicola, etc. Dispersal of Alternaria conidia: The conidia of Alternaria species are dry without any slime and hence, they can easily come into the air and disperse. They are not released actively but come into air by the turbulence of the air or any other mechanical disturbances. In the parasitic species, the conidia are formed on the leaves, and in saprophytic forms they are formed on the substratum in large numbers. The wind speed and turbulence are usually more at noon or early evening hours, and hence the conidia also usually disperse more during day time. Aerobiological surveys conducted in different parts of the world also revealed that the conidia of Alternaria are important constituents of air spora during day time. Germination of conidia: The airborne conidia when deposited on a substratum with some organic matter and moisture, readily germinate under favourable conditions. Temperatures in the range of 25-30°C and 90-100% relative humidity or moisture are favourable for conidial germination. The germ tubes may arise from any cell of the conidia. Usually 5–6 cells of a conidium germinate at a time. In free living species, the germ tubes gradually elongate to form mycelium. In pathogenic species the germ tubes enter the host tissue and mycelium develops. Sexual stage: Most of the species do not show sexual reproduction. However, a few species may show sexual stage occasionally. The sexual stage of the Alternaria species has been identified as belonging to the genus Pleospora of Ascomycetes. 2. Colletotrichum Kingdom: Fungi Division: Deuteromycota Class: Coelomycetes Order: Melanconiales Family: Melanconiaceae Genus: Colletotrichum. One of the most commonly encountered form genera in Melanconiales is Colletotrichum. Over 1000 species were described in the genus Colletotrichum based on host species, but based on cultural studies Von Arx (1957) recognized only 20 species and made rest of the species as synonyms. For example about 600 synonyms exist for Colletotrichum gloeosporioides. Some authors recognize form genus Gloeosporium, and distuinguish it from Colletotrichum on the basis that the acervuli of Gloeosporium lack setae. The current tendency is to combine these two genera under Colletotrichum. Sutton (1980) of Commonwealth Mycological Institute recognized 22 species in the genus Colletotrichum based on cultural characters. 0 Several species of Colletotrichum are parasitic on plants causing diseases of leaves, young twigs, fruits and vegetables. The diseases caused by Colletotrichum are commonly called anthracnoses, because of soot like black colour of the lesions due to the presence of numerous dark setae. Some important species are given in Table 2.6. Mycelium is at first hyaline turning dark brown at maturity. It is septate, highly branched. The contents also become denser with age. In culture, the thick walled mycelium aggregate to form a structure resembling sporodochium from which conidiophores develop. In the host, mycelium occurs in the intercellular spaces and aggregates below the epidermis to form a saucer shaped structure called acervulus. In fairly old mycelium, some thick walled dark brown, irregular or angular cells develop with one or more large oil drops within them. These are formed in either terminal or intercalary cells of hyphae. These get detached and are capable of germination after a long period of rest. Thus these behave as chlamydospores. Table 2.6. Some Colletotrichum species causing disease. Pathogen Disease Colletotrichum falcatum Red rot of sugarcane C. lagenarium Anthracnose of cucurbits C. lindemuthianum Anthracnose of beans C. gloeosporioides Anthracnose of mango, and various hosts C. gossypii Boll rot of cotton C. graminicola Red rot of sorghum and other grasses C. circinans Onion smudge C. capsici Fruit rot and die back of chillies C. musae Anthracnose of banana C. coffeanum Coffee berry disease C. lini Seedling blight of flax Reproduction: It occurs by means of conidia. The fruiting structures are acervuli. In the host, the conidia are formed in acervuli which develop from the stromatic mass of hyphae formed just beneath the epidermis. In grains, this mass is formed just below the pericarp. Asexual reproductive structures are shown in the Fig. 2.29. Fig. 2.29. Acervulus and conidia of Colletotrichum graminicola. A. Portion of host leaf showing acervuli. B. An acervulus. C. Conidiophores and conidia. The acervulus is a saucer shaped structure, and is surrounded by stiff dark brown, unbranched hairs called setae. The setae measure 100-200 μm in length and 5-10 μm in width. The conidiogenous cells form a closed packed palisade like layer of phialides. The phialides are very small producing phialoconidia at their apex. The conidia are aseptate, single celled, fusiform, slightly curved or sickle shaped, hyaline or slightly pink coloured with rounded ends. They vary in size but on an average they measure 15- 30 μm in length and 3- 6 μm in width. They accumulate under moist conditions as glistening mass held in place by setae. The conidia germinate by two polar germ tubes. They enter the host through stomata or directly, and on culture media grow as mycelium. If the conditions are not favourable for infection, the germ tube shows limited growth and produces globose appressoria which become thickened and function like chlamydospores. Perfect stage: The perfect stage for some species is found in ascomycetous genus Glomerella, of family Sphaeriaceae class Pyrenomycetes. Some species showing perfect stages are as follows (Table 2.7). Table 2.7. Conidial and perfect stages of some Colletotrichum species. Conidial stage Perfect stage C. gloeosporioides Glomeralla cingulata C. lindemuthianum G. cingulata C. gossypii G. gossypii C. graminicola G. graminicola C. falcatum G. tucumanensis The genus Glomeralla produces typical flask shaped or globose, ostiolate perithecia. Asci are clavate and irregularly biseriate. The ascospores are hyaline and single celled. Oomycota Introduction The phylum Oomycota, alternatively called Peronosporomycetes (Dick, 2001a), currently comprises some 800_1000 species (Kirk et al., 2001). The Oomycota as a whole have been resolved as a monophyletic group within the kingdom Straminipila in recent phylogenetic studies (e.g. Riethmu‥ller et al., 1999; Hudspeth et al., 2000), although considerable rearrangements are still being performed at the level of orders and families. A scholarly treatment of the Oomycota has been published by Dick (2001a) and will remain the reference work for many years to come. Because of the outstanding significance of Oomycota, especially in plant pathology, we give an extended treatment of this group. Vegetative hypha The vegetative hypha Although some members of the Oomycota grow as sac-like or branched thalli, most of them produce hyphae forming a mycelium. Oomycota are now known to be the result of convergent evolution with the true fungi (Eumycota), and their hyphae differ in certain details. However, the overall functional similarities are so great that they provide a persuasive argument for the fundamental importance of the hypha in the lifestyle of fungi (Barr, 1992; Carlile, 1995; Bartnicki-Garcia, 1996). Much physiological work has been carried out on hyphae of Oomycota, and the results have a direct bearing on our understanding of the biology of the Eumycota. Like them, the hyphae of Oomycota display apical growth and enzyme secretion, ramify throughout the substratum by branching to form a mycelium, and can show morphogenetic plasticity by differentiation into specialized structures such as appressoria or haustoria. The hyphae of Oomycota are coenocytic, i.e. they generally do not form cross-walls (septa) except in old compartments or at the base of reproductive structures. The cytoplasm is generally coarsely granular and contains vacuoles, Golgi stacks, mitochondria and diploid nuclei. The apex is devoid of organelles other than numerous secretory vesicles. These are not, as in the Eumycota, arranged into a Spitzenkorper because the microvesicles which contain chitin synthase and make up the Spitzenkorper core are lacking. This is in line with the general absence, with a few exceptions, of chitin from the walls of Oomycota; instead, cellulose, a crystalline b-(1,4)- glucan, contributes the main fibrous component. As in the Eumycota, these structural fibres are cross-linked by branched b-(1,3)- and b-(1,6)- glucans, although the biochemical properties of the glucan synthases seem to differ fundamentally between those of Eumycota on the one hand and those of Oomycota and plants on the other (Antelo et al., 1998). Other biochemical differences include the lysine synthetic pathway (DAP in plants and Oomycota; AAA in true Fungi) and details of sterol metabolism (Nes, 1990; Dick, 2001a). The mitochondria of Oomycota are indistinguishable by light microscopy from those of the Eumycota, but when viewed with the transmission electron microscope they have tubular rather than lamellate cristae. The vacuolar system of Oomycota is also unusual in containing dense- body vesicles or ‘fingerprint vacuoles’ which consist of deposits of a phosphorylated b-(1,3)- glucan polymer, mycolaminarin. Mycolaminarin may serve as a storage compound for carbohydrates as well as phosphate (Hemmes, 1983), and the polyphosphate storage deposits which are typically found within vacuoles of true Fungi are absent from vacuoles of Oomycota (Chilvers et al., 1985). Apart from that, however, vacuoles of Oomycota share many features with those of true Fungi, including the membranous continuities which often link adjacent vacuoles and provide a means of transport by peristalsis (Rees et al., 1994). Cytoplasmic glycogen granules, which are one of the major carbohydrate storage sites in Eumycota, are absent from hyphae of Oomycota (Bartnicki-Garcia & Wang, 1983). The zoospore The Oomycota are characterized by motile asexual spores (zoospores) which are produced in spherical or elongated zoosporangia. They are heterokont, possessing one straminipilous and one whiplash-type flagellum. Two types of zoospore may be produced and, if so, the auxiliary zoospore is the first formed. It is grapeseedshaped, with both flagella inserted apically (Fig. 2.30), and it encysts soon after its formation. Encystment is by withdrawal of the flagella, so that a tuft of tripartite tubular hairs (TTHs) is left behind on the surface of the developing cyst (Dick, 2001b). The cyst germinates to give rise to the principal zoospore, which is by far the more common type and also the more vigorous swimmer. This typical and readily recognized oomycete zoospore is uniform in appearance across the phylum (Lange & Olson, 1983; Dick, 2001a). In species lacking auxiliary zoospores, the principal zoospore is usually produced directly from a sporangium. It is kidney-shaped, with the flagella inserted laterally in a kinetosome boss which in turn is located within the lateral groove (Fig. 2.30). Encystment is initiated by the shedding, rather than withdrawal, of the flagella; no tufts of TTHs are left on the cyst surface (Dick, 2001a). Fascinating insights into the cytology of zoospore encystment have been obtaiined from several species (see Fig. 5.24). At the onset of encystment, adhesive and cell wall material is secreted by the synchronized fusion of pre-formed storage vesicles with the zoospore plasma membrane (Hardham et al., 1991; Hardham, 1995), thereby providing a rare example of regulated secretion in fungi. Constitutive secretion by growing hyphal tips is more commonly associated with their mode of life. Some members of the Oomycota have no motile spore stages but can be readily related to groups still producing them. Fig 2.30: Asexual reproductive stages in Saprolegnia. (a) Auxiliary (primary) zoospore. (b) Principal (secondary) zoospore. Schematic drawings, based partly on Dick (2001a). Sexual reproduction The life cycle of the Oomycota is of the haplomitotic B type, i.e. mitosis occurs only between karyogamy and meiosis. All vegetative structures of Oomycota are therefore diploid. This is in contrast to the Eumycota in which vegetative nuclei are usually haploid, the first division after karyogamy being meiotic. Sexual reproduction in Oomycota is oogamous, i.e. male and female gametangia are of different size and shape. Meiosis occurs in the male antheridia and in the female oogonia, and is followed by plasmogamy (fusion between the protoplasts) and karyogamy (fusion of haploid nuclei). Numerous meioses can occur synchronously, so that true sexual reproduction can actually happen within the same protoplast (Dick, 1990a). Heterothallic species of Oomycota display relative sexuality, i.e. a strain can produce antheridia in combination with a second strain but oogonia when paired against a third. Steroid hormones play an important role in sexual reproduction. Fig. 2.31. Schematic drawing and terminology of sexual reproductive organs in the Oomycota. Modified from Dick (1995). The mature oospore contains three major pools of storage compounds (Fig. 2.31; Dick, 1995). The oospore wall often appears stratified, and this is due in part to a polysaccharide reserve compartment, the endospore, which is located between the plasma membrane and the outer spore wall (epispore). Upon germination, the endospore is thought to coat the emerging germ tube with wall material, and some material may also be taken up by endocytosis. A large storage vacuole inside the oospore protoplast is called the ooplast. It arises by fusion of dense-body vesicles and, like them, contains mycolaminarin and phosphate. Dick (1995, 2001a) speculated that the ooplast contributes membrane precursor material during the process of oospore germination. The third storage compartment consists of one or several lipid droplets which provide the endogenous energy supply required for germination. Fig 2.32. Life cycle of Saprolegnia. Vegetative hyphae are diploid and coenocytic. Asexual reproduction is by means of diplanetic (auxiliary and principal) zoospores. The principal zoospore state is polyplanetic. Saprolegnia is homothallic, and sexual reproduction is initiated by the formation of antheridia and oogonia. For simplicity, only a single nucleus is shown in each of the oospheres and in the antheridium. Each oogonium contains several oospheres. Karyogaimy occurs soon after fertilization of an oosphere by an antheridial nucleus. The oospore may germinate by means of a germsporangium (not shown) or a hyphal tip. Open and closed circles represent haploid nuclei of opposite mating type; diploid nuclei are larger and half-filled. Key events in the life cycle are meiosis (M), plasmogamy (P) and karyogamy (K). Morphology, Reproduction and Life cycle of: A. Phytophthora Kingdom: Straminipila Division: Oomycota Class: Oomycetes Order: Pythialaes Family: Pythiaceae Genus: Phytophthora The name of the genus indicates that it is a plant destroyer (phyto = plant; phthora = destroyer), and indeed some species are certainly destructive parasites. About 40 species are known in the genus, and of them Phytophthora infestans is the first described and the best known. While some species may live saprophytically as water moulds, the majority are pathogenic forms probably have no prolonged free living saprophytic existence. It is the causal organism of late blight disease of potato and caused Irish famine of 1845-46. The species of the genus are having a wide host range. P. infestans is confined to the members of Solanaceae, but others have much wider host range. For example, P. cactorum has been recorded from over 40 families of flowering plants causing a variety of diseases such as damping-off, root rot and fruit rot. Other important species in the genus and the diseases they cause are given below. Pathogen Disease Phytophthora antiquorum Blight of colocasia P. arecae Koleroga of areca palms P. cryptogea Foot-rot of tomato seedlings P. erythroseptica Pink rot of potato P. fragariae Red core of strawberries P. palmivora Bud rot of palms P. nicotianae Seedling blight of castor Mycelium: It is aseptate, coenocytic, producing branches at right angles, often constricted at their point of origin. Within the host, mycelium is intercellular but finger like haustoria are formed which penetrate the host cells. Asexual Reproduction: It is by sporangial formation. The sporangiophores emerge through the stomata. The first formed sporangium is terminal, and the hyphae bearing it may push it to one side and proceed to form another sporangium and the process is repeated continuously. Thus the first formed sporangium is at the base and youngest at the top. The sporangiophores show unlimited growth. The mature sporangium has a terminal papilla and it is separated from the sporangiophore by a thickening of wall material which forms a basal plug. In terrestrial forms, the sporangia are detached, possibly aided by hygroscopic twisting of the sporangiophore by drying and disperse by wind before germinating, but in aquatic forms zoospore release commonly occurs while the sporangia are still attached. In these aquatic species, internal proliferation of sporangia may occur. The germination of sporangia may be either direct by germ tube or by forming zoospores. Below 15oC uninucleate zoospores are produced while above 20oC multinucleate germ tubes arise. With increasing age, sporangia lose their capacity to produce zoospores. When zoospores are formed, they are typically differentiated within the sporangium, and not in vesicle as in Pythium. The uninucleate, laterally biflagellate zoospores swim for a time, often attracted chemotactically to host tissue, then encyst and germinate by germ tube, but repeated emergence (polyplanetism) has occasionally been reported. Sexual Reproduction: Some species of Phytophthora are homothallic and form oospores readily in culture derived from single uninucleate zoospores. e.g. P. cactorum, P. erythroseptica. Majority of species, e.g. P. infestans, P. palmivora etc., are heterothallic, and normally form oospores only when different isolates are paired together i.e. they are self incompatible. When conditions are favourable for sexual reproduction, two types of sex organs viz. antheridia and oogonia are formed, and the reproduction is oogamous type. Oogonium is a bigger and spherical structure while antheridium is a smaller and club shaped structure. Two distinct types of antheridial arrangement are found. In P. cactorum and others, antheridia are laterally attached to oogonium, and it is called paragynous type. In P. infestans, P. erythroseptica and others, the oogonia during development penetrate the antheridium, grow through it, inflate and develop into a spherical oogonium, with antheridium persisting as a collar around the base of the mature oogonium. This type of arrangement is called amphigynous type. Fig. 2.33. Life cycle of Phytophthora infestans. B. Albugo Kingdom: Straminipila Division: Oomycota Class : Oomycetes Order : Peronosporales Family : Albuginaceae There are about 30 species in the genus Albugo. This genus is also called Cystopus. The genus was first described by C.H. Persoon in 1801 as Albugo. Later, in 1840s Levellie described the genus as Cystopus. Since the genus name was first published validly as Albugo, it should be treated as Albugo, and Cystopus is a later synonym. All the species in the genus are obligate parasites on higher plants. On the host plants they produce white rust disease. Due to the fungal infection white blisters are formed on host leaves and stem. The blisters appear as pustules formed by rust fungi but white in colour. Hence the disease is called white rust disease. In the blisters a large number of sporangia are formed in a mass. Due to the incidence of large number of pustules on leaves, the photosynthetic area is reduced, and the formation of blisters on stem weakens it. The fungus may also infect the inflorescence during severe infections. Because of infection the peduncle becomes thick, and floral parts become green leaf like structures. The species of Albugo infecting the members of the families like Amaranthaceae, Cruciferae, Convolvulaceae etc. are given below. Species Host(s) Albugo bliti Amaranthaceae A. candida Cruciferae A. ipomoeae- panduranae Evolvulus alisinoides A. evolvuli Sweet potato A. oxidentalis Spinach species A. platensis Boerhaavia species A. portulacae Portulaca species Mycelium: All the species of Albugo are obligate parasites. Hence, vegetative growth occurs only in host tissue. The hyphae are aseptate, hyaline, branched filaments. They grow in the intercellular spaces in the host tissue. The hyphae send in small, globose structures into the host tissue to draw nourishment. They are called haustoria. Asexual Reproduction: Albugo species reproduce asexually by production of sporangia and sexual reproduction by gametangial contact. The details of reproduction are presented in Fig. 2.34. The mycelium grows in the intercellular spaces of the host tissue. After a period of growth for 4 or 5 days after infection, mycelium aggregates below the epidermis. The sporangiophores are produced in a layer below the epidermis. The sporangiophores are short, erect, unbranched, cylindrical or club shaped, and produce sporangia at the tip in chains. The tip of the sporangium bulges into a globose structure with accumulation of protoplast with 5-8 nuclei. Then it is cut off from the sporangiophore with formation of a septum. The process repeats a number of times to produce a chain of sporangia, with first formed sporangium at the apex and youngest at the base. Such an arrangement of sporangia is described as in basipetal succession. The sporangiophore, though shows continuous growth producing sporangia, they remain almost constant in size and shape. As the sporangia are formed in large numbers and accumulate below the epidermis, the epidermis, at the region of the sporangial mass, bursts open because of the internal pressure exposing the sporangia. This appears like a pustule. Since the pustules formed by the sporangia are white in colour, and resemble the pustule formation in rust disease, the infection caused by Albugo is described as white rust. The sporangia formed in a chain are attached to one another and usually held together by slime masses. After the exposure of the sporangia, the slime evaporates, and the sporangia become dry and separated. Then they are dispersed through the air like conidia. When the sporangia get are deposited on a susceptible host surface, they germinate under favourable conditions. Two types of sporangial germination have been observed. 1. Zoospore production: When the temperature is 15oC or below and there is abundant moisture or a thin film of water on the host surface, the protoplast of the sporangium cleaves to produce biflagellate zoospores, and they are released by splitting of the sporangial wall. The zoospores are kidney shaped and laterally biflagellate. After a period of motility, the zoospores encyst. The cyst germinates giving rise to an infection peg which enters the host leaf through stomata. 2. Direct germination: when the temperature is 20oC or more and there is no moisture on the host surface, the sporangia do not produce zoospores, but germinate directly to produce a germ tube which enters the host leaf through stomata. Sexual reproduction: Albugo species are homothallic. The male gametangium (antheridium) and female gametangium (oogonium) form on the same thallus and carry out sexual reproduction. The gametangia are formed on the tips of the vegetative hyphae growing in the host tissue. Oogonium is globose, multinucleate at first with 6–12 nuclei. These nuclei undergo repeated divisions to form about 200 nuclei. The cytoplasm in the oogonium is then differentiated into a clear, dense central part called centroplasm or ooplasm, and surrounding less dense cytoplasm called periplasm. The antheridia are relatively small structures formed on separate hyphae. They are club shaped. At maturity the oogonium and antheridium occur side by side. This condition is described as paragynous. Depending on the activity of the nuclei in oogonium, two types of conjugation occur. In some species (e.g. Albugo candida), both oogonium and antheridium contain only one active nucleus each. At the place of gametangial contact, a fertilization tube develops from the antheridium and enters the oogonium and releases the active nucleus into centroplasm. Then the two active nuclei fuse producing diploid zygotic nucleus. In some species (e.g. Albugo bliti) both antheridium and oogonium are multinucleate. In the oogonium, at first, there may be about 300 nuclei, and among them 40 to 50 remain in centroplasm and rest move into the periplasm during the differentiation. Then the nuclei in the centroplasm undergo further division to form about 80 to 100 nuclei. In the antheridium there are about 30-35 nuclei in the beginning and they repeatedly undergo division to form about 100 nuclei. All the male nuclei are passed into centroplasm of oogonium through fertilization tube. The male and female nuclei pair together and karyogamy occurs producing a large number of zygotic diploid nuclei. Since all the zygotic nuclei occur in the single oospore that develops after karyogamy, it is called compound oospore. In both the methods of fertilization, after the formation of diploid nuclei a thick wall is formed around the centroplasm which transforms into oospore. The oospore wall is very thick, and two layers can be distinguished, the outer exospore and inner endospore. The exospore shows various types of thickenings, which may be tuberculate, porous, reticulate etc. The type of thickening is useful in identification of different species in the genus. As in other oomycetes, in the genus Albugo also meiosis occurs in gametangia. Hence the vegetative mycelium is diploid. Germination of oospores: The oospores are resting structures and after a long period of rest they germinate under favourable conditions. The germination of oospores occurs by two methods. Both the types may be found in the same species (e.g. Albugo candida). The outer layer of the oospore (exospore) cracks open and inner endospore comes out as a vesicle. The protoplast of the oospore is transferred into the vesicle, and zoospores are formed in the vesicle. The zoospores are released after the disintegration of the vesicle membrane. During germination oospore directly gives rise to a short germ tube, and a vesicle is formed at the tip of the vesicle. The protoplast of the oospore is transferred into the vesicle, and zoospores are formed in the vesicle. Life cycle: In Albugo species vegetative mycelium is diploid. Meiosis occurs in gametangia to produce haploid nuclei. The fusion of the haploid nuclei results in diploid stage. The life cycle of Albugo is shown in the Figs. 2.34 and 2.35. Fig. 2.34. Life cycle of Albugo candida. A. Vegetative hyphae on host cell. B. Sorus with sporangia. C. Sporangium. D. Liberation of zoospores. E. Zoospores. F. Encystment of zoospores. G. Germination of cysts. H. Gametangial contact. I. Fertilization tube releasing male nuclei into oogonium. J. Nuclear fusion. K. Oospore formation in oogonium. L. Germination of oospore. M. Motile zoospores. N. Germination of zoospores. Fig. 2.35. Life cycle of Albugo. Introduction to Plant Pathology Plant pathology studies plant diseases and helps plants survive unfavorable conditions and parasitic microorganisms. This field is challenging, vital, and fascinating in its own right while also serving the practical goal of safeguarding food for humans and animals. Plant diseases hinder food production by preventing cultivation, damaging crops during growth, or reducing yields before harvest. Alongside entomology and weed science, plant pathology works to address annual global crop losses, estimated between 31% and 42% due to diseases, insects, and weeds. Of these, plant diseases alone account for 14.1%, translating to $220 billion annually. Post-harvest losses add another 6–12%, especially in developing countries lacking resources like refrigeration. These figures exclude losses from environmental factors like drought, freezes, and pollution but highlight the plight of millions in poor regions suffering malnutrition and hunger due to crop diseases. Lost income from crop failures often drives people to overcrowded cities, worsening socioeconomic challenges. Efforts to control plant diseases involve measures like pesticides, soil fumigation, and crop rotation. While necessary, these approaches limit arable land, crop diversity, and food production, and introduce harmful chemicals into the environment. Plant pathology thus strives to maximize food production while minimizing environmental and human impacts, balancing agricultural needs with sustainability. Definition: Plant Pathology or Phytopathology or Fitopathologia is the branch of agricultural or biological science which deals with the plant diseases, their causes, etiology and their management. » The science of plant pathology is the study of all aspects of disease in plants, including causal agents, their diagnosis, physiological effects, population dynamics and control. » Phytopathology (Greek Phyton = plant + pathos – disease, ailments + logos = discourse, knowledge) is study of suffering plants. Plant Pathology has the following major objectives: 1. To study biotic (living) and abiotic (non-living and environmental) causes of diseases or disorders or deficiencies. 2. To study the mechanism of disease development by pathogens. 3. To study the plant (host) pathogen interaction in relation to environment. 4. To develop methods of management of plant diseases. Scope of Plant Pathology: Plant Pathology (phytopathology) is defined as the study of the organisms (infectious organisms) and environmental conditions (physiological factors) that cause disease in plants, the mechanisms by which disease occurs, the interactions between these causal agents and the plant (effects on plant growth, yield and quality). Plant pathology also involves the study of pathogen identification, disease etiology, disease cycles, economic impact, plant disease epidemiology, plant disease resistance, how plant diseases affect humans and animals, pathosystem genetics, and management of plant diseases. It also interfaces knowledge from other scientific fields such as mycology, microbiology, virology, biochemistry, bioinformatics, etc. Moreover, plant pathology is directly relevant to man’s need to grow enough food and fiber to sustain civilization. Development of Plant Pathology Plant pathology is a broad scientific field that combines knowledge of plant biology, production, microbial biology, and ecology to study plant diseases. It focuses on understanding how diseases start, spread, and can be prevented or managed. The term "plant pathology" literally means the study of plant suffering (pathos = suffering, logy = study). The science of plant pathology became essential as humans transitioned from foraging to cultivating crops, encountering plant diseases that ranged from mild to devastating. Early beliefs attributed diseases to bad weather, toxic air, or divine intervention due to the lack of understanding of microorganisms, which were first observed in the 17th century. However, it wasn’t until the 1840s potato late blight epidemics in Europe and Ireland that plant pathology began to emerge as a science. These devastating outbreaks highlighted the need to understand and manage plant diseases. Julius Kühn published the first textbook of plant pathology within 15 years of the late blight epidemics, linking plant diseases to both parasitic and non-parasitic causes. The germ theory of disease, which proved microorganisms cause disease, became the foundation of plant pathology. By the late 19th and early 20th centuries, the science rapidly developed as societies sought ways to control plant diseases and improve crop productivity. The germ theory, stating that living organisms cause diseases, was pivotal in the development of plant pathology. Although early evidence, like Prevost's work on wheat smut in 1807, pointed to this theory, it wasn’t widely accepted until Anton deBary’s conclusive experiments on potato late blight in 1861. DeBary demonstrated that Phytophthora infestans was the cause of late blight by inoculating plants and comparing them to healthy controls, confirming infection as the source of the disease. DeBary’s work established the germ theory for fungal pathogens and inspired similar discoveries for bacteria and viruses. Burrill and Arthur proved bacteria caused fire blight in the late 19th century, while Mayer, Ivanowski, and Beijerinck later demonstrated viruses as plant pathogens, though their exact nature wasn’t understood until the electron microscope was invented in 1931. Beijerinck described viruses as a “contagious living fluid,” coining the term "virus" from the Latin word for poison. The germ theory revolutionized plant pathology by linking microbes to plant diseases, enabling scientists to develop effective management strategies. Anton deBary’s research earned him the title of "Founder of Plant Pathology," and the field continues to play a crucial role in ensuring global food security. Terms used in Plant Pathology A basic understanding of terms and definitions is essential for diagnosing plant problems, conducting research, and communicating effectively in plant pathology. While initially daunting, familiarity with the concepts and vocabulary will improve through experience and practice. Key Terms in Plant Pathology 1. General Definitions Plant Pathology: The study of plant diseases and disorders (path = suffering, ology = study). Etiology: The study of the cause of a disease. Pathogen: A living or non-living agent that causes disease. Pathological: Referring to diseased conditions. Pathogenicity: The ability of a pathogen to cause disease. 2. Types of Diseases Plant Disease: Abnormal structure or function in a plant due to continuous irritation from pathogens or environmental factors. Infectious (Biotic) Disease: Caused by living agents like fungi, bacteria, or viruses. Noninfectious (Abiotic) Disease: Caused by non-living factors such as weather, chemicals, or nutrient imbalances. Environmental Disease: Results from unfavorable conditions like extreme temperature, moisture, or air pollution. 3. Causes and Agents Causal Agent: Any factor (biotic or abiotic) responsible for disease or injury. Virulent Pathogen: Highly aggressive and disease-causing. Avirulent (non-virulent) Pathogen: A less aggressive variant that causes minimal or no disease. 4. Parasites and Their Types Parasite: An organism that lives on or in another organism for nutrition. Obligate Parasite: Fully dependent on a living host. Facultative Parasite: Can survive as a parasite or saprophyte. Saprophyte: Feeds on dead organic material. Autotroph: An organism (like a plant) that produces its own food via photosynthesis. 5. Host-Pathogen Interaction Host: The plant or organism harboring a parasite or pathogen. Host Range: The variety of plants a pathogen can infect. Resistance: The ability of a plant to prevent or overcome disease. Tolerance: A plant’s ability to survive or function despite being infected. Susceptibility: The opposite of resistance; inability to prevent disease. 6. Symbiosis Symbiosis: A mutually beneficial relationship between organisms. Example: Mycorrhizal fungi and plant roots. Fungi provide nutrients; plants supply carbohydrates. Other Examples: Nitrogen-fixing bacteria in legumes, lichens (fungi + algae/cyanobacteria). 7. Disease Identification Signs and Symptoms Signs: Physical evidence of the causal agent, such as spores, pests, or residue. Symptoms: Visible plant responses like chlorosis, wilting, or galls. Syndrome: The collective symptoms of a disease. Diagnosis and Factors Diagnostics: Identifying the cause of plant disorders. Environmental Influence: Conditions like temperature and moisture affect host susceptibility and pathogen activity. 8. Disease Cycles and Transmission Life Cycle Disease Cycle: Includes pathogen reproduction, spread, and effects on the host. Primary Inoculum: Initial infection source (e.g., spores). Secondary Inoculum: Infections during the same growing season. Disease Classification Polycyclic Disease: Completes multiple life cycles in one year. Monocyclic Disease: Completes one life cycle per year. Transmission and Dissemination Transmission: Active transfer by insects, grafting, or mechanical means. Dissemination: Spread over long distances via wind, rain, or irrigation. Vectors: Organisms like insects and nematodes that carry pathogens. 9. Infection and Colonization Infection: Establishment of a pathogen within the host. Colonization: Pathogen growth and reproduction in the host. Infestation: Overrunning of the host surface by pests, without infection. 10. Epidemics and Epidemiology Epidemic: Widespread increase of disease over time. Endemic: Disease permanently present in a specific area. Epidemiology: Study of factors affecting disease spread. Concept and Classification of Plant Diseases Effective communication relies on the assumption that all parties involved understand one another. In science, it is particularly important to have a clear understanding of the terms used within a field to ensure effective communication. This is especially crucial when terms, such as “disease,” can have multiple definitions. If asked to define disease, it is unlikely that any two individuals in a group would provide identical definitions. However, there would likely be agreement on key components that any definition of disease should include. Broadly, disease refers to an abnormal condition or something that causes an organism to deviate from a healthy state. Importantly, there is no clear line between health and disease, and recognizing a diseased plant often requires the expertise of a trained diagnostician. Most definitions of disease imply a clear understanding of what constitutes a healthy, normally functioning plant. For example, the American Phytopathological Society defines disease as “the abnormal functioning of an organism,” while the National Academy of Sciences (1968) defined plant disease as “a harmful alteration of the normal physiological and biochemical development of a plant.” While these are acceptable definitions, for the purposes of this discussion, additional components are required. Here, plant disease is defined as a condition detrimental to the normal development of a plant, resulting from the continuous interaction between the plant and a causal agent, leading to the production of symptoms. There are four essential components of this definition, which distinguish disease from other harmful conditions affecting plants. First, disease is detrimental to a plant’s development and may affect any stage, whether vegetative or reproductive. For instance, some diseases, such as damping-off, occur only in seedlings, while others affect mature or senescing plants, or persist throughout the plant’s life. However, this component does not differentiate disease from the negative effects of physical or chemical environmental factors or damage caused by pests like insects or rodents. Second, disease results from a continuous interaction between the plant and a pathogen. Unlike instantaneous injuries, disease is a dynamic process that develops over time. Even when symptoms appear suddenly, the underlying disease process has been progressing long before the symptoms become visible. This continuous, progressive nature of disease differentiates it from injuries. Third, disease is caused by a specific causal agent, typically a pathogen – an organism capable of causing disease. While diseases are often attributed to a single pathogen, some are caused by a combination of pathogens. The involvement of a causal agent also distinguishes disease from injury and implies that diseases are contagious, capable of spreading to neighboring plants, unlike injuries. Finally, disease leads to the production of symptoms, which serve as evidence that a plant’s normal development or appearance has been altered. Symptoms can range from minor, almost undetectable changes to severe manifestations, including plant death. While symptoms help in identifying disease, they are not unique to it, as injuries can also produce similar visible effects. By understanding these four components, a conceptual framework for analyzing plant diseases can be developed. In a narrow sense, biotic organisms cause all plant diseases. However, in a broader sense, abiotic factors like nutrient deficiencies, pollution, or environmental stressors can also result in conditions referred to as disorders. Unlike diseases, disorders typically lack a continuous interaction with a causal agent and are not contagious. While understanding abiotic disorders is important for diagnosing plant problems, the study of plant pathology primarily focuses on diseases caused by living pathogens. Recognizing these distinctions is key to managing and mitigating the complex challenges associated with plant health. General symptoms and signs A symptom is the visible expression of a disease. Symptom names are often descriptive of the primary abnormality observed, such as leaf spot, wilting, or stunting. Some diseases, however, produce a syndrome—a series of symptoms characteristic of a particular disease. For instance, yellowing, wilting, and death of all above-ground plant parts typically indicate root issues, such as those caused by root rot diseases. Symptoms are frequently part of a disease’s common name. For example, black root rot describes a disease resulting in blackened and decayed roots. However, common names can sometimes be misleading. For instance, the peanut disease caused by Sclerotium rolfsii is referred to both as southern stem rot and white mold. Plants have limited ways to express the harmful effects of diseases or disorders, so symptoms are categorized based on the damage caused, the affected plant part, and the timing relative to plant development. While some diseases are identifiable by specific symptoms, others require additional information for accurate diagnosis. One of the most common symptoms of plant diseases is necrosis, which involves the browning or blackening of tissues due to cell death. Necrosis includes several specific symptom types. A localized area of necrosis is called a lesion. The most commonly observed necrotic lesion is the leaf spot, a defined area of necrosis on a leaf. These spots may have distinctive or nondescript appearances and usually have a specific size and shape. Another necrotic symptom is a canker, a sunken area on the plant’s main stem or trunk, often with raised margins. Anthracnose is a similar sunken lesion that affects leaves, stems, and fruits. Necrosis can also affect multiple tissue types. For example, blight is a rapid blackening of leaves, stems, and flowers, while rot refers to general necrosis that affects larger areas or plant organs, such as root rot, stem rot, or fruit rot. Dieback is another form of necrosis, beginning at the top of the plant and progressing downward. This symptom is often associated with root rot, cankers, or abiotic factors that inhibit root growth. Color changes are another type of symptom, often affecting leaves, flowers, or fruits. Chlorosis, the yellowing of leaves due to reduced chlorophyll, is a common color-change symptom. A specific form of chlorosis, called a halo, appears around necrotic spots. Some diseases produce distinctive color variations, such as red or purple borders around leaf spots, or bleaching of tissues. Viruses often cause complex color patterns, including mosaic, mottle, and ringspot. Historically, a color-breaking virus that affected tulips led to the 17th-century Tulipmania, where variegated tulips became highly valuable. Today, such patterns are the result of genetic selection rather than viral infections. Growth abnormalities are another symptom type. Galls, which are localized overgrowths of tissue caused by cell enlargement (hypertrophy) and proliferation (hyperplasia), are often induced by pathogens. Distortion, such as twisting or deforming of leaves and fruits, is another common growth abnormality. Wilting, caused by water loss due to diseases impacting the va