UNIT I Fundamentals Of Photonics.pdf
Document Details
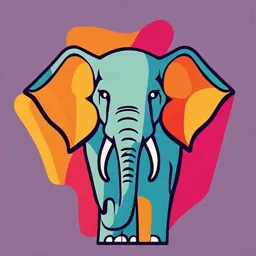
Uploaded by InexpensiveEpiphany6742
Dr. D.Y. Patil University
Tags
Full Transcript
Unit I: Fundamentals of Photonics Topic: Laser Introduction: Transitions in atomic states 1. Absorption When a Photon of energy hν is incident on an atom in energy state E1 the atom gets excited to a higher energy le...
Unit I: Fundamentals of Photonics Topic: Laser Introduction: Transitions in atomic states 1. Absorption When a Photon of energy hν is incident on an atom in energy state E1 the atom gets excited to a higher energy level E2, if the energy of the photon equals the difference in the energy levels i.e., E2– E1 = hν. In this process the photon gets absorbed and the atom gets excited to higher state. The process may be represented as, A + hν = A* Fig. 1.1 2. Spontaneous Emission Consider an atom excited in the absorption process as above. An atom can stay in ground state for infinite time because it is a stable state. But it cannot stay in excited state for long time and comes back to ground state in a very short time called life time of the excited state. The electron in excited state E2 comes to a lower state E1 with the emission of radiation E2 – E1 = hν. This transition is called spontaneous emission. The rate of spontaneous emission depends only on the number of atoms in excited state. The process may be represented as, Fig. 1.2 A* = A + hν 3. Stimulated Emission An atom in the excited state need not wait for spontaneous emission to occur. If a photon with appropriate energy (E2 – E1 = hν) interacts with the excited atom, it can trigger the atom to undergo transition to the lower level, and to emit another photon as shown in the figure 1.3. Fig. 1.3 1|Page This process of emission of photons by an excited atom through a forced transition occurring under the influence of an external agent is called induced or stimulated emission. The existence of this mechanism was predicted by Einstein in 1916. The process may be represented as, A* + hν = A + 2(hν) The rate stimulated emission depends on the number of atoms in excited state and number of photons of required frequency. The photons emitted in this process are coherent. The emitted photons have exactly the same frequency, phase and plane of polarization as those of incident photons. The light produced in this process is directional, coherent, and monochromatic. The outstanding feature of stimulated emission is multiplication of photons. For one photon hitting an excited atom there are two photons emerging. The two photons are in phase and travel along the same direction. These two photons stimulate two excited atoms in their path and produce four photons in this way amplification process takes place in LASER. Fig. 1.4 4. Population inversion: When atomic system is in thermal equilibrium, photon emission and absorption processes take place simultaneously but because N1 > N2 absorption dominates. However, LASER operation requires obtaining stimulated emission. To achieve a high percentage of stimulated emission the majority of atoms should in higher energy level. The non-equilibrium state in which population N2 of higher energy level exceed to a large extent population N1 of the lower energy level is known as state of population inversion. Fig. 1.5 Extending the Maxwell-Boltzmann distribution to this non-equilibrium state of population 2|Page inversion, it is seen that N2 can exceed N1 only if the temperature were negative. In view of this state of population inversion is also referred as a negative temperature state. It does not mean that we can obtain temperature below absolute zero. It should be kept in mind that state of population inversion is obtained at normal temperature. Consider two energy levels in an atomic system as shown figure. Initially N1 > N2 at equilibrium; When the state of population inversion is achieved N2 > N1. Under the population inversion condition, the stimulated emission can produce a cascade of light. The first few randomly oriented spontaneous photons trigger stimulated emission of more photons and those stimulated photons induce still more stimulated emission and so on. As long as the excited state population is more than the lower state population, stimulated emission is more likely than absorption, and consequently light gets amplified. As soon as the population at lower state becomes equal to or more than population at higher state, population inversion ends, stimulated emission diminish and amplification of light ceases. 5. Pumping: In order to realize and maintain the state of population inversion, it is necessary that atoms must be continuously promoted from lower level to upper level. Energy is to be supplied to the laser medium for raising atoms from lower level to the excited metastable state and for maintaining population at higher level at a value greater than that at lower level. The process by which atoms are raised from lower energy level to higher energy level is called pumping. There are various methods of pumping; the two which are commonly used are discussed below a. Optical Pumping: In optical pumping, an external light source (flash lamp) is used to produce high population in some particular energy level E2. After staying there for some time, some of the atoms make spontaneous transition to metastable state E1. Probability of spontaneous emission from metastable state is less due to longer lifetime of stay of atoms in this state; a large population accumulates in this state. This results in population inversion between two LASER levels E1 and E0. Generally this method is used in solid state laser for e.g. Ruby LASER. Fig. 1.6 3|Page b. Electrical Pumping: Electrical pumping can be used in case of LASER materials that can conduct electricity without destroying lasing activity. This method is limited to gases. In case of gas LASER a high voltage pulse initially ionizes the gas so that it conducts electricity. An electric current flowing through the gas excites atoms to the excited level from where they drop to the metastable upper LASER level leading to population inversion. 6. Resonant Cavity (Amplification): A cavity can be constructed using mirrors such that the light rays return to their original position after travelling through the cavity for a certain number of times. Such cavity is known as resonant cavity. Figure shows cavity formed by two parallel mirrors M1 and M2. One of the mirrors is completely silvered (M1) and the other is partially silvered (M2). The LASER beam emerges out of the resonant cavity through the partially silvered mirror M2. Fig. 1.7 The active system is placed in the resonant cavity. The photons travelling along the axis bounce back and forth whereas those photons which do not travel along the axis escape from the cavity after some reflections. As a result, the LASER beam acquires a very high degree of unidirectionality. Stationary waves are formed and the cavity resonates when the distance ‘L’ between the mirrors is integral multiple of λ/2 where λ is the wavelength of the radiation in the active system. Hence the resonant cavity resonates and amplifies in a very narrow range of frequencies due to which the LASER is highly monochromatic Various levels of LASER system (Pumping Schemes): Atoms in general are characterized by a large number of energy levels. Among these energy levels, two, three or four levels will be pertinent to the pumping process. Accordingly pumping schemes are classified as two level, three level, or four level pumping scheme. Among them two level pumping scheme will not lead to population inversion. So in LASER three and four level pumping schemes are important and are widely employed. 1. Two level pumping scheme: It appears that the most simple and straight forward method to establish population inversion is to pump excess of atoms into the higher energy state by applying intense radiations. But a two level pumping scheme is not suitable for obtaining population inversion. 4|Page Fig. 1.8 Population inversion requires that N2 > N1. It is possible only if upper level is populated faster than it decays, so that population of upper level increases and exceeds that of the lower level. Thus it is required that lifetime at upper level should be longer. But, ∆E2·∆t2 ≈ ћ/2 If ∆t2 is longer ∆E2 will be narrow. However, if E2 is narrow we have to use specific frequency photon (ν = (E2-E1)/ћ) to pump atoms. It requires that the pump source should be highly monochromatic. In practice monochromatic source of required frequency may not exist. Even if exist pumping efficiency is very low. The result is that enough population cannot excited to higher level. Further, pumping radiation on one hand excites ground state atoms and on the other hand induces transitions from the upper level to lower level. It means that pumping operation simultaneously populates and depopulates the higher level. Hence population inversion cannot be achieved in two level LASER. All that we may achieve is the system of equally populated levels. 2. Three level pumping scheme: A three level scheme is one in which the lower LASER level is either the ground state or a level whose separation from the ground state is small compared to thermal energy kT. Initially, the population distribution among three levels obeys the Maxwell-Boltzmann distribution. When the atoms are subjected to intense radiation of pumping frequency νp= (E3-E1)/h; the atoms are pumped to the higher level E3. Some of the excited atoms make spontaneous transitions to ground state but many of Fig. 1.9 them undergo, spontaneous non-radiative transition to the metastable level E2. As spontaneous transition from E2 to E1 do not occur; often the atoms accumulates in the metastable level E2. The built up of atoms at E2 continues because of pumping process. Eventually population N2 at E2 exceeds the population N1 at E1 and population inversion is 5|Page obtained. Now a photon of energy hν=E2-E1 can induce stimulated emission and LASER action. Major disadvantage of a three level pumping scheme is that it requires very high pump powers. In this scheme the terminal level of LASER transition is simultaneously the ground state. Therefore, the population inversion requires more than half of the ground state atoms to be lifted to higher energy level. As the ground state is heavily populated, large pumping power is to be used to depopulate the ground state to the required extent. Three level scheme can produce light only in pulses. Once stimulated emission commences, the metastable state E2 gets depopulated very rapidly and population of the ground state increases quickly. As a result, the population inversion ends. One has to wait till the population inversion is again established. Thus, three level LASERs operate in pulsed mode, e.g. Ruby LASER. 3. Four level pumping scheme: A typical four level pumping scheme is shown in fig. In this scheme the terminal LASER level E2 is well above the ground level such that (E2-E1) >> kT. It guarantees that at thermal equilibrium the population of E2 level is negligible. As In three level pumping scheme, pump energy elevates the atoms to short lived upper level E4. The atoms then drop spontaneously to the metastable upper LASER level E3. As the terminal LASER level is virtually vacant, population inversion between states E3 and E2 is quickly established. A spontaneous photon of energy hν=E3-E2 can initiate a chain of stimulated emissions resulting in lasing. The LASER transition takes the atom to E2. From there the atoms lose rest of their energy by radiative or non-radiative transitions Fig. 1.10 and finally reach to ground state E1. Atoms are once again available for excitation. In contrast to three level scheme, the lower laser transition level in four level scheme is not the ground state and is virtually vacant. As soon as some atoms are pumped to the upper level, population inversion is achieved. Thus it requires less pumping energy than a three level LASER does. This is the major advantage of this scheme. Further the lifetime of the lower laser transition level E2 is much shorter as it is not a metastable state. Hence atoms in E2 quickly drop to ground state. This steady depletion of E2 helps sustain population inversion by avoiding accumulation of atoms in the lower lasing level. Therefore, four level lasers can operate in continuous wave (cw) mode. Characteristics of LASER: LASER is a source of light but it has certain properties which makes it different from ordinary source of light. 6|Page 1. Monochromaticity: If light coming from a source has only one frequency of oscillation, the light is said to be monochromatic and the source a monochromatic source. In practice it is not possible to produce a light with only one frequency. Light coming out of any source consists of a light of frequencies closely spaced around a central frequency (ν0). The band frequencies, ∆ν, are called as line width or bandwidth. The light from conventional sources has large bandwidth of the order of 1010 Hz or more. On the other hand, light from LASERS is more monochromatic having bandwidths of the order of 100 Hz. 2. Coherence: Light waves are said to be coherent if they are in phase with each other. Two things are necessary for light waves to be coherent. a. They must start with the same phase at the same position b.Their wavelengths must be the same or they will drift out of phase, because crest of higher frequency wave will arrive ahead of the crest of lower frequency wave. Fig. 1.11 We can distinguish coherence in two classes, temporal coherence or longitudinal coherence and spatial or transverse coherence Temporal coherence: The concept of temporal coherence can be easily understood with the help of following example. Let us consider a single wave propagating along the line SP1P2. Let the phase difference between the points P1 and P2 at time t1 be φ1 and the phase difference between them at time t2 be φ2. If φ2 = φ1, then the wave is said to be temporally coherent. If phase difference φ2 ≠ φ1 and changes from interval to interval and in an irregular fashion then the wave is said to be incoherent. Temporal coherence can be possible for infinite time and infinite length only for a source which can emit light of single wavelength. The existence of finite bandwidth (∆ν) means that the different frequencies present in LASER can get out of phase with each other. If a source of light has a bandwidth ∆ν means that the different frequencies present in LASER can get out of phase with each other. If a 7|Page P2 P1 P3 Fig. 1.12 source of light has a bandwidth of ∆ν hen the time for which the light remains in phase is given as ∆τ = 1. This time is called coherence time. ∆ν For a LASER of bandwidth ∆ν = 1 MHz. ∆τ = 1 μs. On the other hand, sunlight has a bandwidth ∆ν = 1014 Hz, therefore ∆τ = 10-14 s which is much smaller than that of LASER. The length for which the light remains in phase is called as coherence length. ∆L= c×∆τ is called coherence length of beam. For the LASER beam with ∆ν = 1 MHz, the coherence length ∆L=300 m. Only the portions of the same beam separated by a distance less than coherence length are capable of producing stable interference pattern. Spatial coherence: Spatial coherence refers to the continuity and uniformity of a wave in a direction perpendicular to the direction of propagation. If the phase difference for any two fixed points in a plane normal to the direction of propagation of wave does not vary with time, then the wave is said to exhibit spatial coherence. Referring to above fig., SP1 = SP3 and therefore the fields at points P1 and P3 would have the same phase. Thus, an ideal point source exhibits spatial coherence. On the other hand an extended source exhibits lesser spatial coherence. Light emerging from a LASER is both temporally and spatially coherent to a high degree. The reason is that each stimulated transition is a sort of forced oscillation of the excited atomic system and atom radiates a photon in phase with the incoming photon. These two photons eventually interact with two other excited atoms, each causing the emission of a photon on phase with it. The process goes on and as all the photons are produced in phase with the original photon; the LASER beam exhibits a high degree of coherence. Theoretically LASER light could be completely coherent. In practice, coherence is not ideal because all photons do not originate from the same original photon. So, all of them do not start in phase. 3. Directionality: A LASER has a very high degree of directionality and can travel very long distances without deviation. The active medium is kept in optical cavity. The light is reflected back and forth in cavity and light travelling parallel to the axis gets emitted as a LASER beam. The 8|Page light travelling in other directions gets reflected back in the cavity and ultimately gets absorbed at the absorbing material. 4. Brightness: LASER radiates light into a very narrow beam and its energy is concentrated in a small region. This is because divergence is very less. This results into extremely high intensity. Due to this property LASERs have applications in drilling, welding, cutting etc. Active Medium: Active medium refers to a material or substance in a laser or other light-amplifying device that is capable of amplifying light through the process of stimulated emission. When energy is supplied to active medium (Usually in the form of light or electrical energy), it excites the atoms or molecules within it. This energy causes them to emit photons in a coherent manner, which is the basis for the laser’s operation. Active Center: Active Center in a laser refers to the specific atoms, ions, or molecules within the active medium that are directly responsible for the emission of light through the process of stimulated emission. These active centers are part of the medium that actually gets excited and then returns to a lower energy state by emitting photons. CO2 LASER: In a molecular gas laser, laser action is achieved by transitions between vibrational and rotational levels of molecules. Its construction is simple and the output of this laser is continuous. It is a four level laser and it operates at 10.6 μm in the far IR region. It is a very efficient laser. A carbon dioxide molecule has a carbon atom at the center with two oxygen atoms attached, one at both sides. Such a molecule exhibits three independent modes of vibrations. They are: a) Symmetric stretching mode. 9|Page b) Bending mode c) Asymmetric stretching mode The active medium is a gas mixture of CO2, N2 and He. The laser transition takes place between the vibrational states of CO2 molecules. Fig. 1.13 It consists of a quartz tube 5 cm long and 2.5 cm in the diameter. This discharge tube is filled with gaseous mixture of CO2 (active medium), helium and nitrogen with suitable partial pressures. Mixture of CO2, N2, He is in the proportion of 1: 4: 5. The terminals of the discharge tubes are connected to a DC power supply. Two concave mirrors one fully reflecting and the other partially reflecting form an optical resonator. When an electric discharge occurs in the gas, the electrons collide with nitrogen molecules and they are raised to excited states. Now N2 molecules in the excited state collide with CO2 atoms in ground state and excite to higher electronic, vibrational and rotational levels. Since the excited level of nitrogen is very close to the E5 level of CO2 atom, population in E5 level increases. As soon as population inversion is reached, any of the spontaneously emitted photon will trigger laser action in the tube. There are two types of laser transition possible: 10 | P a g e Fig. 1.14 1. Transition E5 to E4 This will produce a laser beam of wavelength 10.6μm 2. Transition E5 to E3 This transition will produce a laser beam of wavelength 9.6μm. Normally 10.6μm transition is more intense than 9.6μm transition. The power output from this laser is 10kW. Helium helps in de-excitation from E3 and E2 level to avoid accumulation at that level. Applications of Lasers: Lasers are used almost in every field: 1. High power Lasers have wide applications in mechanical industry like cutting and welding of metals, drilling holes in metals 2. Because of less divergence it is used in RADAR 3. It has many applications in medical treatments e.g. eye surgery, cosmetic surgery 4. Semiconductor diode LASER is used in communication 5. Lasers have an application in hologram recording and hologram reconstruction 6. Low power semiconductor lasers are used in CD players, laser printers, bar code scanners etc. 7. For distance measurement (range finders) 8. Very high power lasers are used to bring about thermonuclear fusion reaction 9. In war fare to guide the missiles to the targets Application of Laser in Communication: In this technology, optical energy is transferred through the guided media, called optic fiber. When a beam of light enters at one end of a transparent rod the light gets totally internally reflected and gets trapped within the rod. Similar behavior is exhibited by a bundle of fine fibers. A beam enters at one end and gets transmitted to other end even if the fiber is curved. A bundle may have thousands of fibers with diameters in the range of 2 *10 -4 cm to 10-3- cm. The study of properties of such bundles is called fiber optics. One of the most important applications of fiber optics is in telecommunication. Due to high frequency, a light beam in visible region is capable of carrying far more information in comparison with radiowaves and microwaves. 11 | P a g e Holography: This is a technique of producing interference pattern between a direct Laser beam and a Laser beam reflected from an object on a photographic plate. This pattern on a photographic plate when illuminated with the laser in proper manner, produces a three dimensional image of the object called a hologram. It is used in investigations. Holography deals with three dimensional image of the object whereas photography is a two dimensional effect. In photography, the photographic plate records only intensity of light while in holography both the intensity and phase distribution are recorded simultaneously using interference technique. Due to this the image produced by technique of holography has a true three-dimensional form and is as true as the object itself. One more advantage of hologram over photograph is even if a hologram is cut in a number of pieces you can view the whole image with any of the cut pieces. Hologram recording: The recording of hologram is achieved by superposition of the object wave with another wave called reference wave. The reference wave is usually a plane wave. The resulting interference pattern is recorded on a photographic plate. As the shape of object in general is very irregular it results in complicated interference pattern. As shown in fig. a portion of the coherent beam is allowed to be scattered by the object and remaining portion is reflected by a mirror. The former corresponds to the object wave and later to the reference wave. The object beam and reference beam interfere and resultant interference pattern is recorded on a photographic plate. When a photographic plate which has recorded the intensity variation is developed, one obtains the Fig. 1.15 hologram of the object. Hologram reconstruction: To see the reconstructed image, the hologram is illuminated by the reference beam alone, maintaining original alignment and orientation. This process is called as reconstruction. The developed photographic film (hologram) will have alternate transparent and opaque parts of very irregular shape. Thus, it 12 | P a g e will serve as a diffraction grating when illuminated by a light source. The light from hologram will be diffracted inward and outward. One set of diffracted rays will converge to form real image while one set will diverge to form virtual image as shown in fig. Thus, the hologram can form real as well as virtual images. The geometry of construction can be changed, so as to give more emphasis on real or virtual image Fig. 1.16 Advantages of holography: Three-dimensional image High data storage capacity. Large number of images can be recorded on same hologram and each can be seen separately without any overlap Hologram contains complete information of the object in coded form. Hologram cannot be duplicated. Hence holograms are now commonly used for identification of product, on I-cards, credit cards, books etc. Data is secure. Even if part of the hologram is damaged, complete image of the object can be obtained from the remaining part of the hologram 13 | P a g e Optical fiber Introduction: Fiber optics is the technology in which signals are converted from electrical signals into optical signals transmitted through a thin glass fibre and reconverted into electrical signals. In 1870 John Tyndall demonstrated that light can be guided along the curve of a water stream. Because of total internal reflection phenomenon, light gets confined to the water stream and it appears luminous. In 1960 Kapany established that light can be guided by glass fibre. In 1966 Charles Kao and Geogre Hockham proposed transmission of information over glass fibre but there was a huge loss of information due to attenuation. In 1970 Corning glass works developed low loss glass fibres. Invention of solid state lasers in 1970 resulted into development of communication systems based on optical fibres which can be widely used in various fields. An optical fiber is a cylindrical and flexible waveguide made up of transperent material (glass or plastic) which guides light waves along its length by total internal reflection. It is as thin as human hair (70 μm in diameter). Basic structure of optical fibre Core – It is the innermost cylindrical light guiding region. Diameter is 8.5 μm to 62.5 μm. Cladding - Outer optical material surrounding the core that reflects the light back into the core. Diameter is of the order of 125 μm. The refractive index of cladding (n2) is always less than the Fig. 1.17 refractive index of core (n1). Buffer coating - Plastic coating that protects the fiber from physical damage and environmental effects. Hundreds or thousands of these optical fibers are arranged in bundles in optical cables. The bundles are protected by the cable's outer covering, called a jacket. 14 | P a g e Important functions of Cladding: 1. It keeps the size of the fibre constant and reduces the loss of light from the core into the surrounding air. 2. Protects the fibre from physical damage and absorbing surface contaminants. 3. Prevents leakage of light energy from the fibre through evanescent waves. 4. Prevents leakage of light energy from the core through total internal reflection. 5. Reduces the cone of acceptance and increases the rate of data transmission. 6. A solid cladding instead of air also makes it easier to add other protective layers over the fibre. Phenomenon of Total Internal reflection: When a ray of light travels from a denser to rarer medium, it bends away from the normal in the rarer medium. When the angle of incidence θ1 in the denser medium increases, the refracted rays bend more and more away from the normal. At particular angle θc, the refracted ray glides along the surface boundary so that angle of refraction (θ2) becomes 90°. At angles greater than θc, there are no refracted rays at all as they are reflected back in the denser medium. This phenomenon in which light is totally reflected from a denser to rarer medium boundary is known as Total Internal Reflection. Critical angle (θc): when the light travels from denser to rarer medium, the angle of incidence at which light ray travels along the surface instead of refraction is called critical angle. For 𝜃1 = 𝜃𝑐, 𝜃2 = 90° Thus, using Snell’s Law, we get, sin 𝜃 = 𝑛2; 𝑛2 Thus, Critical angle, 15 | P a g e Fig. 1.18 Acceptance angle (θ0 or im):- The acceptance angle is the maximum angle of incidence with respect to the axis of the core for which total internal reflection takes place inside the core. where n is the refractive index of the medium in which optical fibre is kept. (If optical fibre is kept in air then, n0 = 1) Fig. 1.19 Acceptance cone:- The acceptance cone of an optical fiber decides its light gathering power and depends on Acceptance angle. Larger the acceptance angle, larger is the light gathering power Numerical aperture (NA): The numerical aperture of an optical system is defined as the sine of the acceptance angle. It is the measure of amount of light that can be accepted by a fibre. It depends on the R.I. of the core and cladding and is independent of physical dimensions of the optical fibre. Its value ranges from 0.13 to 0.5. Larger NA implies that a fibre will accept large amount of light from the source. 16 | P a g e NA = sin θ0 = Fig. 1.20 Fractional Refractive index change (∆): The fractional difference between the refractive indices of the core and the cladding is known as fractional refractive index change. It is expressed as, ∆= 𝑛1−𝑛2 𝑛1 In order to guide light rays effectively through fibre ∆