Tunneling: Components, Types, and Geological Hazards PDF
Document Details
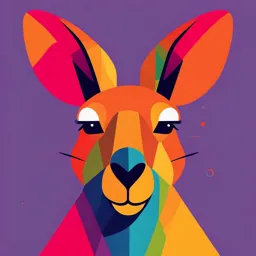
Uploaded by FearlessRhodium1961
Father Saturnino Urios University
Sian Padua
Tags
Summary
This document provides an overview of tunneling, including its components, types, and challenges related to geology, such as overbreak, spalling, flowing ground, squeezing and heaving, and more.
Full Transcript
TUNNELING COMPONENTS AND TYPES OF TUNNELS By: Sian Padua 16.2 COMPONENTS AND TYPES OF TUNNELS A tunnel is a horizontal or slightly inclined passageway excavated through rock or unconsolidated materials, open at both ends. The shape of a tunnel in cross- section varies widely,...
TUNNELING COMPONENTS AND TYPES OF TUNNELS By: Sian Padua 16.2 COMPONENTS AND TYPES OF TUNNELS A tunnel is a horizontal or slightly inclined passageway excavated through rock or unconsolidated materials, open at both ends. The shape of a tunnel in cross- section varies widely, but most tunnels in India are circular, semicircular, or horseshoe-shaped. Tunnel Components: Crown: The roof of the tunnel. Invert: The floor of the tunnel. Walls: The two sides of the tunnel. Spring Line: The meeting point of the roof and sides. Vault Head: The topmost part of the tunnel. Tunnel Support: Structures like steel ribs and masonry walls that support the tunnel and protect it from overburden. EXCAVATION Tunnels are usually excavated from both ends, known as inlet bulkhead or inlet portal and outlet bulkhead or outlet portal, and shafts or caissons may be used for entry or underwater work. DRIFTS AND ADITS: Drift is a small length tunnel used for observation, and testing rock quality. Adit is a drift, that provides passage for entry of men and machineries to conduct tunneling work in additional faces Types of Tunnels: Hydraulic Tunnels: Used for water passage, including power tunnels (for hydropower), irrigation tunnels, diversion tunnels, and spillway tunnels. Traffic Tunnels: Used for transportation, such as highways, subways, and railways. Examples include the Banihal highway tunnel and the Kolkata Metro railway tunnel. Other Purposes: Tunnels are also built for fuel storage, nuclear installations, and hazardous material disposal. Tunneling Through rock By: Shane Sapatalo 16.3 Tunneling through rock Definition: It is a complex engineering feat that requires a deep understanding of geology. It involves excavating underground passages through solid rock formations to create infrastructure such as highways, railways, water supply systems, and more. Challenges: Rock Type and Strength: Hard or soft rock types influence excavation methods and support requirements. Joints and Faults: Fractures in the rock mass can lead to instability and water ingress. Groundwater Pressure: High water pressure can cause flooding and damage to the tunnel lining. Rock Stress: High stress can lead to rockbursts and squeezing, deforming the tunnel. 16.3.1 rock pressure and arching action Rock Pressure is the force exerted by the surrounding rock mass on the tunnel lining. When a tunnel is excavated, the removal of material disturbs the natural stress equilibrium in the surrounding rock. The redistributed stresses exert forces on the tunnel lining, referred to as rock pressure. Arching Action is a natural phenomenon where the rock mass forms an arch-like structure above the tunnel, transferring part of the load to the sidewalls. Strong, self- supporting rocks form a natural arch, reducing the pressure on the tunnel lining. Effective arching minimizes support requirements and enhances tunnel stability. However, in weak or fractured rocks, artificial support systems are necessary to prevent collapse. 16.3.2 Effects of Bedded Rocks on Tunnel Lining Bedded rocks consist of layers with varying strengths and mechanical properties. The interaction between the tunnel and bedded rocks impacts stability: Weak Bedding Planes: These may slide or deform under stress, increasing pressure on the lining. Orientation: If the bedding planes are parallel to the tunnel axis, they may act as weak zones, reducing support capacity. Differential Strengths: Layers of different strengths can lead to uneven load distribution, causing localized stress concentrations on the tunnel lining. Proper alignment of the tunnel with respect to the bedding orientation and tailored support systems can mitigate these effects. 16.3.3 Effect of a Fault Traversing a Tunnel Faults are weak zones in the rock mass caused by fracturing and displacement. When a tunnel intersects a fault: Stability Issues: Fault zones are typically weaker and may contain loose or crushed material, leading to high deformation and collapse risks. Water Ingress: Faults often act as conduits for groundwater, increasing the risk of water inflow into the tunnel. Stress Redistribution: The fault alters stress patterns, potentially inducing differential movement along the fault plane Tunneling through a fault requires special measures such as grouting to stabilize the weak zone and water-proofing systems to manage groundwater inflow. 16.3.4 Effect of folds on tunnel lining Folds in the rock layers introduce varying stress and deformation patterns that can impact tunnel design: Axial Zones: Tunnels passing through fold crests (anticlines) or troughs (synclines) experience stress concentrations, requiring additional support. Inclined Layers: Steeply inclined limbs of folds can result in asymmetric loading on the tunnel lining. Shear Zones: Stress concentrations near fold hinges may cause localized rock failure. Careful geological mapping and orientation of the tunnel with respect to the fold geometry are essential to minimize adverse effects. 16.3.5 Rock Cover and Overbreaks in Relation to Joints The stability of a tunnel is influenced by the amount of rock cover and the presence of joints: Rock Cover: Greater cover provides higher confining stress, enhancing stability. Shallow tunnels with minimal cover are more susceptible to overbreaks and deformation. Joints: The presence of joints or fractures weakens the rock mass and increases the likelihood of overbreaks during excavation. Overbreaks (excess material removal beyond the designed tunnel profile) are common in jointed rock masses and must be minimized through controlled blasting or excavation methods. 16.3.6 Relation of Overbreak with Tunnel Dimensions The size and shape of the tunnel influence overbreak tendencies: Large Tunnels: Larger tunnels expose more surface area, increasing the likelihood of overbreak due to stress redistribution and joint-related instability. Tunnel Shape: Circular or horseshoe-shaped tunnels are less prone to overbreak compared to rectangular profiles, as they distribute stress more evenly. Using proper excavation techniques, pre-support measures, and quality control during blasting can help reduce overbreaks. 16.4 TUNNELLING THROUGH SOFT GROUND Tunneling through soft ground involves excavating unconsolidated materials (muck), requiring immediate support due to the ground's inherent instability. Unlike rock tunneling, the main concerns are managing soil moisture and swelling pressure to prevent collapse. The cut-and-cover method, involving excavation and immediate support, is commonly used 16.4.1 Type of Material, Imposed Load, and Stability Soft Ground Composition: Consists of unconsolidated alluvial deposits or decomposed rock, including clay, silt, sand, gravel, and pebbles, often mixed. Key Challenge: Excavation can damage surroundings and cause subsidence (downward vertical movement of the Earth's surface), requiring careful consideration of the ground's nature. Stand-Up Time: Crucial for stability, representing the time the ground can support itself. Varies from a few hours (stiff clay with supports) to near zero (loose deposits). Ground Behavior: Overburden acts like a fluid, with horizontal pressure one-third to two-thirds of the vertical pressure. Low shear and tensile strength. Pressure on tunnel lining increases over time. Water Table Challenges: Tunneling below the water table significantly increases difficulty due to constant water inflow, frequent overbreaks, and severely reduced stand-up time, making support installation challenging. High-power pumps, compressed air, and drainage systems are used to manage water inflow and lower the water table below the tunnel floor. formula for stability ratio The stability ratio of soil (Nc) at a depth of Z in soft ground tunnelling is the ratio of effective soil pressure (Pz) at the depth Z to undrained shear strength of the soil (Su). Thus, Nc = Pz/Su If Nc is below five, the tunnel is considered safe because it indicates that the soil's shear strength is significantly greater than the pressure acting on it, meaning the soil has the capacity to support itself and can withstand forces imposed during excavation without collapsing. But if it is in more than five, immediate support is necessary, because the pressure acting on the soil approaches or surpasses its shear strength, thus there is a high risk that the tunnel walls may collapse. Use of compressed air is most effective in this sort of support (Sengupta 1991) method of soft ground tunneling Commonly used methods of soft ground tunneling: 1. Cut and Cover method - is best suited for any kind of soft ground with limited overburden cover. Steps: First: Construct vertical retaining walls on either side of the future tunnel alignment to stabilize the ground and prevent collapse during excavation. These walls are often built using the diaphragm wall technique. Second: Excavate the soil between the retaining walls down to the desired tunnel level. Third: Line the excavated space with reinforced concrete (RCC) to create the tunnel structure, forming a robust box-like structure. Fourth: Backfill the area above the newly constructed RCC tunnel box with earth and restore the original ground surface, including any pre-existing roads or structures. method of soft ground tunneling 2. Shield method - is highly effective for tunneling in soft ground at various depths, except in squeezing or swelling clays. It allows for full-face excavation and provides continuous support. Here's how it works: Shield Advance: A jack-powered, metallic shield (shaped like a circular box with a cutting edge) is pushed forward into the soil by hydraulic jacks. This shield shapes the tunnel profile and provides initial support. Support Installation: As the shield advances, iron wire supports are installed at the rear of the shield. The annular spaces between these supports and the excavated ground are immediately filled with thick cement grout (1:1 water-cement ratio) to create a strong, stable lining. Reinforcement and Lining: The grouted section is then heavily reinforced, starting from the bottom and moving upwards to the roof. This creates the permanent tunnel lining. Shaft and Operation: A shaft is typically excavated to the tunnel level, and the shield is introduced and operated from there. The stability ratio (Nc) (Nc = (Pz − Pa)/Su, where Pa is the net air pressure and Su and Pz stand for undrained shear strength and effective pressure of soil) is carefully controlled by managing the net air pressure (Pa). The tunnel lining is designed to withstand both the overburden pressure and the jacking thrust from the shield. The shield method is particularly well-suited for sandy soils with minimal stand-up time because it provides immediate support to the excavated face, preventing collapse. Geological Hazards in Tunnelling By : Nheil Salmasan introduction Geological hazards in tunneling refer to natural conditions or processes in the earth that can pose risks to tunnel construction, safety, and stability. 1. Types of Geological Hazards Overbreak Spalling of Tunnel Rock Flowing Ground Squeezing and Heaving Thermal Springs Gas Flow Seismic Effects 2. overbreak factors affecting overbreak blasting fractured rock sidementary rocks with layered structure is when excavation extends past homogenous rocks the intended boundary of the micaceous metamorphic tunnel rocks folding and faulting 3. spalling is a brittle type of rock failure that occurs in tunnel construction when compressed rock crushes and creates shear cracks 4. flowing ground water, mud, silt, and other loose mitigation measures materials flow out of the tunnel as it Dewatering is being excavated. Ground Freezing or Grouting 5. Squeezing and heaving MITIGATING MEASURE is encountered when immediately covering the tunnelling is done in entire stretch by unconsolidated rock shotcreting and then or claystone providing steel rib containing deleterious clay minerals. supports capable of holding the distributed load. 6. Thermal springs are natural discharges of hot water or steam from the Earth's crust. gas flow refers to the movement of gases, particularly natural gases, from underground sources into the tunnel environment during excavation. 7. Seismic Effects refer to the impact of earthquakes or ground movements on the stability and safety of tunnels. 8. 16.6 DIFFERENT STAGES OF GEOTECHNICAL WORKS FOR TUNNEL By: Arron Bornales 16.6.1 Selection of Tunnel Alignment Keypoints: Initial Evaluation: The first step in choosing a tunnel route is to assess the land's shape (geomorphology) and the types of rocks and soil (geology) in the area. This helps engineers understand what challenges they might face. Alignment Considerations: Prefer a straight tunnel; bends should have an angle ≥120°, avoiding bends near portals. Consider the Landscape: The tunnel's path should be chosen to avoid unstable areas like valleys and hillsides with thin rock cover. Geological and Topographical Factors: Favor alignment through hard, stable rock, free from faults, subsidence, or adverse conditions (karst, slides). Mapping and Surveying: Geological mapping (scale 1:1000 to 1:2000) and cross-sectional profiling help assess terrain. 16.6.2 Subsurface Exploration Subsurface exploration is the process of investigating the geological conditions beneath the surface to gather information about the rock layers, water conditions, and potential hazards before tunnel construction begins. Various techniques included: Exploratory drilling is conducted to gather information about the characteristics of rocks beneath the surface, including the presence of faults and fractures. It helps identify rock types, faults, fractures, and weak areas, and can involve vertical, inclined, or horizontal drilling methods. Testing the Rock: Rock samples from the drill holes are tested in a laboratory to determine their strength, hardness, and other properties. This information is essential for designing the tunnel and choosing the right construction methods. Geophysical surveys: Use techniques such as seismic refraction to identify concealed geological features. These methods are effective in detecting hidden faults and weak zones within the earth. Laboratory Testing: Core samples are tested for density, strength, porosity, and swelling properties. 16.6.3 Construction Stage Work: 3D Tunnel Logging Tunnel logging, also known as three-dimensional (3D) logging, involves plotting various geological features on the walls and ceiling of the tunnel. This includes identifying different rock types, angles of rock layers (dip and strike), and structural elements like faults, joints, and areas where water seeps in. PROCESS OF TUNNEL LOGGING Mapping Geological Features: process that involves the systematic recording and analysis of rock formations, structures, and other geological characteristics encountered during tunnel construction. Creating The Tunnel Plan a–a′: The tunnel floor. a–b and a′–b′: The two walls. b–c and b′–c: The half-arch sections of the roof. o–o′: A central reference line with markings at 3-meter intervals. Dipping Beds (DB) Appear as curved lines in the arch section. Vertical Beds (VB) Appear as straight lines. conventional methods and machineries used in tunneling By: Tyron Xandrix Yatan tunnel boring machine road header machine shield method of tunnel excavation EXCAVATION METHODS OF ROCK TUNNELLING AND SUPPORT SYSTEM excavation methods full-face method top heading and bench method side drift method multiple drift method Types of Tunnel Supports Including Rock Bolting timber planking steel ribs Types of Tunnel Supports Including Rock Bolting steel ribs with pre-cast or shotcreting with or without cast-in-sites concrete slabs wire mesh Types of Tunnel Supports Including Rock Bolting Perfobolt with shotcreting forepoling Types of Tunnel Supports Including Rock Bolting Rock bolting Rock bolting is undertaken when the rock loads are excessive, especially in a large-diameter tunnel (Fig. 16.21). Rock bolting reinforces and supports partially detached, laminated, or otherwise incompetent rocks that would be subjected to failure by gravity and loss of frictional effect. PRESSURE TUNNEL AND LINING By: Jay Vee Burdeos WHAT IS PRESSURE TUNNEL AND LINING? A pressure tunnel is a type of underground tunnel designed to transport water under high hydraulic pressure, typically in hydropower projects or water supply systems. The lining refers to the watertight protective layer made of materials like Plain Cement Concrete (PCC) or Reinforced Cement Concrete (RCC) that ensures the tunnel's structural integrity and efficient operation. Pressure tunnels are designed to withstand high hydraulic and rock pressures, ensuring smooth water flow and structural stability. They use watertight linings of PCC or RCC, bonded to the surrounding rock with cement grouting to seal fractures and distribute pressure. 4. Design considerations include hydraulic needs, geological conditions, and loads (e.g., rock pressure, blasting impacts). Lining thickness ranges from 20–76 cm, with concrete strength varying based on requirements. Construction is staged: corner concreting, side and arch lining, and invert lining. 4. WHAT IS the purpose of PRESSURE TUNNEL AND LINING? Prevent Seepage: Ensures the tunnel remains watertight, avoiding water loss. Handle Hydraulic Pressure: Resists internal hydrostatic forces and external geological pressures. Protect the Structure: Safeguards against erosion, rock deformation, and wear over time. Facilitate Water Flow: Provides a smooth surface to reduce friction and turbulence for efficient water movement. 4. WHere IS PRESSURE TUNNEL AND LINING used? Pressure tunnels and their linings are primarily used in: Hydropower Plants: To transport water to turbines under controlled pressure. Water Supply Systems: For urban or industrial water transport over long distances. Irrigation Projects: To deliver water to agricultural areas efficiently. Mountainous or Geologically Challenging Areas: Where external forces like rock pressure demand enhanced structural support. 4. 16.10 ROCK MASS QUALITY AND SUPPORT REQUIREMENT In designing a rock tunnel, the nature of rock mass with respect to its structural features in addition to its strength is considered. Fault, fold, joints, shear zones, etc. are harmful features of tunnel rocks. In order to bring stability of the tunnels and save from overbreaks, various types of supports are designed as detailed in the following paragraphs... Design Aspects The design aspect of a tunnel including excavation, lining, and support system depends upon the quality of the rock mass at the tunnel. Among the various geological factors that control the quality of rock mass, the following are considered while designing tunnel support: (i) Open joints, clay fi lling, porous and permeable layers, or foliation planes (ii) Unfavourable orientation of rock attitudes (iii) Fault or shear zone or karstic condition (iv) Swelling type of clay (v) Build-up of water pressure (vi) Presence of in-situ stress or deformed rock The support requirements suggested by Proctor (1971) for hard rock and fi rm rock with jointing are as follows: (i) Hard and intact rock—unsupported (ii) Hard stratifi ed and schistose rock—unsupported (iii) Massive, moderately jointed—unsupported in fi rm ground and very fi rm ground and steel rib support at 3 m interval or shotcrete in arch only in troublesome jointed zone Rock Load System of Terzaghi for Tunnel Support In most of the Indian tunnels, rocks are of varied nature and numerous problems are encountered during tunnelling. The rocks of the Himalayan terrain possess high stress and tunnelling is always difficult. The type of support required in a tunnel is dependent on the overall quality (or rock mass quality) that includes all weaknesses of rock. Several classifications of the rock mass quality were devised and modified by a number of authors. Terzaghi (1950b) was probably the first to suggest rock load based simply on geological observation of rock condition. The rock load is expressed as a function of tunnel size. Rock Load System of Terzaghi for Tunnel Support While Terzaghi's rock load method is considered conservative and doesn't account for factors like dip, strike, or alteration, it provides a good, easy-to-estimate approximation. More comprehensive classifications, like the Norwegian Geotechnical Institute (Barton, Lien, and Lunde) and Bieniawski's geomechanics classification, are commonly used in different countries to determine rock mass quality and support requirements, offering more satisfactory rock load predictions for support system design. Methods of Evaluating Tunnel Support by Tunnel Quality Index and Rock Mass Ratio Systems Evaluation of support requirement for a tunnel as suggested in the NGI classification requires first the estimation of tunnel quality index Q (Table 10.5 of Section 10.8) and then finding its relation with equivalent dimension De of the tunnel from the chart. The value of De is obtained from the following relation: De = Excavation span, diameter, or height (m)/Excavation support ratio (ESR) The ESR values of different excavation types proposed by Barton, Lien, and Lunde (1975) fall into five categories (A to E) as shown in Table 16.3 thank you