Flight Deck Automation PDF - Batangas State University
Document Details
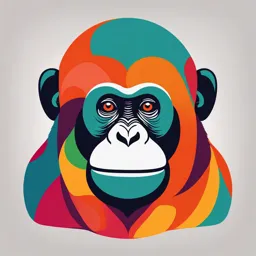
Uploaded by FineKineticArt
Batangas State University
2024
Bagsic, Marc Josef B. Penales, Bernies Maurene M. Rosales, Victor Macgavi B.
Tags
Related
Summary
This Batangas State University document covers flight deck automation, its history, and safety implications. It delves into human factors in aviation and provides examples of automation systems like autopilots and flight management systems (FMS).
Full Transcript
College of Engineering – Department of Electronics Engineering Department of Electronics Engineering Batangas State University - The National Engineering University Alangilan Campus Main II Batangas City Flight Deck Automation In Partial Fulfillm...
College of Engineering – Department of Electronics Engineering Department of Electronics Engineering Batangas State University - The National Engineering University Alangilan Campus Main II Batangas City Flight Deck Automation In Partial Fulfillment of the Requirements of the Course AeE 418 - Human Systems Engineering for the Degree in Bachelor of Science in Aerospace Engineering Prepared by: Group No. 7 Bagsic, Marc Josef B. Penales, Bernies Maurene M. Rosales, Victor Macgavi B. BSAEROENG – 4101 Engr. Alvin Rex L. Arellano Instructor October 11, 2024 College of Engineering – Department of Electronics Engineering Main Topic 6 - Flight Deck Automation: Human Factors and Aviation Safety What is Flight Deck Automation? The utilization of different control systems and technologies that reduce the requirements of human interference; refers to the integration of computer-based systems that assist pilots in controlling and managing aircraft functions. Automation in aviation encompasses a range of systems and tools, from autopilots and navigation aids to advanced flight management systems. These systems handle tasks like flight path calculations, altitude control, autopilot, and auto-throttles, reducing the need for direct manual input. These technologies reduce pilot workload, improve decision-making, enhance operational efficiency, and enhance overall safety. However, as automation becomes more sophisticated, there is a growing concern regarding the potential complacency it can breed among pilots. Historical Overview of Automation in Aviation On December 17, 1903, the Wright Flyer became the first heavier-than-air powered airplane to achieve controlled, sustained flight with an onboard pilot. In these early days of aviation, the pilot controlled all aspects of the aircraft, using only their perceptions as inputs. An impressive feat, given that the machines they operated were generally unstable and difficult to fly. 1900s The concept of flight automation in aviation can be traced back to the 20th century, in the early 1900s with the invention of the gyroscopic autopilot by Lawrence Sperry in 1914, allowing pilots to maintain steady flight without manual control. Over time, automation became increasingly sophisticated, particularly after World War II. 1970s The 1970s saw the introduction of Flight Management Systems (FMS), which revolutionized flight by enabling pilots to input flight plans for the computer to manage, and the Ground Proximity Warning System (GPWS), which is a system designed to alert pilots if their aircraft is in immediate danger of flying into the ground or an obstacle College of Engineering – Department of Electronics Engineering Flight Management Systems (FMS) is a comprehensive system used by pilots to manage the flight plan, navigation, and automation of flight paths. It helps optimize the route, fuel management, and other flight operations. Pilots input flight data into the FMS, which then controls autopilot, auto-throttles, and navigation systems to ensure the aircraft follows the planned flight path. On the other hand, Ground Proximity Warning System (GPWS) is a system designed to alert pilots if their aircraft is in immediate danger of flying into terrain, such as mountains or the ground, especially in low-visibility situations. It works by monitoring the aircraft’s altitude and descent rate and providing warnings if the plane is getting too close to the ground unexpectedly. 1980s With the increasing amount of airspace and system complexity in the 1970s, this spurred the need to improve cockpit functionality – developed in 1980s with the early forms of automation, Integrated Cockpit Displays were introduced, with the purpose of reduced crew workload and improved safety by processing, interpreting, and presenting flight and aircraft system data. These systems consolidate essential flight and aircraft systems information into a unified interface, making it easier for pilots to monitor vital parameters. Key features of integrated displays include digital representations of critical flight parameters such as navigation, altitude, speed, and aircraft system status. This enhanced data presentation aids in quick decision-making, particularly in complex or high-pressure situations. Examples of integrated displays include the Primary Flight Display (PFD), which shows essential flight data, and the Multi-Function Display (MFD), which can present navigation maps, weather information, and other relevant data. Together, these systems significantly improve pilot efficiency and enhance overall flight safety. Traffic Collision Avoidance Systems (TCAS) were also introduced, to warn pilots of potential midair collisions. This system monitors the position and trajectory of nearby aircraft and provides increased situational awareness to pilots. If the system detects the threat of a midair collision, it recommends a maneuver that reduces that risk. Traffic Collision Avoidance Systems (TCAS) works by using transponder signals from nearby aircraft to calculate their positions and movement. This information allows TCAS to determine if there is a potential collision threat and to alert the pilots accordingly. There are two types of alerts provided by TCAS. The first is a Traffic Advisory (TA), which notifies pilots to increase vigilance and monitor the identified nearby aircraft. The second type is a Resolution Advisory (RA), which offers specific instructions to pilots College of Engineering – Department of Electronics Engineering on how to avoid a collision, such as advising a climb or descent to safely alter the aircraft's trajectory 1990s The 1990s brought the advent of Fly-By-Wire (FBW) technology, which replaced mechanical controls with electronic systems. This technology uses electronic sensors and computers to interpret pilot inputs and manipulate control surfaces, allowing for real-time monitoring of the aircraft’s performance and the implementation of advanced control algorithms. In critical situations, FBW systems can automatically intervene to prevent the aircraft from exceeding its safe operating limits, mitigating the risk of stalls, spins, and other hazardous conditions, enhancing overall safety of flight. This era also marked the widespread use of highly automated cockpits, as seen in aircraft like the Airbus A320, which is known for their highly automated cockpits, further refining pilot interaction with sophisticated flight control systems. In modern times, advancements in computing power and sensors have led to the development of highly automated cockpits, where pilots oversee systems that manage nearly all flight phases, from takeoff to landing. Automation continues to enable a level of operational safety that we often take for granted, with aviation remaining one of the safest forms of commercial transportation available. Looking back on the now 120 years of powered flight, it’s remarkable how aviation engineers have matured the airplane into one of the most complex–and safe–machines on earth. In particular, flight automation advancements have significantly transformed the relationship between pilots and aircraft. Long before the highly-automated systems are developed today, aviation engineers developed simple machines that automated manual flight tasks. The advantages of these automation systems are evident and several–including improved accuracy and speed– however increased safety has shown to be the most important benefit. Automation Advantages Reduces the Risk of Human Errors Automation reduces human errors, which are a leading cause of aviation accidents. By taking over routine tasks, automation minimizes the chance of mistakes, especially during critical phases of flight. College of Engineering – Department of Electronics Engineering Reduces Pilot Workload Automation handles routine and repetitive tasks, allowing pilots to focus on decision-making, communication, and system monitoring. This helps reduce fatigue, improving overall cognitive performance and alertness. Precision and Accuracy Automation systems execute tasks like navigation, altitude control, and landing procedures with high precision and accuracy, leading to smoother and more consistent operations. Assistance During Emergencies Advanced automation features, such as autothrottle and Autoland, assist during emergencies like engine failures or low-visibility landings, increasing the chances of a safe outcome. Increases Passenger Comfort Automation helps maintain smoother flight operations, ensuring stable altitude, reduced turbulence impact, and optimized routes, which enhances the passenger experience. Improved Flight Path Control and Reduced Weather Minima Automation enhances flight path precision and can reduce the impact of adverse weather conditions by automating landing and approach in low visibility. Enhanced Systems Monitoring and Diagnostics Systems such as ECAM (Electronic Centralized Aircraft Monitor) and EICAS (Engine Indicating and Crew Alerting System) improve pilot awareness of aircraft system status, aiding both pilots and maintenance staff. Importance of Studying Automation Enhancing Safety Automation significantly reduces the risk of human error, particularly during critical phases of flight such as takeoff, landing, and high-altitude cruising. Automated systems maintain consistent control over aircraft functions, providing pilots with accurate data and College of Engineering – Department of Electronics Engineering real-time decision support. This allows pilots to focus on higher-level decision-making tasks while automated systems manage routine or repetitive operations. The reliability of automation in detecting and correcting anomalies contributes to overall flight safety, preventing incidents that may arise from human limitations such as fatigue or situational misjudgment. Improving Efficiency Automation optimizes flight operations by streamlining tasks like navigation, fuel management, and flight path optimization. These systems improve fuel efficiency and reduce operational costs by minimizing unnecessary deviations and ensuring the most efficient routes are flown. This not only reduces costs but also helps minimize the environmental impact of aviation through lower emissions. Furthermore, by reducing the workload of pilots, automation allows them to perform their roles more effectively, without becoming overwhelmed by mundane tasks. Mitigating Human Limitations Automated systems are designed to handle repetitive or high-stress tasks that human operators may struggle with during long or complex flights. By reducing the cognitive load on pilots, automation helps maintain optimal decision-making capabilities, especially in high-pressure situations. However, while automation can improve overall performance, it is important to study its limitations and potential risks, such as over-reliance or technical failures, which could lead to complacency or diminished pilot proficiency. Supporting Decision-Making Automation provides critical real-time data and assists pilots in complex decision-making, especially during abnormal or emergency situations. Automated alerts and recommendations help ensure that pilots have all the necessary information to make informed decisions, reducing the chances of human error and improving overall situational awareness. Levels of Automation Represents a spectrum of human involvement in system operation. At the manual level, the operator has complete control, with no automation. As the level of automation increases, the system takes on more tasks, with the operator providing oversight and intervention as needed. College of Engineering – Department of Electronics Engineering These considerations include the design of the human-machine interface, workload management, situational awareness, trust between the operator and the system, and the smooth transition between manual and automated control. 1. Sheridan and Verplank’s 10 Levels of Automation Level 1: Human performs the entire task except for the actual operation by the machine: The operator is fully in control, with the machine only executing basic operations. Level 2: Human requests suggestions from the computer and selects from options: The system provides options, but the final decision rests with the human. Level 3: Computer suggests options to the human: The system generates alternatives for consideration without requiring a request. Level 4: Computer suggests options and proposes one of them: The system recommends a specific action among its suggestions. Level 5: Computer chooses an action and performs it if the human approves: The system acts based on its choice but requires human consent to proceed. Level 6: Computer chooses an action and performs it unless disapproved by the human: The machine can act independently unless explicitly stopped by the operator. Level 7: Computer chooses an action, performs it, and informs the human: The system executes its decision autonomously and communicates its actions to the operator. Level 8: Computer does everything autonomously, but may inform the human if requested: The system operates independently without needing to notify the operator unless asked. Level 9: Computer informs the human if it considers it necessary: The machine autonomously decides when to communicate with the operator based on its judgment. Level 10: Full autonomy: computer decides everything and acts independently: The system functions entirely on its own without any human intervention or oversight. 2. Roles of Automation Human Factors Considerations Situational Awareness College of Engineering – Department of Electronics Engineering Increased reliance on automation can lead to diminished situational awareness. Pilots may become complacent and less engaged. Skill Degradation Prolonged use of automation can result in a decline in manual flying skills. Essential for pilots to maintain proficiency for unexpected situations. Training Implications Training programs must adapt to ensure: Pilots maintain manual flying skills. Human-Automation Interaction 1. Human Roles in the Automated Cockpit In a modern flight deck, pilots play a dual role: they are both operators and supervisors of automated systems. Automation is responsible for executing tasks such as navigation, throttle management, and autopilot functions, allowing the pilot to focus on higher-level decision-making and flight management. However, the human operator remains responsible for the safe operation of the aircraft, especially during critical or non-routine situations where manual intervention may be necessary. 2. Cognitive Workload and Automation Automation significantly alters the pilot’s cognitive workload by offloading routine tasks, such as maintaining flight trajectory or managing engine performance, to the automated system. However, this reduction in manual control can lead to two potential outcomes: A. Reduced Workload During Routine Operations: Automation can relieve pilots of repetitive tasks, allowing them to focus on monitoring the system and making strategic decisions. This reduces fatigue and enhances the crew’s ability to manage complex flight environments, such as air traffic control instructions and weather conditions. B. Increased Cognitive Demands in Non-Routine Situations: College of Engineering – Department of Electronics Engineering Automation requires constant monitoring, and when failures or unexpected events occur, the cognitive workload can spike. Pilots must quickly diagnose the issue, decide on the appropriate corrective action, and sometimes override automation to regain manual control. This shift from a passive monitoring role to active problem-solving in critical moments can lead to cognitive overload if the system's failure occurs abruptly. 3. Trust in Automation: Finding the Balance Trust is a key factor in human-automation interaction, as it influences how much pilots rely on automated systems. The relationship between the pilot and automation is delicate, and either over-trusting or under-trusting the system can lead to serious risks. A. Over-Trust: Over-trusting automation can lead to complacency, where pilots become too dependent on the system and fail to remain vigilant. This complacency may result in delayed responses during unexpected events, as pilots may be slow to recognize the need to intervene. B. Under-Trust: Under-trusting the system can lead pilots to unnecessarily disengage automation or override it, missing out on the safety and efficiency benefits that automation provides. This can lead to higher workloads, especially during routine tasks that automation could handle more efficiently. The key to effective human-automation interaction is ensuring that pilots develop an appropriate level of trust through experience, training, and a clear understanding of the system's limitations and capabilities. 4. Pilot Decision-Making and Automation Automation provides support in pilot decision-making by processing large amounts of information and offering data-driven recommendations or alerts. However, the interaction between human decision-making and automated systems is complex: A. Supporting Decision-Making: Automation can enhance decision-making by providing timely alerts, suggesting optimal flight paths, and offering performance-related data. For instance, autopilot systems can recommend altitude changes based on wind conditions, optimizing fuel efficiency. B. Impairing Decision-Making: College of Engineering – Department of Electronics Engineering Over-reliance on automation can reduce a pilot’s situational awareness, leading to degraded decision-making skills. This is especially critical in high-stress or emergency scenarios, where quick judgment and manual control are necessary. A pilot’s ability to make independent decisions may diminish if they become overly reliant on automated recommendations. Effective decision-making in a flight deck requires that pilots maintain an active role in monitoring and verifying the automated system’s outputs and remain prepared to take manual control when needed. 5. Communication and Feedback in Automation Effective communication between automated systems and pilots is essential for maintaining situational awareness and ensuring that the pilot remains informed about the current state of the flight. A. System Feedback: Automated systems must provide clear and understandable feedback regarding the status of various aircraft functions. For example, alerts and system messages should be intuitive and unambiguous so that pilots can quickly interpret the information and take appropriate action. B. Mode Awareness: Mode confusion is a common issue in human-automation interaction, where pilots are unaware of the current state or mode of the automated system. This confusion can lead to inappropriate actions or inaction at critical moments. For example, a pilot may assume that the autopilot is engaged when, in reality, it has disengaged due to a system fault, leading to a potential safety hazard. Mode awareness is critical because: Safety: Knowing the current mode helps pilots anticipate the system's behavior and respond appropriately, especially in dynamic situations. Decision-Making: It aids pilots in making informed decisions regarding manual versus automated control. Designing systems that offer clear, intuitive communication—such as visual displays, auditory alerts, and tactile feedback—helps reduce confusion and maintain effective interaction between pilots and automation. College of Engineering – Department of Electronics Engineering Automation Risk Automation Reliability Failure modes and limitations of automation systems;Automation reliability refers to the dependability of automated systems in performing their intended functions under expected conditions. In aviation, while flight deck automation enhances safety and efficiency, it is not without its limitations. These systems are subject to technical malfunctions, software glitches, sensor failures, and environmental factors that may degrade their performance. For instance, reliance on faulty sensor data could lead an automated system to make incorrect flight decisions, endangering the aircraft. Human Error and Automation How over-reliance on automation can lead to human error; Failure modes in automated systems can stem from both hardware and software issues. Common hardware failures include sensor degradation, communication link disruptions, or component wear. Software issues, such as bugs or faulty algorithms, can lead to incorrect decisions by the system. Situational Awareness Degraded situational awareness in highly automated environments; Situational awareness (SA) is the pilot’s ability to perceive, comprehend, and project the current and future state of the aircraft in relation to its environment. In automated cockpits, SA can be degraded when pilots become passive monitors instead of active participants in the flight process. This 'automation-induced complacency' can cause pilots to lose awareness of critical flight parameters, such as speed or altitude, especially during long flights or during routine operations when the system appears to be functioning correctly. Automation Bias The risk of automation bias: when pilots become overly dependent on automation; Automation bias occurs when pilots place undue trust in automated systems, even when there are signs the system may not be functioning properly. Pilots may ignore or discount contradictory manual input or alerts, assuming that the automated system is more accurate. This misplaced confidence can lead to poor decision-making during critical moments. For example, pilots may fail to cross-check information presented by the automation system with other data sources, leading to incorrect actions. College of Engineering – Department of Electronics Engineering Automation Disadvantages Risk of Complacency Over-reliance on automation can lead to pilot disengagement, causing slower reaction times in critical situations when manual intervention is required. Loss of Manual Flying Skills Extended use of automation can erode pilots' manual flying skills. Much like a driver accustomed to autopilot systems, pilots may struggle to operate manually during automation failures. Complex Cockpit Environment The integration of multiple automation systems can create a cluttered cockpit environment. Pilots may become overwhelmed when diagnosing and responding to system failures, potentially distracting them from their primary task of flying the aircraft. Automation Dependency Over-dependence on automation can lead to difficulties handling situations when these systems fail, reducing a pilot’s ability to perform necessary manual interventions. Challenges in Monitoring Role Automation shifts the pilot’s role from active operation to monitoring, a task humans are less suited to perform effectively for long periods. For instance, when autothrottle disengages unexpectedly, pilots accustomed to its use may not notice speed deviations, as seen in incidents like the 2009 B737-800 crash in Amsterdam. Increased Workload Due to Automation Management While automation can reduce workload by managing tasks, it can also add tasks related to managing and inputting data, leading to increased mental workload. Poorly designed automation systems may further reduce situational awareness, increasing cognitive load during system malfunctions. College of Engineering – Department of Electronics Engineering Overwhelming System Failures In complex failure events, such as the Airbus A380 engine breakup after takeoff from Singapore in 2010, failure information can overwhelm pilots, hindering diagnosis and distracting from the primary task of flying the aircraft. Strategies for Mitigating Automation Risks Design solutions to enhance situational awareness and reduce risk; To mitigate the risks associated with automation, cockpit designs must prioritize situational awareness. Enhanced feedback mechanisms, such as visual and auditory cues, can help keep pilots informed of the aircraft's real-time status, even when automated systems are in control. Adaptive automation is another solution, where the system adjusts its level of autonomy based on the pilot's cognitive workload, ensuring that they remain engaged. Ensuring that automated systems are transparent and provide clear, interpretable feedback is essential to maintaining pilot awareness and control. Training programs for pilots on managing automation risk; Training programs are vital to managing automation risks. Pilots must regularly train in manual flying skills to ensure they are prepared to take control when automation fails. Simulation-based training allows pilots to experience automated system failures in a controlled environment, helping them practice quick decision-making and recovery techniques. Additionally, training should emphasize monitoring and understanding automated systems to maintain situational awareness and minimize the risks of automation bias Examples of Automation Systems: 1. Autopilot Systems Function: Automatically controls the aircraft's flight path, maintaining altitude, heading, and speed. Example: Aileron and elevator control adjustments during cruise flight. 2. Flight Management Systems Function: Integrates navigation, performance, and flight planning functions. It optimizes the flight route and monitors fuel usage. College of Engineering – Department of Electronics Engineering Example: Programming a flight route and automatically adjusting for optimal fuel consumption. 3. Automatic Landing Systems Function: Helps in landing the aircraft using precision guidance. Example: Instrument Landing System (ILS) that provides lateral and vertical guidance during approach and landing. 4. Thrust Management Systems Function: Automatically adjusts engine thrust to maintain desired speed or altitude. Example: Autothrottle systems that adjust engine power during climb and descent phases. 5. Traffic Collision Avoidance Systems (TCAS) Function: Monitors surrounding air traffic and provides alerts or recommendations to avoid mid-air collisions. Example: TCAS issuing a "climb" or "descend" instruction to avoid a potential collision. Accidents related to the Automation System: Case 1: Notable Aviation Incidents Involving Automation 1. Asiana Airlines Flight 214 (2013) Incident: While landing at San Francisco International Airport, the Boeing 777-200ER crashed short of the runway. Cause: The National Transportation Safety Board (NTSB) attributed the crash to the flight crew's mismanagement of the airplane's descent, which involved unintended deactivation of automatic airspeed control. The pilots relied too heavily on the automation and failed to monitor their altitude correctly. Outcome: Three fatalities and 187 injuries occurred as a result of the crash, highlighting issues related to pilot training and reliance on automated systems College of Engineering – Department of Electronics Engineering Case 2: Successful Automation Interventions 2. Qantas Flight 72 (2008) Incident: An Airbus A330 experienced an in-flight upset due to a malfunction in its Air Data Inertial Reference Unit (ADIRU), which caused the aircraft to pitch down unexpectedly. Automation's Role: The aircraft's fly-by-wire system automatically engaged to stabilize the aircraft after the initial pitch down, allowing the pilots to regain control. The automation helped prevent a potential crash, despite the initial loss of altitude. Outcome: While there were injuries among passengers and crew, the automation prevented a catastrophic outcome, showcasing its effectiveness in managing unexpected situations. College of Engineering – Department of Electronics Engineering References Huang, T. (2024, August 12). Council Post: What The History Of Automation In Flying Can Teach IT Operations. Forbes. https://www.forbes.com/councils/forbestechcouncil/2021/11/24/what-the-history-of-a utomation-in-flying-can-teach-it-operations/ Human Factors in Aviation and Aerospace. (2022). Google Books. https://books.google.com.ph/books/about/Human_Factors_in_Aviation_and_Aerospa ce.html?id=hO5eBwAAQBAJ&redir_esc=y Knudsen, A. (2023, October 6). Piloting the Future: Pros & Cons of Automation in Aviation. NaviMinds. https://naviminds.com/automation-in-aviation/ Kwak, Y.-P., Choi, Y.-C., & Choi, J. (2018). Analysis between Aircraft Cockpit Automation and Human Error Related Accident Cases. International Journal of Control and Automation, 11(3), 179–192. https://doi.org/10.14257/ijca.2018.11.3.16 Little, B. (2019, March 20). Automation of Planes Began 9 Years After the Wright Bros Took Flight—But It Still Leads to Baffling Disasters. HISTORY. https://www.history.com/news/plane-automation-autopilot-flight-302-610 Mosterd, M. (2024, February 15). The Evolution of Flight Automation - Merlin Labs. Merlin Labs. https://merlinlabs.com/the-evolution-of-flight-automation/ Qantas 72. (2024). CODE 7700; The Secret to Flight Safety. https://code7700.com/case_study_qantas_72.htm College of Engineering – Department of Electronics Engineering Skybrary. (2021a, May 26). Cockpit Automation - Advantages and Safety Challenges. SKYbrary Aviation Safety. https://skybrary.aero/articles/cockpit-automation-advantages-and-safety-challenges Skybrary. (2021b, May 29). Maneuvering Characteristics Augmentation System (MCAS). SKYbrary Aviation Safety. https://skybrary.aero/articles/maneuvering-characteristics-augmentation-system-mcas Republic of the Philippines BATANGAS STATE UNIVERSITY The National Engineering University Alangilan Campus Golden Country Homes, Alangilan Batangas City, Batangas, Philippines 4200 Tel Nos.: (+63 43) 425-0139 local 2847 E-mail Address: [email protected] | Website Address: http://www.batstate-u.edu.ph NARRATIVE REPORT FLIGHT DECK AUTOMATION Flight deck is usually referred to as the cockpit. It contains flight instruments on an instrument panel, and the controls that enable the pilot to fly the aircraft. Automation refers to the use of technology, programs, robotics, or processes to achieve outcomes with minimal human input. Flight deck automation has revolutionized the aviation industry, enhancing safety, efficiency, and reducing pilot workload. It involves the use of computerized systems to perform various tasks, from navigation to autothrottle and autopilot. However, the effective integration of automation into flight operations requires a careful understanding of its levels, the interaction between pilots and automated systems, and the potential risks associated with its use. Why do we need flight deck Automation? Flight deck automation plays a vital role in enhancing safety, efficiency, and comfort for the aviation industry. By automating routine tasks and providing pilots with advanced tools, automation helps to ensure safer and more reliable air travel. 6 Levels of Automation Level 0: No Automation Level 1: Pilot Assistance Level 2: Partial Automation Level 3: Conditional Automation Level 4: High Automation Level 5: Full Automation Level 0: No Automation The pilot is in complete control of every movement. Cockpit are controlled 100% manually at all times. Republic of the Philippines BATANGAS STATE UNIVERSITY The National Engineering University Alangilan Campus Golden Country Homes, Alangilan Batangas City, Batangas, Philippines 4200 Tel Nos.: (+63 43) 425-0139 local 2847 E-mail Address: [email protected] | Website Address: http://www.batstate-u.edu.ph Example: The pilot activates the autothrottle to maintain a target speed. The pilot can manually adjust the speed if needed. It means the pilot does all the work with no help from automation/instruments. Level 1: Pilot Assistance The pilot is fully in control, with automation providing only advisory information. Example: The pilot activates the autothrottle to maintain a target speed. The pilot can manually adjust the speed if needed. Autopilot mode is engaged, but the pilot remains fully in control. The autopilot provides advisory information such as suggested heading and altitude. Level 2: Partial Automation The pilot is still in command, but automation can execute tasks under pilot supervision. Example: The pilot activates the autothrottle to maintain a target speed. The pilot can manually adjust the speed if needed. Level 3: Conditional Automation Automation can execute tasks independently, but the pilot can intervene at any time. Example: The autopilot is engaged and controlling the aircraft's heading, altitude, and speed. The pilot can intervene at any time to override the automation. Level 4: High Automation The pilot is essentially a supervisor, overseeing the automated system's operations. Example: The autopilot is controlling the aircraft's entire flight path. The pilot monitors the system's performance and can intervene if necessary. Level 5: Full Automation The aircraft is fully autonomous, with no pilot intervention required. This level of automation is still under development and not yet in widespread use in commercial aviation. Human-Automation Interaction Human-automation interaction (HAI) involves people specifying task goals and constraints, controlling the automation to start or stop execution, and receiving information, Republic of the Philippines BATANGAS STATE UNIVERSITY The National Engineering University Alangilan Campus Golden Country Homes, Alangilan Batangas City, Batangas, Philippines 4200 Tel Nos.: (+63 43) 425-0139 local 2847 E-mail Address: [email protected] | Website Address: http://www.batstate-u.edu.ph energy, physical objects, or substances from it. This interaction involves trade-offs between goals and constraints, allowing for effective task management and control. This emphasizes the importance of understanding how pilots interact with automated systems, particularly regarding their decision-making processes about when to engage or disengage automation. This understanding is crucial for designing systems that enhance safety and efficiency in aviation operations. Factors Affecting Human- Automation Interaction Automation Features: Automation features influenced workload judgments, task management, and the possibility of automation-related errors; however, task features appear to attenuate automation's impact on situation awareness. Unanticipated tasks had a greater impact on pilot judgments than operational pressures. The findings indicate that, while modifications to automated systems may be minor in technical terms, the cognitive and behavioral impact on operators may be significant. The performance implications of automation innovations in aviation and other areas must be evaluated in terms of work characteristics and situational needs. Task and Context Features: The context in which automation is used, such as operational stressors or unexpected tasks, can alter pilots' perceptions and strategies for using automation. For instance, unanticipated tasks tend to have a more pronounced effect on pilots' judgments than operational stressors. The interaction of automation features with task and context variables influenced pilots' predictions of behavioral and psychological consequences identified as critical HAI outcomes: workload demands, effort involved in task management, required automation cross-checking, level of automation awareness, level of situation awareness, and likelihood of an automation-related error. Design Challenges: This includes design issues with current automated systems, particularly the flight management system (FMS), which can be cumbersome and lead to errors due to its complex interface. Although newer systems have attempted to fix past issues, current design characteristics remain deficient, most notably in terms of system observability—the extent to which the automation allows the operator to understand its logic and see its current and future activities and targets—and system directability—the ease with which the system's design allows the user to intervene when necessary. Observability in automated systems is affected by system displays with multiple layers or tightly coupled subsystems, leading to poor automation, increased workload, and mode errors. Physical control features, such as spatial configuration of input devices, can also impair directability, potentially causing delays or errors in critical input. Individual Differences: Variability in individual pilot characteristics, such as experience level and fatigue, also influences how they interact with automation. Experienced pilots may have developed specific strategies that affect their reliance on automated systems Republic of the Philippines BATANGAS STATE UNIVERSITY The National Engineering University Alangilan Campus Golden Country Homes, Alangilan Batangas City, Batangas, Philippines 4200 Tel Nos.: (+63 43) 425-0139 local 2847 E-mail Address: [email protected] | Website Address: http://www.batstate-u.edu.ph differently than less experienced pilots. Some pilot training programs, like multi crew pilot license training, focus on preparing co-pilot candidates for advanced airliner seats using a competency-based approach, aiming to improve flight deck safety. Those who achieve this license may exhibit different HAI patterns and preferences. Fatigue was also found to slow pilots’ system monitoring and impair their understanding of system indications and may lead to overreliance. Future Changes in Automation: As aviation technology continues to evolve, it is essential to consider how changes in automation will affect pilot performance and decision-making processes. This suggests that even minor technical adjustments can have significant cognitive impacts on operators. Future flight operations will require pilots to interact with more and increasingly complex automation. It is conceivable that there also will be increased pressure on pilots to rely on automation to enable high levels of efficiency in operations. Crew training therefore must provide pilots with the opportunity to explore automation use in diverse situations, highlighting different task and operational constraints. Automation Risk Feedback on System States Automation-related incidents often occur when the state of the automated system changes without clear communication to human operators. For example, in the 1972 Lockheed L-1011 crash in the Florida Everglades, the flight crew did not recognize that the altitude hold function of the autopilot had been disconnected. Poor feedback on the state of automation contributed to the accident. To validate the disengagement of automation, it should be clearly signaled to the human operator. Most current autopilots provide an aural or visual alert upon disconnect, which remains active for a few seconds or requires additional pilot input before silenced. Persistent warnings, especially when requiring additional input, aim to decrease the chance of an autopilot disconnect or failure going unnoticed. Lack of Understanding of Automation Automated systems are known for their complexity, with numerous interacting subcomponents that make understanding their effects difficult or impossible. Misunderstandings of complex systems, such as flight management systems (FMS), can arise due to a mismatch between the pilot's mental model and the automated system's behaviors. This has led to incidents and accidents, some of which resulted in serious loss of life. For example, in 1994, an A300 crashed in Nagoya, Japan, after pilots accidentally engaged the autopilot's go-around mode. The pilots attempted to continue the approach by Republic of the Philippines BATANGAS STATE UNIVERSITY The National Engineering University Alangilan Campus Golden Country Homes, Alangilan Batangas City, Batangas, Philippines 4200 Tel Nos.: (+63 43) 425-0139 local 2847 E-mail Address: [email protected] | Website Address: http://www.batstate-u.edu.ph manually deflecting the control column, which normally disconnects the autopilot. However, in this particular aircraft and mode, the autopilot had to be manually deselected and could not be overridden by control column inputs. This led to a power struggle between the pilot and the autopilot, with the pilot pushing the nose down through elevator control and the autopilot lifting the nose up through trim control, causing the aircraft to become so far out of trim that it could no longer be controlled. Overreliance and Dependency Automated systems are generally reliable, except for some with high false alarm rates. Operators often rely on automation due to its opacity and complexity, but this can lead to ‘complacency effect’ when the automation fails to indicate a hazard. Conversely, if automation reliability is low, operators usually have high compliance, attending to tasks other than automated tasks until the alert is triggered, improving multitask performance. Pilots often resist reducing their full automation capability in routine or abnormal situations. If available, they may partially retain automated systems instead of reverting to manual control. This leads to a loss of situational awareness and reduced PM monitoring of PF actions. For example, the Royal Majesty cruise liner ran aground near Nantucket due to an overreliance on automated systems. The catastrophe caused $2 million in structural damage and $5 million in lost revenue. According to the NTSB investigation, the crew's overreliance on the Automatic Radar Plotting Aid, as well as management' failure to provide proper training on automation features and capabilities, contributed to the accident. Strategies to Reduce Automation Risk Pilot Enhancements Training Understanding Automation: Pilots must develop a comprehensive understanding of both full and partial automation functionalities. This includes recognizing the importance of monitoring automated systems to quickly address incorrect inputs or malfunctions. Airmanship Skills: Emphasizing basic airmanship and manual flying skills is crucial. Pilots should be trained to competently operate aircraft with or without automation engaged, ensuring they can manage situations requiring manual control effectively. Task-Specific Automation Use: Training should guide pilots in selecting the appropriate level of automation for specific tasks. For instance, while high levels of automation may reduce workload during cruise, they can lead to cognitive overload in complex terminal environments. Operational Procedures Republic of the Philippines BATANGAS STATE UNIVERSITY The National Engineering University Alangilan Campus Golden Country Homes, Alangilan Batangas City, Batangas, Philippines 4200 Tel Nos.: (+63 43) 425-0139 local 2847 E-mail Address: [email protected] | Website Address: http://www.batstate-u.edu.ph Flexible Standard Operating Procedures (SOPs): SOPs should allow flexibility for pilots to choose between full automation and manual flying, particularly during intervals between recurrent simulator training. This flexibility helps maintain pilot competence. Monitoring Automation Use: Implementing Operational Flight Data Monitoring (OFDM) programs can track the extent of automation usage across flights, providing data to refine training and operational practices. Regulatory Improvements Automation Management Policies: The European Aviation Safety Agency (EASA) recommends developing specific automation policies tailored to different aircraft types. This ensures that training aligns with the unique automation characteristics of each aircraft. Competence-Based Training (CBT): Enhancing CBT and Evidence-Based Training (EBT) approaches will better address how pilots manage automation, improving overall safety and efficiency. Crew Resource Management (CRM): Improving Multi-Crew Cooperation (MCC) training will facilitate better management of automated systems among flight crews, thereby enhancing safety. Summary The presentation discusses that while flight deck automation has improved safety and operational efficiency, it also requires careful management of human-automation interaction and ongoing pilot training to mitigate risks. The presentation aims to raise awareness of these issues and encourage the development of more resilient and cooperative flight systems for the future. The section on Levels of Automation provides a structured framework ranging from Level 0 (no automation) to Level 5 (full automation), illustrating how these levels apply to flight deck operations. Real-world examples clarify how different levels influence the responsibilities shared between pilots and automated systems. The human-automation interaction (HAI) segment addresses the dynamic relationship between pilots and automated systems. Automation risk is explored with a focus on real-world incidents, discussing potential hazards like mode confusion, system failures, and cognitive underload or overload. The report emphasizes how the aviation industry has learned from past accidents, fostering a more cautious and calculated approach to automation use. Republic of the Philippines BATANGAS STATE UNIVERSITY The National Engineering University Alangilan Campus Golden Country Homes, Alangilan Batangas City, Batangas, Philippines 4200 Tel Nos.: (+63 43) 425-0139 local 2847 E-mail Address: [email protected] | Website Address: http://www.batstate-u.edu.ph Mitigation strategies such as enhanced pilot training and regulatory improvements are also covered to address these risks. References: 1. https://canadianaam.com/2022/06/07/aam-aircraft-levels-of-autonomous-flying/ 2. https://ph.images.search.yahoo.com/search/images?p=cockpit+of+boeing+737&fr=m cafee&type=E210PH885G0&imgurl=https%3A%2F%2Fph.images.search.yahoo.co m%2Fsearch%2Fimages%3Fp%3Dcockpit%2Bof%2Bboeing%2B737#id=1&iurl=htt p%3A%2F%2Fwww.aviationtoday.com%2Fwp-content%2Fuploads%2F2016%2F04 %2FMAX12028229.jpg&action=click 3. https://ph.images.search.yahoo.com/search/images;_ylt=AwrKA3HqGgVnwjcFORKz Rwx.;_ylu=Y29sbwNzZzMEcG9zAzEEdnRpZAMEc2VjA3Nj?p=cockpit+of+ATR& fr=mcafee#id=4&iurl=https%3A%2F%2Fi.ytimg.com%2Fvi%2FjapuiVakXIM%2Fm axresdefault.jpg&action=click 4. https://ph.images.search.yahoo.com/search/images;_ylt=AwrKA3G_HAVnwA8DVFu zRwx.;_ylu=Y29sbwNzZzMEcG9zAzEEdnRpZAMEc2VjA3BpdnM-?p=cockpit+of +turbolet+L419+UVP-E&fr2=piv-web&type=E210PH885G0&fr=mcafee#id=3&iurl =https%3A%2F%2Fimgproc.airliners.net%2Fphotos%2Fairliners%2F3%2F5%2F2% 2F1731253.jpg%3Fv%3Dv40&action=click 5. https://skybrary.aero/articles/cockpit-automation-advantages-and-safety-challenges 6. https://skybrary.aero/articles/cockpit-automation-advantages-and-safety-challenges 7. Mosier, K. L., Fischer, U., Morrow, D., Feigh, K. M., Durso, F. T., Sullivan, K., & Pop, V. (2013). Automation, task, and context features: Impacts on pilots’ judgments of human–automation interaction. Journal of cognitive engineering and decision making, 7(4), 377-399. 8. Sheridan, Thomas & Parasuraman, Raja. (2005). Human-Automation Interaction. Reviews of Human Factors and Ergonomics. 1. 89-129. 10.1518/155723405783703082. College of Engineering – Department of Electronics Engineering Main Topic 6: FLIGHT DECK AUTOMATION Group 6 Members: Balcera, Jade Limwel D. Datingaling, John Edward Anthony J. College of Engineering – Department of Electronics Engineering FLIGHT DECK AUTOMATION Automation represents one of the major trends of the 20th century. In many cases automation has provided the desired benefits and has extended system functionality well beyond existing human capabilities. Situation awareness, a person's mental model of the world around him or her, is central to effective decision making and control in dynamic systems. This construct can be severely impacted by the implementation of automation. Complacency overreliance on automation is one major factor associated with a lack of vigilance in monitoring automation. Monitoring problems have also been found with systems that have a high incidence of false alarms, leading to a lack of trust in the automation. In addition to vigilance problems, the fact that operators are passive observers of automation instead of active processors of information may add to their problems in detecting the need for manual intervention and in reorienting themselves to the state of the system in order to do so. Flight deck automation refers to the use of modern technology to automate various functions in the cockpit that were traditionally performed by human operators. While it aims to enhance efficiency and reduce operational costs. This includes systems such as autopilots, flight management systems, and electronic flight instrument systems that assist pilots in navigation, monitoring, and control. A. LEVELS OF AUTOMATION Over the past few decades, automation has transcended its initial applications in manufacturing and extended its reach into sectors like healthcare, transportation, and agriculture. Robotics and autonomy have taken center stage in the development of automated and semi-automated systems, sparking concerns about the replacement of humans in the workforce and the symbiotic relationship between humans and machines in achieving shared objectives. Yet, the extensive use of autonomous functions in both crewed and uncrewed aircraft raises concerns about human operators' reliance on these systems. This dependency often leads to limited understanding of the system functions and reduced control over intervention points for human operators during certain processes. College of Engineering – Department of Electronics Engineering Autonomy characterizes a system's capability to act, function, or decide independently or with limited external influence, embodying self-governance and self-control. It enables entities to function without direct human intervention, adhering to predefined instructions or making decisions based on acquired patterns, rules, or algorithms. Level 0 (No Automation): Pilots handle all tasks without any automation support. Level 1 (Low Automation): Basic automation assists with specific tasks, such as altitude hold, while pilots remain in control. Level 2 (Partial Automation): Automation manages some aspects of flight, like speed or heading, but pilots must monitor and can override the system. Level 3 (Conditional Automation): The system can manage all tasks in certain conditions (e.g., during cruise) but requires pilots to be ready to intervene if needed. Level 4 (High Automation): The system can handle most flight operations without pilot intervention, although pilots must still monitor and take control if necessary. Level 5 (Full Automation): The system operates independently, making decisions without pilot input, essentially eliminating the need for human intervention. B. HUMAN-AUTOMATION INTERACTION DEFINITION Of HUMAN-AUTOMATION INTERACTION Human-Automation Interaction (HAI) is the study of how humans and automated systems work together to accomplish tasks. It involves understanding human capabilities, designing effective automated systems, and creating intuitive interfaces that facilitate seamless communication between humans and machines. HAI aims to enhance efficiency, improve safety, support decision-making, and create a better user experience. College of Engineering – Department of Electronics Engineering IMPORTANCE OF HUMAN-AUTOMATION INTERACTION The importance of Human-Automation Interaction (HAI) lies in its potential to revolutionize various industries and improve our lives. By understanding how humans and automated systems can effectively work together, we can design more efficient and safe technologies, enhance productivity, and support decision-making. HAI can also help address challenges such as job displacement and ethical concerns associated with automation. Ultimately, HAI plays a crucial role in shaping the future of technology and human interaction. BENEFITS OF HUMAN-AUTOMATION INTERACTION Imagine a world where robots do the boring or dangerous jobs, and people get to do the fun and exciting ones. That's the idea behind Human-Automation Interaction. By working together, humans and machines can achieve amazing things. Here are some of the benefits of HAI in Flight Deck Automation. Improved Safety: - Reduced workload: Automation can handle routine tasks, freeing pilots to focus on higher-level decision-making and monitoring. This can help to reduce pilot fatigue and improve their ability to respond to emergencies. - Enhanced situational awareness: Automation systems can provide pilots with real-time information and alerts, improving their understanding of the aircraft's status and the surrounding environment. This can help pilots to detect and avoid potential hazards more quickly. - Reduced risk of human error: Automation can help to reduce the risk of human errors that can lead to accidents. By automating routine tasks, pilots can focus on more complex and challenging tasks, reducing the likelihood of mistakes. College of Engineering – Department of Electronics Engineering Increased Efficiency: - Improved navigation and flight planning: Automation systems can assist pilots in navigation and flight planning, reducing the workload and improving accuracy. This can help to shorten flight times and reduce fuel consumption. - Enhanced communication: Automation systems can facilitate communication between pilots and air traffic control, improving the efficiency and safety of flights. This can help to reduce delays and avoid conflicts between aircraft. Enhanced Comfort and Convenience: - Improved passenger experience: Automation can provide passengers with a more comfortable and enjoyable flight experience, such as automated climate control and entertainment systems. This can help to increase passenger satisfaction and loyalty. - Reduced fuel consumption: Automation can help to optimize flight paths and reduce fuel consumption, leading to lower operating costs for airlines. This can help to make air travel more affordable for passengers. Improved Training and Education: - Enhanced training tools: Automation can be used to develop more realistic and effective training tools for pilots, such as flight simulators that incorporate advanced automation systems. - Improved knowledge transfer: Automation can help to facilitate the transfer of knowledge and expertise between pilots, improving the overall level of skill and competence in the aviation industry. Increased Competitiveness: - Reduced operating costs: Automation can help to reduce operating costs for airlines, making them more competitive in the global aviation market. - Improved customer service: Automation can help to improve customer service by providing passengers with a more efficient and enjoyable flight experience. College of Engineering – Department of Electronics Engineering C. AUTOMATION RISK Automation risk is the likelihood that a particular occupation or job function can be automated. This metric quantifies the potential for computer technology to replace human labor, impacting various industries and job markets. By understanding automation risk, individuals and organizations can anticipate the future of work and make informed decisions about education, training, and economic development strategies. While offering numerous benefits, Automation also poses significant risks that must be carefully considered. Here are some of the automation risks in Flight Deck: - Loss of Situational Awareness: Overreliance on automation can lead to pilots becoming less engaged with the aircraft, potentially reducing their situational awareness. This can make it more difficult for pilots to detect and respond to potential hazards or emergencies. - Complacency: Pilots may become complacent about their ability to manually control the aircraft, leading to slower response times in emergencies. This can be particularly dangerous in situations where automation fails or is not able to handle the situation effectively. - Automation Surprises: Unexpected or unintended actions by automated systems can startle or confuse pilots, leading to errors. This can happen when pilots are not fully aware of the capabilities and limitations of the automation system. - Difficulty in Understanding Automation: Complex automation systems can be difficult for pilots to understand, increasing the risk of misinterpretation or misuse. This can be particularly problematic for new pilots or those who have not received adequate training on the automation system. - Loss of Manual Skills: Reduced opportunities for manual flying can lead to a decline in pilots' hand-flying skills, which may be necessary in certain situations. This can be particularly dangerous in emergencies or when automation fails. - Cybersecurity Threats: Automated systems in flight decks are vulnerable to cyberattacks, which could compromise aircraft safety. This could lead to the hijacking of aircraft or the manipulation of flight controls. College of Engineering – Department of Electronics Engineering REFERENCES https://www.taylorfrancis.com/chapters/edit/10.1201/9781315137957-8/automation-situat ion-awareness-mica-endsley?context=ubx https://www.researchgate.net/publication/240756917_Human-Automation_Interaction https://nationalequityatlas.org/research/analyses https://elite-human-engineering.blogspot.com/2023/11/automation-in-aircraft-systems.ht ml https://skybrary.aero/articles/cockpit-automation-advantages-and-safety-challenges