OCR (A) Physics GCSE Waves in Matter Summary Notes PDF
Document Details
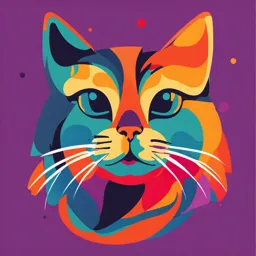
Uploaded by BelovedPine
OCR
Tags
Summary
This document is a summary of waves in matter for OCR (A) Physics at GCSE level. It covers wave behaviour, types of waves, and concepts like reflection, transmission, and absorption. The document appears to be a set of notes, not a full-fledged past paper.
Full Transcript
OCR (A) Physics GCSE Topic P5: Waves in Matter Summary Notes (Content in bold is for Higher Tier only) This work by PMT Education is licensed under https://bit.ly/pmt-cc https://bit.ly/pmt-edu-cc CC BY-NC-ND 4.0...
OCR (A) Physics GCSE Topic P5: Waves in Matter Summary Notes (Content in bold is for Higher Tier only) This work by PMT Education is licensed under https://bit.ly/pmt-cc https://bit.ly/pmt-edu-cc CC BY-NC-ND 4.0 https://bit.ly/pmt-cc https://bit.ly/pmt-edu https://bit.ly/pmt-cc P5.1 Wave Behaviour Wave motion can be described in terms of: Wavelength – the distance between a point on one wave and the same point on the next. Typically measured in metres. aximum displacement (crest Amplitude – the distance from equilibrium line to the m or trough). Typically measured in metres. Frequency – the number of waves that pass a s ingle point per second. Typically measured in Hertz (Hz). Period – the time taken for a whole wave to completely pass a single point. Typically measured in seconds. v elocity = f requency × wavelength = f λ ms−1 = Hz × metres Relationships Increasing frequency, increases velocity (directly proportional). Increasing wavelength, increases velocity (directly proportional). Period is inversely proportional to frequency, so the smaller the period, the higher the frequency and greater the velocity. Types of Waves Transverse waves have p eaks and troughs with the vibrations are at right angles to the direction of travel. Light, or any electromagnetic wave are transverse waves. Longitudinal waves have c ompressions and rarefactions with the vibrationsin the s ame direction as the direction of travel. Sound waves are longitudinal waves. Passing into Different Media A medium is a substance the wave passes through, it could be air, water, glass etc. Density of a medium refers to the o ptically density, not necessarily its physical dense. Frequency of a wave is always constant no matter of the medium. Passing into a denser medium: The speed of the wave decreases, so wavelength decreases because the frequency is constant. Speed decreases because it is travelling through a more dense medium, so it cannot travel as fast. https://bit.ly/pmt-edu-cc https://bit.ly/pmt-cc https://bit.ly/pmt-cc https://bit.ly/pmt-edu https://bit.ly/pmt-cc The energy of the wave must be constant, because of conservation of energy. So this means frequency does not change, colour is dependent on frequency so colour stays the same. Waves at Material Interfaces (Physics Only) At an interface, the wave could either be reflected, transmitted or absorbed depending on the electrons in the material. Electrons can only absorb certain amounts of energy which is directly related to frequency (higher f = more energy). So light of different frequencies interact differently. Reflection Waves will reflect off aflat surface. The smoother the surface, the stronger the reflected wave is. Rough surfaces scatter the light in all directions, so appear matt and not reflective The angle of incidence of a wave = angle of reflection. Light will reflect if the object is opaque and is not absorbed by the material. The electrons will absorb the light energy, then reemit it as a reflected wave Transmission Waves will pass through a transparent material. The more transparent, the more light will pass through the material. It could still refract, but the process of passing through the material and still emerging is transmission. Absorption If the frequency of light matches the difference in energy levels of the electrons, the light will be absorbed by the electrons and not re-emitted except over time as h eat. If a material appears a certain colour, o nly that coloured light has been reflected, and the rest of the frequencies in visible light have been absorbed. Ultrasound When ultrasound reaches a b oundary between two media, they are p artially reflected back. The remainder of the waves continue and pass through. A receiver next to the emitter can record the reflected waves. The speed of the waves is constant, so measuring the time between emission and detection can show the distance from the source at which they were reflected. They can also be used for imaging under surfaces. e.g. A crack in a metal block will cause some waves to reflect earlier than the rest, so will show up. e.g. A foetus scan uses ultrasound as a non-invasive imaging method. https://bit.ly/pmt-edu-cc https://bit.ly/pmt-cc https://bit.ly/pmt-cc https://bit.ly/pmt-edu https://bit.ly/pmt-cc Sonar These waves undergo the same processes as ultrasound, but on a larger scale. e.g. Ultrasound sent from beneath the ship will bounce off the seabed and the time to receive the echo is used to calculate the depth to the seabed. How the Ear Works (Physics Only) The outer ear collects the sound and channels it down the ear canal. As it travels through the canal, it acts as an air pressure wave. The sound waves hit the eardrum which is a tightly stretched membrane causing it to vibrate at the same frequency as the sound as the incoming pressure waves reach it: Compression forces the eardrum inward. Rarefaction forces the eardrum outward, due to pressure. The small bones (hammer, anvil and stirrup) connected to the drum then also vibrate at the same frequency. These small bones act as an amplifier of the sound waves and transfer the compression waves to the fluid in the cochlea. As the fluid moves, small hairs that line the cochlea move too. Each hair is sensitive to different sound frequencies, so some move more than others for certain frequencies. Each hair is attached to a nerve cell. When a specific frequency is received, the hair attuned to that frequency moves more, triggering an electrical impulse to the brain, which interprets this as the sound. Diagram showing the structure of a human ear (bbc.co.uk) Limitation of Frequency Range The human hearing range is 20 - 20,000Hz. The hairs in the cochlea attuned to the higher frequencies can easily die or get damaged. Commonly caused by exposure to constant loud noise over a long period of time. It can also be due to the changes in the inner ear as you grow older. A a result, humans tend to lose the upper frequencies of their hearing range as they get older. We have evolved to hear this specific range of frequencies as it gives us the greatest survival advantage. We cannot hear ultrasound as we do not use sonar to hunt, instead, we have accurate vision. https://bit.ly/pmt-edu-cc https://bit.ly/pmt-cc https://bit.ly/pmt-cc https://bit.ly/pmt-edu https://bit.ly/pmt-cc Ripple Tanks A ripple tank is a shallow glass tank containing a fluid (usually water) with a needle or paddle which o ater waves at a chosen frequency. If light is shone through scillates, producing w the tank, dark and light patches will appear underneath it as light passes through wave crests and troughs. Troughs appear light. Crests appear dark as there is a greater depth of water so the light is scattered the most. By counting the number of times a dark (maximum) passes through a point in a minute, then dividing this by 60 (seconds in a minute), the frequency (number of oscillations per second) can be calculated. Wavelength can also be measured by using a strobe light at the same frequency as the waves, so the pattern of waves appears fixed on the screen. The distance between two maxima can be measured to calculate wavelength in the wave equation: v = fλ Reflection can be shown in a ripple tank by using an obstruction in the tank. lass sheet on part of the tank Refraction can be shown in a ripple tank by placing a thick g floor. The depth of water becomes shallower in that area. Since wave speed depends on depth, the ripples slow down in the shallow area. This is similar to how waves slow down when entering a denser medium. Evidence of Particles Particles travel perpendicular to the direction of the wave’s travel. If a ping pong ball is placed in a ripple tank, it doesn’t get carried by the wave, as the particles simply move up and down, not in the direction of the wave. This provides evidence that it is the wave travelling, not the water itself. https://bit.ly/pmt-edu-cc https://bit.ly/pmt-cc https://bit.ly/pmt-cc https://bit.ly/pmt-edu https://bit.ly/pmt-cc P5.2 The Electromagnetic Spectrum (Need to learn the main groups, and the order for wavelength or frequency.) Electromagnetic (EM) waves are transverse waves meaning they do n ot need particles to move to transfer energy. In space (a vacuum), all EM waves have the same velocity equal to 8 the speed of light (3x10 m/s). Frequency-Wavelength Relationships Speed is constant for all EM waves. Therefore as wavelength decreases, frequency must increase and as frequency increases, the energy of the wave increases. Uses of each group Radio – communications Micro – cooking, as it heats the water or fat in foodstuffs Infra-red – short rangecommunication, remote controls Visible – to illuminate things so we can see them UV – sterilisation, as it kills bacteria X ray – to see through soft tissue and look at skeleton Gamma – used to kill cancer cells in radiotherapy UV, X-ray and Gamma are dangerous as they have a small wavelength, high frequency, and therefore high energy. This high energy means they can cause cells to mutate, potentially causing cancer. This means radiotherapists, who constantly operate with gamma sources, try to maintain minimal exposure by leaving the room or wear lead aprons. Also pilots, flying at high altitudes where there is more UV, are more likely to suffer from cancer. Imaging Non-invasive imaging of the inside the body is one of the uses of EM radiation. Some radiation gets reflected back at each material boundary (such as an organ) and a detector outside of the body can detect the reflections to build up an image. https://bit.ly/pmt-edu-cc https://bit.ly/pmt-cc https://bit.ly/pmt-cc https://bit.ly/pmt-edu https://bit.ly/pmt-cc Higher energy waves reflect less, and just pass straight through the materials or get absorbed. In these cases, the detector is placed on the opposite side of the body to the source. Denser material causes more of the wave to be absorbed, so less energy reaches the detector. Speed of the wave decreases in denser material, therefore reflected signals are stronger from solids compared to liquids. Substances will absorb, transmit, refract or reflect certain EM waves depending on wavelength: glass will transmit or refract visible light, absorb UV radiation and reflect IR radiation. The material interacts differently for different parts of EM spectrum because the wavelengths (and frequencies) are different. Some effects are due to differences in velocity. When light enters a denser medium, it slows down. Shorter wavelengths slow down more than longer wavelengths. White light passing through a prism leads to diffraction as the different wavelengths refract different amounts, and therefore spread out creating a rainbow effect. Diffraction of white light through a prism (gcsescience.com). Colour Objects appear a certain colour because only a certain wavelength of light colour is reflected by the object. All the other wavelengths are absorbed. Colour filters can be used to only allow one wavelength of light to be transmitted. All other colours are absorbed. https://bit.ly/pmt-edu-cc https://bit.ly/pmt-cc https://bit.ly/pmt-cc https://bit.ly/pmt-edu https://bit.ly/pmt-cc Lenses (Physics Only) For reflection off a plane surface, the a ngle of incidence (i) = angle of reflection (r). These angles are always measured from n ormal. Reflection off a plane surface (aplusscience.com) enser material, it bends intowards For refraction at a plane surface, if the wave enters a d the normal. If the wave enters aless dense material, it bendsaway from normal. Refraction through a glass block (gcsescience.com) Lenses are generally drawn as a d ashed vertical line.Focal points are points either side of the lens and are the points at which light c onverges. If light passes through the centre of a lens, it does not change direction. Concave Lenses These lenses cave inwards so they are thinner at centre than at edges. Concave lenses spread the light outwards. Light appears to have come from the focal point: Draw horizontal ray from top of object to lens. Draw a faint line from focal point to point where the ray hits the lens. The ray exits the lens along the same direction as the faint line. https://bit.ly/pmt-edu-cc https://bit.ly/pmt-cc https://bit.ly/pmt-cc https://bit.ly/pmt-edu https://bit.ly/pmt-cc Concave lens diagram (gcsescience.com). Concave lenses are used to spread out light further for things such as correcting short-sightedness. Light is focused in front of the retina, so needs to be spread out slightly to be able to be focused o n the retina. Convex Lenses These lenses bulge o utwards so they are thicker at centre than at the edges. They focus the light inwards. Draw horizontal rays to focus onto the focal point. Convex lens diagram (gcsesciene.com). Convex lenses are used to m agnify things, for example in magnifying glasses and binoculars. They are also used to correctlong-sightedness, as it focuses the rays closer. https://bit.ly/pmt-edu-cc https://bit.ly/pmt-cc https://bit.ly/pmt-cc https://bit.ly/pmt-edu https://bit.ly/pmt-cc