Global Change Summary PDF
Document Details
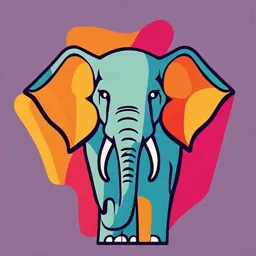
Uploaded by RegalDysprosium7795
Tags
Summary
This document provides a comprehensive overview of global change, focusing on various aspects including climate change, its impact on coral reefs, and other environmental issues. It details the drivers of global change, encompassing both natural and human influences, particularly emphasizing the significant role of human activities since the industrial age.
Full Transcript
Inhalt {#inhalt.Inhaltsverzeichnisberschrift} ====== [1. Introduction 1](#introduction) [2. Climate change overview 3](#climate-change-overview) [3. Climate change and coral reefs 5](#climate-change-and-coral-reefs) [4. Climate change and fire 12](#climate-change-and-fire) [5. Climate change an...
Inhalt {#inhalt.Inhaltsverzeichnisberschrift} ====== [1. Introduction 1](#introduction) [2. Climate change overview 3](#climate-change-overview) [3. Climate change and coral reefs 5](#climate-change-and-coral-reefs) [4. Climate change and fire 12](#climate-change-and-fire) [5. Climate change and permafrost 19](#climate-change-and-permafrost) [6. Glacier, ice sheets and climate change 23](#glacier-ice-sheets-and-climate-change) [7. Ocean acidification 30](#ocean-acidification) [8. Human impacts on the global freshwater cycle 36](#human-impacts-on-the-global-freshwater-cycle) [9. Natural climate solutions 41](#_Toc185526785) [10. Pollution as a global driver 48](#pollution-as-a-global-driver) [11. Biodiversity loss and ecosystem functioning 54](#biodiversity-loss-and-ecosystem-functioning) [12. Biological invasions 60](#biological-invasions) Introduction ------------ **What is global change?** - Planetary-level changes in the Earth system - commonly used to describe changes caused by humans, particularly since the mid 20th century (the so-called Great Acceleration) - More than climate warming - intersection of 'Ecology', 'Earth Science' and 'Environmental Science' **Main drivers of planetary change have been (prehistorically)** - *Natural forces: plate tectonics, volcanism, Earth's axial tilt and orbit, solar radiation shifts, and meteorite impacts (the latter paving the way for human dominance).* - *Human influence:* - ***Prehistoric**: Homo sapiens emerged \~300,000 years ago, with agriculture and animal domestication beginning around 10,000 years ago.* - ***Industrial Age**: Major shifts occurred with the Industrial Revolution (\~250 years ago) and the Haber-Bosch process (1909), which enabled large-scale agriculture and fueled population growth* **The great acceleration** - refers to the rapid and unprecedented changes on Earth over the last 75 years - shifts in Earth's systems are far beyond what has happened in the past 500,000 years **Drivers of human population growth** Ein Bild, das Text, Screenshot, Schrift, Reihe enthält. Automatisch generierte Beschreibung **CO~2~ (and methane) enrichment causes climate change** - Greenhouse gas molecules absorb a wider range of wavelengths, including infrared (heat), and re-emit this energy -- half of this re-emitted energy returns to earth as heat **N deposition changes over time** - Asia, industrialization, still going up - Europe, policies, past industrialization, mitigation, n going down **Global Change Drivers -- the 'Big Five'** - Carbon dioxide enrichment - Climate change (most important in 200 years) - Land use (most important currently) - Nitrogen deposition - Biotic exchange (extinctions, invasions) - Others: Human population growth, light pollution, overfishing,\... **Anthropocene** - Proposed geological epoch defined by significant human impact on Earth's geology, landscape and ecosystems through global change - Start date debated but probably start of mid 20th century - Marker: 1950 layer with spikes in plutonium, pollen, and diatom shifts. - Debate doesn't lessen the importance of the term \"Anthropocene.\" **Planetary boundaries** - Framework defining limits to human impact on Earth. - Crossing boundaries risks loss of environmental self-regulation. - Could push us out of the stable Holocene era. - Based on \"tipping points\" and \"system resilience.\" - Concept is appealing but difficult to measure accurately due to data gaps. **Ecosystem services** - Services provided by ecosystems upon which humanity depends - Threats to functioning ecosystems compromise their ability to deliver ecosystem services  Climate change overview ----------------------- **CO2** - Winter: CO₂ levels are higher in the Northern Hemisphere due to the absence of a growing season, which limits plant-based CO₂ absorption. - Summer: CO₂ becomes more evenly distributed globally, as plants actively absorb it during their growing phase. - Atmospheric CO₂: Currently at 422.17 ppm, CO₂ constitutes about 0.04% of the Earth\'s atmosphere. **Keeling curve** - shows the ongoing rise in atmospheric CO₂ levels, with seasonal fluctuations, measured since 1958 - Seasonality of vegetation in Alaska leads to higher variability **ECS (Equilibrium Climate Sensitivity)** - Refers to the projected global temperature increase if CO₂ levels double from pre-industrial levels and the climate reaches a new equilibrium. - Measures the climate system\'s sensitivity and long-term warming effects in response to doubled CO₂ concentrations **Radiation, Sun and Earth** - Sun emits mostly shortwave radiation (UV and visible light), which passes through the atmosphere more easily. - Earth absorbs this energy and re-emits it as longwave infrared radiation. - However, certain gases in the atmosphere---like water vapor, CO₂, and methane---absorb much of this infrared radiation - trapping heat and contributing to the greenhouse effect. - Only specific \"atmospheric windows\" allow some infrared radiation to escape directly into space, helping to balance Earth\'s temperature. **Greenhouse effect** - Without the (natural) greenhouse effect, earth would be around 30° C colder - Water vapour, CO2, CH4, N2O, O are the most important greenhouse gases today - Water vapour mainly driven by temperature (warmer air holds more water) - Human activities drive increases in CO2, CH4 and more **IPCC AR6 WG1 some conclusions** - CO₂ Levels: Highest in 2 million years. - Sea Level Rise: Fastest in 3,000 years. - Arctic Sea Ice: Lowest in 1,000 years. - Glacier Retreat: Fastest in 2,000 years. **Future climate scenarios AR6** - SSP1: Sustainability - SSP2: Middle of the road - SSP3: Regional Rivalry - SSP4: Inequality - SSP5: Fossil-fueled development Climate change and coral reefs ------------------------------ **Coral reefs in numbers** - Corals have been around for hundreds of millions of years - Cover 1,554,000 km\^2 - \~90,000 species found on coral reefs - 25% of all fish species use corals in some kind of way (feeding, hatching...) - Half the calcium that enters the ocean ends up in reef systems **The value of coral reefs** - Ecosystem services are worth £65 billion per year - Services: - Food - Medicine - Building material - Tourism - Biodiversity - Cultural value - 500 million people directly benefit from reefs - 30 million people completely rely on reefs for their livelihood **What is a coral?** - small marine invertebrate - colonies of polyps, not organism - Polyps build hard skeletons of calcium carbonate. - Corals host Zooxanthellae (algae) that provide food and color. - Composition: - Most mass is rock - Coral is animal - Plant is algae **Endosymbiontic relationship** - Coral host - Provides shelter - Provides nutrients - Zooxanthellae - Provide energy from photosynthesis - Variable temperature tolerance **Coral reproduction** - Sexual - Most broadcast spawners - Many hermaphroditic (75%), some have separate sexes - Simultaneous Gamete Release: Male and female gametes are released at the same time. - Genetic Diversity: widespread dispersal of gametes. - Most species or genera have staggered spawning times to prevent interbreeding - Hybrids still common - Some brooders - Produce eggs and sperm internally to form planula larvae - Asexual - Fragmentation - Each fragment becomes a clone - Not on purpose, but happens and can be used for intentional reproduction for conservation purposes - Polyp Diversity: Polyps within a coral colony are genetically identical (clones) due to asexual reproduction, but they can specialize in different roles (e.g., feeding, defense, or reproduction). So, while genetically the same, polyps within a colony may serve distinct functions. **Coral skeleton** - Coral skeleton = CaCO3 as aragonite in crystalline form (calciumcarbonate/limestone) - aragonite is more susceptible to acidification - high risk - Process of calcification releases CO2 - ongoing debate if corals are sinks or sources - depending on the situation - can be CO₂ sources, especially when isolated from algae. - Not Easily Replaced: Corals offer critical benefits beyond CO₂ absorption, so replacing them with algae isn\'t straightforward **Coral morphology** - Corals have a varied morphology - one species might grow differently, depending on where and what the situation is - eg: in deeper water might grow more like a plate to capture a s much light as possible - This determines their resilience to environmental conditions (e.g. wave action) - Different morphologies suit different parts of the reef - Can be a critical part of their life-history strategy - Massive coral - growing like a boulder - slow growing, long living - more tolerant to diverse conditions - Free-living corals - no colonies, but one singular polyp - can be moved around - represents what corals looked like in past - other forms: Branching coral, Table coral, Blade-like coral, Columnar coral, plate-like coral, foliose coral **Reef-building or not** - Hermatypic corals: reef-building and contain zooxanthellae - Ahermatypic corals: NOT reef-building and tend not to contain zooxanthellae **Coral reef distribution** **Reef structure** - Reefs occur in different sizes and shapes due partly to - Hydrological conditions - Geological conditions - Can be grouped into 4 general categories - Atolls - Barrier reefs (barrier between land and ocean) - Fringing reefs - Patch reefs / coral bommies **Atolls** - Very old structures (eg. 60 million years) - Darwin's subsidence theory Ein Bild, das Text, Screenshot, Schrift, Algebra enthält. Automatisch generierte Beschreibung **Barrier/fringing reefs** - Quite young (\ - Growth is a product of carbonate deposition and erosion (bioerosion (Fish eating corals and turning into sand poop) and dissolution) **Reef bommies** - Overgrowth of solitary or massive corals - Leads to high diversity in a localised area - Can be statig point of barrier reef - Can establish through corraliths (free living) - Might have free space on them and at some point it gets to hevy and then other corals can settle over time bommie established **Coral reefs aren't just corals** - Other components - Seagrass - Fleshy macroalgae - Calcifying algae - Coralline algae **Coralline algae** - RCA are vital for holding the reef structure together - Crustose coralline algae can contribute more than corals to coral reef carbonate production - Reef cement - RCA cementation gives protection from wave action - RCA are the deepest known photosynthesisers: 300+ m depth! - Free-living RCA: maerl/ rhodoliths - A reef-like habitat in their own right - Important for ecosystem service provision **Threats to coral reefs** - Temperature (todays biggest contemporary threat), marine heatwaves - Acidification - High Salinity/low salinity - Fishing, plastics - Extreme weather events **Coral bleaching** - In response to environmental stress - Phenomenon where corals lose their symbionts - Bleached corals are still alive and can survive without their algae if they obtain enough food - in areas where food is scarce, algae contribute up to 90% of their energy, - bleached corals more vulnerable to starvation and disease. - Causes - Ocean warming (heatwaves also becoming more frequent & extreme) - Ocean acidification - Solar radiation & ultraviolet radiation - Disease - Chemical pollution - Too much sedimentation **Coral recovery from bleaching** - Is possible - Takes time and needs to happen before starvation - Bleached corals have slower growth and productivity - Natural process - evolutionary advantage - contemporary climate change and human stressors are pushing corals to the limit - possible to intake a more fitting zooxanthellae to the climate and surrounding conditions - Restoration Climate change and fire ----------------------- **Prehistoric driver fire** - Charcoal deposits from 420 million years ago mark the emergence of land plants. - In the Carboniferous (300--360 million years ago), fires driven by high oxygen levels and natural ignition shaped gymnosperm evolution. - Fires facilitated by lightning, volcanism and climate has been an important evolutionary driver of land plants. **Secondary succession** - Soil remains speeding the succession process - Recovery begins with fast-growing pioneer species (grasses, shrubs), followed by intermediate species (young trees), eventually leading to a stable, mature ecosystem. **Need for fire** - Many organism naturally depend on fire - Seeds - Seritonous seeds that are sealed by resins ( banksia eucalyptus) - opened by fire - Cones that need fire to open (pinus contorata) - Smoke - Smoke from fire triggers seed dormancy break in many plants, promoting germination in nutrient-rich, low-competition post-fire environments - Animals - Some fire-adapted beetles (pyrophyllic), like the black fire beetle (Melanophila acuminata), have infrared sensors to detect burning wood from kilometers away. - Red-cockaded woodpecker: - Endangered - prefers pine, which is favoured by fire - prefers softer wood, created by fungi following fire - creating cavities for other organisms - Fungi - genus Pholiota need fire in order to produce fruiting bodies **Adaptations to fire** - Thick bark - Epicormic branches - Clonal spread - Significant underground structures - Resprouting **Fire releases nutrients from soil** - Initially -- pulse of mineral N and P released, excess nutrients available - Leads to initial depletion of N and P capital in the soil, which is replenished over time - Eventually N and P are immobilized in plants and recalcitrant OM, leads to lower availability **Global change as a driver of fire** - Land use -- deliberate fire supression so [fewer fires] - Climate warming -- drier and warmer conditions so [more fires] - Fire supression can also lead to fuel accumulation so [more severe fires] when they occur **Intensity of fire depends on** - amount of fuel - moisture content - arrangement - wind speed - weather (temp and humidity) - topography and atmospheric conditions - \\>Several of these factors are driven by global change factors **Unusually intense fires lead to biodiversity loss** - Food sources are lost for herbivores and insectivores, leading to less predators - Loss of habitats and also habitat corridors - Dominant plants, and frequently their seed sources, are lost - Loss of soil organic matter and nutrient capital, and thus fungi and invertebrates needed for nutrient cycling **Areas that burn now, that have never burend before** - Siberian arctic on permafrost - Alaska - Greenland - Tropical peat forests in southeast asia **Multiple drivers of more frequent Arctic fires** - Rising of: - Air temperature - Vapor-pressure deficit - Mean NDVI (measure of the amount and vigor of vegetation on the land surface) - Climatic water deficit - Surface temperature - Length of season - Number of ignitions **Massive losses of cabon- peatlands se asia** - Southeast Asia\'s peatlands store 77% of tropical peat carbon and over 50% of the region\'s forest soil carbon. - 50% cleared in 30 years, mainly for oil palm and Acacia plantations, causing \~0.3 Gt carbon loss annually. - Drained peatlands are flammable; 1997--1998 El Niño fires made Indonesia the third-largest greenhouse gas emitter globally **Greenhouse gas emission from degradation of peatland and land use**  **Effects of burning peatlands** - Regional pollution - Transboundary smoke haze is a major health hazard - Premature deaths **Restoring carbon and nutrients in degraded peat forests** - Blocking drainage channels and paludification - Need to restore vegetation but is extremely difficult for various reasons (perhaps they have transcended a 'tipping point') **Ground vs crown fires (effects)** Ein Bild, das Text, Origami, Darstellung, Design enthält. Automatisch generierte Beschreibung mit mittlerer Zuverlässigkeit **Significance of boreal forest for C sequestration** - Most C in boreal forests is in the soil - Fire determines whether the soil is a sink vs source of C  **Post fire succession** - Starts with relatively fast growing plants that like the pulse of nutrients and light post-fire, - Over time, slower growing plants establish -- these can tolerate lower availability of nutrients and frequently invest in secondary compounds. Decomposition is slowed down and nutrients are locked up - Process is reset and locked up nutrients are released through the next fire. Ein Bild, das Reihe, Diagramm, Text, Schrift enthält. Automatisch generierte Beschreibung  \#\#\# Fire Management Impacts \#\#\#\# \*\*British Heathlands\*\* \- Fire prevents grassland takeover and supports heathland species. \- Biodiversity is higher on cut sites; a mosaic of management is most effective. \#\#\#\# \*\*Northern Sweden\*\* \- Wildfires boost forage diversity and abundance for a decade, benefiting moose. \- Prescribed burns are less effective but can improve forage if they mimic large wildfires in size and severity. \#\#\#\# \*\*Australia\*\* \- Prescribed burns reduce wildfires for up to 6 years but harm biodiversity if too frequent. \- Small, targeted burns near infrastructure are more effective than large-scale burns. \- Adaptive management is essential to balance fire control and biodiversity protection. In total: - dependend on the area and ecosystem \- dependend on what the goal is \- maybe prescribed burning needs to evolve, more complicated than just doing it and being done \- mosaic and variability should be used Climate change and permafrost ----------------------------- **Permafrost** - Definition: "Ground (soil or rock and included ice and organic material) that remains at or below 0°C for at least two consecutive years" - Defined by temperatur, not if it's frozen! - Often contains ice, mineral particles (rock), and organic material. **Active layer** 1. \- Definition: "The layer of ground that is subject to annual thawing and freezing in areas underlain by permafrost" \- Strictly not defined by temperature, but whether or not it remains frozen. \- The active layer is typically a few decimeters up to a few meters thick, depending on climate, substrate, and water content. **Soil with permafrost temperature curve and composition** - The more you go to the surface, the more the seasonal variation is visible **Heat transfer basics** - Thermal conduktivity(k) - W/(mK) - *The abilityof a material to conductheat.* - Heat capacity(C) - J/m3K (volumetric) (or J/kgK(specific)) - *The amount ofheatrequired to change a substance\'stemperatureby a given amount.* - Thermal diffusivity = k/C - m2/s - *Materials with high thermal diffusivity rapidly adjust their temperature to that of their surroundings since they conduct heat quickly and do not require much energy to reach thermal equilibrium.* **Latent heat (of fusion)** When permafrost reaches 0°C, the energy supplied is not immediately used to increase the temperature further. Instead, it is used for the **phase change**---melting the ice into water. This energy is called the **latent heat of fusion** **Freezing water** 1. \- Pure water freezes at 0°C (normal pressure) \- Salt lowers the freezing point \- In fine grained soils, water is adsorbed to particles which lowers the freezing point **Cyrosuction** migrating water moving towards frozen front- leading to build up of pure ice chunks \- in some soils water moves very slow and freezes very slowly \- frost susceptibl soils are good at moving water in freezing state \- happens on both sides of the freezing front **Frost-susceptible soils** - Certain pore sizes promote capillary flow in the ground, enabling cryosuction. Soils with high silt content are frost-susceptible. - Soils are often heterogeneous, leading to varying ice content (in layers). - Access to water needed to build up large quantities of ground ice. **Syngenetic permafrost** - Simultaneous Formation: - Sediments accumulate at the same time as the permafrost develops, leading to layers of frozen ground that grow progressively over time. - As the sediment builds up, the permafrost often becomes rich in both ground ice (in various forms such as ice lenses) and organic matter, preserved due to freezing conditions. - Loess, a fine-grained, windblown (aeolian) sediment, is frequently associated with syngenetic permafrost. Its deposition provides an ideal environment for permafrost development in cold climates. - Rapid climate warming in the Arctic - Warming is faster than thawing (latent heat exchange) - Upper layers not warming in same rapid gradient, because close to 0°C - Wide range of projections for future permafrost distributions (reductions from 14 to 92 % in extent year 2100) - huge amplitude, partly because studies looked at different depths of permafrost (first 5 meters, 100 meters, etc.) - Future depends on what climate is to be expected and depths are of interest to us **Water change with permafrost thaw** - New paths for groundwater due to thawed permafrost - More released material ( sulfate, iron, acid ,trace metals) into groundwater and streams, because material gets exposed to chemical weathering **Landslides and permafrost** - problems with landslides due to active layer sliding away in summer **Permafrost in Sweden** - Permafrost can be found high up in the mountains and in some northern peatlands, due to the special thermal properties of peat. - It is thin, warm (close 0° C), and rapidly changing due to warmer climate **Permafrost and carbon cycle** - Top 3 m of ground in permafrost areas estimated to contain 1,000 ± 200 Pg (1 Pg = 1,000 Tg) organic carbon and 55 Pg nitrogen. - Deeper deposits contain 400--1,000 Pg C, making permafrost areas the largest terrestrial C and N pools. - Thawing of permafrost can lead to increased emissions of greenhouse gases (CO2, CH4 och **Gradual thaw**- slow gradual defreezing of permafrost from the top **Abrupt thaw**: very local and abrupt process, causing land sinking, then the upper will slied away and freilegen deeper permafrost Glacier, ice sheets and climate change -------------------------------------- **What is a glacier** - Having a big amount of ice that has a movement towards the ablation zone and glacial front - Form in cold climates (high latitude or elevation) - Balance between snowfall and glacier melt (mass balance) - Increased snowfall --glaciers grow - Increased melt (or calving) --glaciers shrink - Ongoing changes in temperature and precipitation impact glacier mass balances - Often not permafrost beneath glacier - All overall latitudes in high elevation, more in north - Zones: - Accumulation zone: snowfall - Equilibrium: between accumulation (snowfall) nd melting - Ablation zone: melting and sublimation( change in aggregate state from ice to gas directly) Trends: - Mass budget decreasing in all regions - Scandinavia: high variability, need to study for long time to see trend - Increased ouflow water due to higher metling rates, but after some time less runoff due to missing ice that have already been melted **Glacier and ice types** - **Mountain glacier** - **Ice sheets** - Greenland and Antarctica (today) - An ice sheet is a mass of glacial land ice extending more than 50,000 square kilometers - Ice speed can be an indicator for something happening - Greenland one outlet of it is biggest iceberg producer - Coast very different from antarctica - Greenland: coast land is rock - Antarctica: coast line is ice shelves, so ice right into the ocean - **Ice shelves** - Can form from ice sheet and sea ice - Basal melting: melting from underneath due to warmer water - **Tidewater glacier** - fed by snow, flow out of the mountains and down to the sea - break off or calve into saltwater at sea level, and a few others that reach the sea at high tide only. - Can change quickly - **Sea ice** - Is not a type of glacier, but frozen sea water - It reacts strongly to global warming and have important feedback to the climate system (e.g. albedo) **Calving** - Marine terminating glaciers (tidewater glaciers, ice shelves) lose mass through calving (icebergs breaking loose) **Buttressing** - Ice shelves around Antarctica are up to 50,000 km2in size, and can be up to 2000 m thick. Glaciers that feed into ice shelves are held back by the ice shelf in front of them(buttressing) **Can melting of ice sheets be reversed?** - Melting way faster than restoring of ice, but not impossible - Easier to maintain ice sheet at higher elevation, there is lower temp - Harder to rebuild ice from down there , because it is warmer there **Will the gulf stream shut down?** - Gulf stream is a warm current - Expected to weaken but not cease - This slowdown will affect regional weather and sea level **Earth ice imbalance (Slater et al)** - Total ice loss 28 trillions tons (1994-2017). - Loss contributions of different reservoisres in decreasing order: Arctic sea ice, Antarctic ice shelves, mountain glaciers, the Greenland ice sheet, the Antarctic ice sheet, Southern Ocean sea ice - The Earth\'s cryosphere initially contained approximately 1,740 trillion tons of ice, distributed as follows: - Antarctic Ice Sheet: (75%) Greenland Ice Sheet: (14%), Glaciers and Ice Caps: (4%), Sea Ice and Permafrost: The remainder (7%) - The loss represents about 1.6% of the Earth\'s total ice mass over 23 years - 58 % of the caluclated ice loss comes from northern hemisphere (consider why this could be) - Eventhough Less ice on northerh hemisphere? - Faster warming in northern hemisphere - Northern hemisphere ice is more fragmented - Northern hemisphere higher levels of industrial activity, urbanization, and air pollution - Glacier Retreat: Sensitive glaciers (e.g., in Alaska, Himalayas) added significantly - Arctic Amplification: The Arctic\'s geography (ocean surrounded by land) accelerates warming, with exposed ocean surfaces amplifying heat absorption and regional temperature increases. - Ice loss reflects global reach of climate change - Change of rate of ice loss (57% since 1990), also AIS increase. Dramatic acceleration in mountain glacier (triple between 1970s and 2010) - Loss of grounded ice to sea level vs loss of sea ice to albedo - Sea level 35 mm change from ice melt so far (since 1990s) - Monitoring ice dynamics effects on coastal communities and ecosystems - Atmospheric vs oceanic melting needs to be understood to accurately predict how cryosphere will respond to continued warming. **Atmospheric vs. Oceanic Melting** 1. **Atmospheric Melting**: Caused by warmer air, leading to surface ice melt (e.g., Greenland, glaciers). 1. significant for Greenland and glaciers now and in the future. 2. **Oceanic Melting**: Driven by warm ocean currents eroding ice from below (e.g., Antarctic ice shelves). 2. major contributor to Antarctic ice loss, especially by 2100 and beyond. 3. **Key Regions**: Atmospheric dominates in the Arctic; oceanic in Antarctica. 4. **Feedbacks**: Atmospheric melting reduces albedo; oceanic melting destabilizes ice flow. **Short-long term variability ice sheets (Hanna et al.)** - Need for enhanced monitoring and modelling (NASA and ESA RS data). Need for in situ observations (expensive but really necessary to validate modeling). Need to incorporate short and long term variability in the same models - Some short term melt events can be very impactful and need to be understood to better predict long term changes. - Melting in Antarctica mostly basal and oceanic, different from Greenland (ENSO and similar atm/ocean circulation has effect on Antarctica). But short term AIS melt events impacted also by extreme weather/circulation events - There are several feedback loops between ice sheets and the climate system, both positive and negative but most are positive. - High emission and low emissio scenarios for 2100 not too different, but melt continues beyond 2300. - 1.1 mm per year from ice sheets to sea level rise, currently. **Changes in these ice sheets over geologic time scales. How do they compare with our predictions of future change?** - Over geologic time, ice sheets have grown and retreated slowly with natural climate cycles, spanning thousands to millions of years - current and predicted changes due to human-driven warming are occurring much faster---up to hundreds of times the natural rate - leading to rapid ice loss and significant sea-level rise within decades to centuries. **Main Ice Reservoirs** 1. Antarctic Ice Sheet: 90% of Earth\'s freshwater ice. 2. Greenland Ice Sheet: 7% of Earth\'s freshwater ice. 3. Mountain Glaciers: In the Alps, Himalayas, Andes, etc. 4. Sea Ice: Floating ice, doesn\'t contribute to sea-level rise directly. 5. Permafrost: Contains frozen water, impacts climate but not sea levels directly. **Sea Level Rise Projections** 1. **Antarctica**: - **Now**: \~0.25 meters. - **2100**: 0.3--1 meter. - **2300**: 3--5 meters. 2. **Greenland**: - **Now**: \~0.2 meters. - **2100**: 0.1--0.3 meters. - **2300**: Up to 7 meters over millennia. 3. **Mountain Glaciers**: - **Now**: \~0.6 meters. - **2100**: 0.1--0.2 meters. - **2300**: 0.5--1 meter. **Role of Ice in Global Energy Imbalance** - **Albedo**: Ice reflects solar radiation; melting exposes darker surfaces, absorbing more heat. - **Heat Absorption**: Melting ice absorbs heat, worsening the global energy imbalance and accelerating climate change. - **3% of excess energy accumulates in ice (Ocean**:91, Land 5, Atmosphere:1) Ocean acidification ------------------- **General process/facts of ocean acidification** - CO2 dissolving into the ocean acidification - 30% of CO2 released from fossil fuel burning in oceans - Otherwise climate crisis would be much worse - But it is a huge problem for ocean chemistry - Half of the anthropogenic CO~2~is contained within the top 400 m of the water column **pH value** pH = -log~10~\[H^+^\] - Hydrogen ion concentration - Log 10 scale so change from 8 to 7 is ten times more acidic - First doesn't seem much because the trend shows just small numeral change, but actually it's a lot (log scale!) - **Ocean pH trends** - Earlier: 8.1-8.2 - Now: 8.0-8.1 - Still alkaline, but trend visible - Future: 7.8-7.7 - 150+% increase in pH - 50+% decreaseincarbonateion availability - Current change: - Overwhelms natural variation over the past 800,000 years - Rate unprecedented based onrecordsfromthepast300millionyears - Already a 30+% increase in acidity since Industrial Revolution **Ocean carbonate chemistry** Ein Bild, das Text, Screenshot, Schrift, Zahl enthält. Automatisch generierte Beschreibung  **Total dissolved inorganic carbon** - total carbon available in water for processes like photosynthesis (carbon for plants underwater!) - Includes dissolved carbon dioxide, bicarbonate and carbonate  **Total alkalinity** - indicates how the system resists pH changes and how accessible carbonate is for shell-building Ein Bild, das Text, Schrift, weiß, Algebra enthält. Automatisch generierte Beschreibung **Carbonate Saturation** - how much carbonate ion is available in water compared to what's needed for minerals like calcium carbonate to form or dissolve. - critical for organisms like corals and shellfish that build their skeletons and shells using calcium carbonate - Low saturation (caused by ocean acidification or low alkalinity) threatens marine life that depends on CaCO3  - Ω\>1: Water is **saturated** with carbonate; organisms can build shells/skeletons - Ω=1: Equilibrium; CaCO3 won't form or dissolve - Ω\ - Irrigation (more vapour) and deforestation (less vapour) is same size, but in the other direction, cancelling each other out globally seen - Deforestation), which has decreased global vapor flows from land by 4% (3,000 km³/yr), is as significant a driver of changes in the hydrological cycle as irrigation. - Regional differences - The total global vapor flows for potential vegetation are estimated at 67,000 km³/yr, with humid tropical regions contributing the most, highlighting their critical role in Earth System functioning. - Modifying vapor flows in the Indian Ocean basin increases the risk of changes in the Asian monsoon system. - sub-Saharan Africa, increased food production will most likely come from further expansion of agricultural land through deforestation - Irrigation widespread in South Asia, vapor flows have already increased substantially Climate change: **Anthropogenic climate change has influenced global river flow seasonality (1960/70s-2015)** - Anthropogenic climate change has influenced global river flow seasonality in norther high latitudes - Significant Decreasing in seasonality of river flow - Decrease in snowfraction due to ealy snow melt, - Increasing low flows and decreasing high flows - If there is a n early spring greening there is earlier water intake - - more seasonality in brazil - influence on monsoon, influenceing multiple factors **surface Water body changes (river, lake, resevoirs, dams, etc.)** **High-resolution mapping of global surface water and its long-term changes** - not uniform changes - some places with increased water bodies - some places: water scarcity - All continental regions show a net increase in permanent water - Much coming from reservoir filing, also climate change implicated - except Oceania, which has a fractional (one per cent) net loss. - Over 70% of global net permanent water loss occurred in the Middle East and Central Asia, linked to drought and human actions including river diversion or damming and unregulated withdrawal. - Losses in Australia and the USA linked to long-term droughts are also evident. - Northern latitude has most surface water - More than half of the lakes 44° north - On average 3% land is covered by surface water - Using landsat ### Natural climate solutions **Natural climate solutions -- definition** - *conservation, restoration and improved management strategies (pathways) in natural and working ecosystems with the primary motivation to mitigate GHG emissions and remove CO~2~ from the atmosphere* **Benefits provided by NCS** - 7 Gt CO2 reduction - 60 % avoidance, 40 % restoration Natural pathways for climate mitigation by NCS - Forests - Reforestation - Avoided Forest conversion - Natural forest management - Improved plantations - Avoided woodfuel - Fire management - Agriculture and Grasslands - Biochar - Trees in croplands - Nutrient management - Grazing (Feed, Animal management, legumes,..) - Conservation Agriculture - Improved Rice - Avoided grassland conversion - Wetlands - Coastal restoration - Peat restoration - Avoided peat impacts - Avoided coastal impacts **Most promising pathways for climate mitigation by NCS** - Protecting of existing ecosystems - Management of ecosystems - Restoration of degraded ecosystems - Key focus on: - Carbon sequestration - Biodiversity **Goals for restoration - policy** - Convention on Biological Diversity (CBD) in COP15 (Montreal, 2022) - at least 30% of degraded ecosystems should undergo ecological restoration to improve their function by 2030. - doubling of the previous Aichi 15 target for 2020 of 15% which was not achieved **Achievement of targets of the Convention of Biological Diversity (CBD) requires** 1. Translation of CBD targets into national targets 2. Implementing systematic monitoring 3. Adaptive management **Forests in NCS** - General - Key NCS (especially protecting natural forests for sequestering C) - According to The Nature Conservancy, 'forests offer the single largest \"natural climate solution\" - Boreal , most c belowground, tropics: most c aboveground - Stopping deforestation - Deforestation=12-20% of greenhousegas emissions - Mitigating this is an obvious ncs - Especially in tropics - Main c stocks: warm and tropical regions - Boreal forests as c sinks - Due to tropical forest degradation, the main C sink now is temperate and boreal forest - But the C sink potential of boreal forest faces its own threats, primarily through fire - Ground fires burn off humus and thus cause losses of soil C - Promoting the role of forests as c sink - Fire suppression- not good - Controlled burning - planting - Tree planting as solution - C sequestration - Negative - Planting non-forested areas - Trees are non-native to the system and might be invasive - Trees might not promote C sequestration, due to C loss from fire or from the soil - Tree invasions often impair biodiversity, economic opportunity, water loss - Lack of available land for tree planting - There isn't 900 million ha of free land - Much of it is under agriculture - Risks of afforestation surplanting land for agriculture and food security especially in the Global South - Planting schemes not well thought out - often ineffective (and associated with high mortality) if: - Choice of species for the conditions are not thought out well - There is insufficient follow-up or monitoring - Planting single species vulnerable to disease - Improper matching of species to local scale site conditions - Lack of after-planting care like watering - Trees are commercial commodity - Planted trees are therefore often harvested - Harvesting, and final fate of timber and paper products, might all work against C sequestration - Native systems - Natural forests usually have more tree species (biodiversity) than planted forests - More tree species leads to greater resource utilization (through 'resource partitioning') and thus more biomass and carbon sequestration - - **Tree planting-saving the planet?** - Best way to reduce atmospheric CO~2~ is to reduce emissions in the first place, not to plant up 900m ha of land in trees - Next best way is to focus on preserving C already stored in existing ecosystems and halting its degradation - Extensive tree planting has a lot of potential but - **extensive tree planting helps as a NCS if:** - Species are chosen carefully to match environmental conditions - Species are planted at right time of the year - First plant early successional trees - Areas are avoided that are naturally not forested - Seedlings are monitored, watered, looked after and not abandoned - Local communities are involved and their sources of livelihood are respected. - Seen as more than just a 'numbers game' - - Tree planting - Study by Bastin - Study by Bastin: room for 900 million ha more forest - But potential canopy cover may shrink by 223 million ha by 2050 - Claim (later removed from article) that *'global tree restoration is our most effective climate change solution to date* - criticism: - Ignores C already stored in the system - Only a 'one-off' fix - Assumes biomass in replanted area is maximal (ignoring fire, herbivores) - Planting up of areas in trees that shouldn't be in forest - - Trillion trees campaign - Historically the Earth had 6 trillion trees - only 3 trillion remain - Campaign, released at the World Economic Forum in Davos in 2020, is to plant one trillion of them - Claim: would absorb 200 Gt of C or 2/3 of human emissions - So far about 14 billion trees planted **Wetlands in NCS** - Peatland - Partially decomposed plant material (peat) -- formed and conserved by persistent saturation with water (oxygen free cond.) - Store 600 gigatonnes of C - This represents 44% of the world's total soil C - Peatlands -- main threats to their carbon storage - Drainage and drying (leads to O~2~ and thus decompositon) - Fires (exacerbated by drying) - Land use (agriculture and forestry) - Climate warming (promotes decompositon) - Tropical peat forests in SE asia - Very deep layers of peat (Ca. 14 m) have formed over the peat few thousand years - These peatlands are heavily forested and store lots of C and nutrients - More than half SE Asian forest soil C is in peat - 50% of SE Asia's peatland cleared in past 30 yrs, for oil palm and Acacia - Drainage of peat - 0.3 Gt C loss per year, about 1/3 of global land use emissions - Drained peatland is really flammable, which also leads to massive C losses - 1997-1998 El Nino peat fires made Indonesia third biggest emitter of greenhouse gases, after China and US - Degradation of peatland and land use  - Restoring carbon and nutrients in degraded peat forests - Blocking drainage channels and paludification - Need to restore vegetation but is extremely difficult for various reasons (perhaps they have transcended a 'tipping point') **Concerns about NCS** 1. Can infringe on the rights of 'Indigenous Peoples and Local Communities' (IPLCs) 2. Can distract or detract from other urgently needed actions such as decarbonization 3. Can be misinterpreted and misused (eg inappropriate tree planting to meet trillion tree targets). Biochar - Produced from the thermal degradation ('pyrolysis') of organic material in the absence of oxygen. Includes charcoal and is very carbon-rich. - Some have strongly advocated it as a NCS. The idea is to bury it in the soil to serve as a long term store of carbon - Very stable for long time (hundreds to thousands of years) - There has been strong advocacy for it, and belief that it is some sort of silver bullet - 1\. What are the benefits of widespread application of biochar to terrestrial ecosystems? - **Carbon Sequestration:** Biochar traps carbon dioxide in a stable form for thousands of years, reducing greenhouse gases. - **Soil Fertility:** It improves soil productivity by enhancing nutrient retention, water retention, and microbial activity. - **Renewable Energy:** Byproducts of biochar production can be used to create sustainable biofuels, reducing reliance on fossil fuels. - **Waste Utilization:** Converts agricultural and forestry residues into a valuable product instead of leaving them to decompose and release CO2. - **Absorbing polyphenols, etc.** so sepuesters toxins ( as it gets older, this capacity goes down) - Water Management: Its porous structure helps soils hold water more effectively, which can be especially valuable in arid regions. - - 2\. What are the drawbacks of doing this? - **Charcoal dust Is ghg** - **Not globally used** - **Needs specific conditions where to be used** - **Not too long term** - **Land Use Concerns:** Large-scale biochar production could lead to deforestation or competition for arable land, impacting biodiversity. - **Uncertain Effects:** Modern biochar may not mimic ancient terra preta soils in stability or fertility improvements. - **Potential Emissions:** Some studies suggest biochar may accelerate microbial breakdown of organic matter, releasing CO2. - **Distraction Risk:** It might divert focus from reducing emissions at the source, acting as a \"band-aid\" solution. - Deforestation and Biodiversity Loss: Large-scale biochar production might encourage deforestation and plantations if biomass harvesting is not managed sustainably. - Social Inequities: Widespread application might disproportionately benefit certain regions or industries while negatively impacting others, especially in developing areas. - insufficient long-term research on the impacts of biochar in different soils, climates, and ecosystems. - Biomass is already used for other purposes, such as producing biogas, which is more profitable. -  Practical Challenges in Application: Where and how to apply biochar is a logistical problem. If biochar is spread on top of the soil, it risks being blown away by wind or washed away by rain. However, burying it deeper in the soil can disrupt soil structure and is labor-intensive, potentially making large-scale deployment challenging. - 3\. Based on the balance of (1) and (2), what do you think would be the environmental consequences of the widespread application of biochar, and do you think that this is something we could or should not be doing (giving reasons)? - In some areas, biochar could significantly improve soil quality and carbon sequestration, particularly in degraded soils or water-scarce regions. - Large-scale production could lead to deforestation and ecosystem degradation, particularly if forests are cut down to produce biochar. - Not a Silver Bullet: Biochar should not be seen as a one-size-fits-all solution to climate change or soil degradation. It may be worth exploring in specific contexts, but it is not a universal remedy. - A more sustainable approach might be to use biochar to enhance existing ecosystems rather than disrupting forests or harvesting biomass unsustainably. (applying biochar in degraded agricultural areas could provide benefits without harming natural ecosystems) - 4\. Despite strong advocacy from the biochar lobby over the past 15 years, and political buy-in, widespread biochar application in ecosystems has yet to be extensively deployed as a NCS in practice. Why do you think that is? - **Uncertainty about long-term impacts** on ecosystems. - **Limited awareness** and slow adoption by policymakers and industries. - **Competing NCS solutions** (e.g., biofuels) offer faster economic returns. - **Concerns about nutrient loss** in forests from residue removal. - Viewed as **not a universal solution**, requiring case-specific applications. - **More studies needed** to determine optimal use and scalability. - Logistical Challenges: Applying biochar effectively at scale presents logistical and labor challenges. As David noted, if biochar is left on the surface, it can be lost to wind or rain, while burying it disrupts soil structure and requires significant effort. - often written in the media about biochar can be overly optimistic, While biochar has potential, best way to address climate change remains reducing fossil fuel use and transitioning to sustainable energy systems. ### Pollution as a global driver **Main types of human pollution** - Carbon dioxide enrichment - Nitrogen deposition - Acid rain - Ozone pollution - Light and noise pollution **CO2 enrichment - general** - doesn't just induce climate change - also has ecological effects - because CO~2~ is a resource for plants and increased atmospheric CO~2~ means that this resource is increased **CO2 fertilization and plant growth** - promotes plant growth - but different effects in different areas and ecosystems **Other physiological responses to CO2 fertilization (other than growth)** - Higher photosynthetic rate - Greater water use efficiency - Production of more sugars, structural carbohydrates, carbon-based defense compounds - Increased tolerance of low light levels - More roots which aid nutrient uptake - Reduced plant litter quality reduced decomposition rate - Less N - Higher polyphenols etc. (harder to decompose) - Decline in forage quality **Elevated CO2 and plant nutrients** - More CO2 but not nutrients - Leads to plants having more carbon but not more nutrients in their leaves - Means that the ratio of C to nutrients in their leaves increases and plants get less nutritious -- notably the C to N ratio increases - Less nutritious less leaf damage or greater consumption, but less performance **Effects of decline in forage quality through increased C to N ratio** - Reduction in forage quality - Reduced development rate, body size/condition - Reduced reproduction **CO2 enrichment above- vs. belowground** - Aboveground: reduction of C-storage - Belowground: increase in C-storage **CO2 enrichment and soil food web** - often positive effects because more C goes belowground **Haber-Bosch process** - major step in human alteration of global N cycle - production of ammonia (discovered in 1909; first industrial production in 1913) - Combines N~2~ gas with hydrogen using a metal catalyst under high pressure and temperature - Facilitated modern agriculture, and resultant human population increase **Human emission contribution to global N cycle (from most to less)** - Nitric oxide (NO) - Ammonia (NH3) - Nitrous oxide (N2O) **N deposition effects** - promotion of NPP - transforming plant communities - Increased plant biomass in the long runreduced diversity, through increased production - Changes from butterfly species that dislike N to butterfly species that like N - soil C sequestration - may improve litter quality and decomposition - Variable effects on litter decomposition and turnover - exacerbates P limitation **N deposition and NPP** - promotion of NPP **N deposition and plant communities** - transforming plant communities - Fast growing competitive plants dominate at the expense of slower growing stress tolerant plants - Important driver in heathlands -- heath vegetation gets replaced by grasses **N deposition and diversity** - Increased plant biomass in the long runreduced diversity, through increased production - First, increased diversity - Increasing biomass or productivity leads to dominance by competitive plant species and competitive exclusion of others **N deposition and litter quality** - Adding N reduces plant foliage and litter C to N ratio - As such, the effects are opposite to those of CO~2~ enrichment - may improve litter quality and decomposition - in reality the effects are not so simple **N deposition and litter decomposition and turnover** - Variable - Accelerates cellulose breakdown through promoting microbial cellulase activity - Retards lignin breakdown through supressing microbial ligninolytic enzyme activity **N deposition and tree litter decomposition** - Initially high N litter breaks down easier - then later residues are there with higher lignin - enzymes that break down lignin don't like N so low N litter is better at that point **N deposition and P limitation** - N exacerbates P limitation - Increased inputs of N (and C) to the ecosystem increases biological demand for P - This exacerbates P limitation in ecosystems and causes increases in ratios of C to P and N to P **N deposition and policies** - eg. In Europe , limitation of n fertilization - strong policies, and they worked **Time lag for recovery from N deposition** - N is stored and cycled in systems - Systems have sometimes long life cycles, which cause this lag **Acid rain definition** - Rain with pH of 4 to 5 (most water has a pH of \6.5) - Derives from SO~2~ and NO~x~ (= NO, NO~2~) gases, - through unclean burning of coal and oil **Acid rain and forests:** Large scale dieback in forests in Europe & eastern North America in 70s & 80s emerges from: - Acid stripping nutrients from leaves - Acid mobilizing toxic aluminium in soils - Acid dissolving waxy coating on leaves, causing leaf damage and impairing photosynthesis - Weakening trees to make them more susceptible to other stressors **Acid rain effects on trees in eastern US** - Calcium leached from needle membranes - Decreased cold tolerance - Increased freezing injury - Calcium & magnesium leached from soil - Aluminium mobilized and taken up by tree - Root function and nutrition impaired **Acid rain and lakes** - lower water pH adversely affects freshwater biota - directly - through mobilizing aluminium - Reduced calcium resulting from acidification - greatly reduces *Daphnia* (key basal trophic group in lakes) **Acid rain and policies** - Reduction of acid rain stemming from successful policy - Emissions of SO2 going significantly down **Light pollution and freshwater ecosystems** - disrupts physiological and reproductive rhythms. - Light lowers melatonin affecting biological processes. - Insects are drawn to light, increasing predation and exhaustion. - Light pollution misguides species to unsuitable egg-laying habitats. - Light disrupts migratory paths for species like bats, fish, and amphibians. - Light alters food-web dynamics and species interactions in ecosystems. - Skyglow affects zooplankton migration, impacting fish and phytoplankton. - Light reduces evolutionary potential in some species - Light pollution affects aquatic organisms like cyanobacteria and periphyton. **Light pollution and soil** - During peak plant biomass, increasing ALAN: - Reduced plant biomass - Decreased soil water content - Reduced soil respiration - Microbial communities - maintained stable biomass - Did not affect microbial community structure - Nematodes increased - Homogenization of nematode communities **Light pollution and species interactions** - Temporal Mismatches: - Temporal Niche Expansion: Diurnal species extend their activity due to ALAN, leading to increased predation as photophilic prey are exposed. - Temporal Niche Contraction: Nocturnal species reduce activity due to ALAN, disrupting natural behaviors. - Spatial Mismatches: - Photophobic nocturnal species avoid areas affected by ALAN, limiting their range and behavior. **Light pollution and ecological networks** - It alters ecological interaction networks via pathways - Primary production, species survival, movement, distribution affecting species interactions, predator prey dynamics, changes in pollination processe - Cascading effects - Impacting not just individual species but entire communities - Can disrupt food webs, ecological functions, with potential decline in biodiversity **What are the main research gaps needing to be filled to better understand how light pollution affects ecosystems** - interactions - species and systems - cascading effects - multi-stressors (ALAN and other stressors effecting systems together) - long term changes - Possible adaptation - **Do you think light pollution is a big environmental problem?** - cascading effects - maybe not the biggest problem, but in cascading and addition: Bigger than thought - multi stressor **Mitigation of light pollution** - Maintaining and increasing natural unlit areas is likely to be the most effective option - conflict with other social and economic objectives - Decrease duration of lighting - shift direction of light - Use red or at least not very white light color - Decrease intensity ### Biodiversity loss and ecosystem functioning **Diversity** - Richness: how many types of things - Evenness: Even composition of things - AAAAAAAAAAAAAABCD - AAAABBBBCCCCDDDD higher diversity index - Used by Shannon index, Simpsons index **Scales of Diversity** - Alpha diversity: how many species are in the community - Beta diversity: how many compositional differences between communities - Gamma diversity: total overall diversity in the system **Diversity types** - Within species (genetic) - Species - Higher levels (families, orders, etc) - Groups (= functional groups) - Trees, Shrubs, Mosses, Grasses, Forbs - Functional diversity - Example: - Grassland: High species diversity - Forest: High structural diversity **Why should we care about diversity?** - Ethical reasons -- nice not to have things become endangered or go extinct - Necessary for functioning of the Earth's ecosystems - Delivery of ecosystem services upon which we all depend (food, forage, fibre, C sequestration, water quality, etc). **Insect diversity development** - Global decline of insects - Many vulnerable, endangered or extinct - Loss of pollinators - Butterfliy risk state dependend on region **What is the most important current driver of biodiversity loss globally?** - Human land use change and habitat loss - In arctic: biggest driver = climate change - Biggest factor in future - Climate change (and invasive species) - Land use already so much change that now other factor are major **Biodiversity loss and ecosystem functioning , many studies have used...** - Local scale biodiversity manipulation experiments to addreass this **Biodiversity and ecosystem function hypothesis**  - Redundant- small portion of species can do the job **Random assembly experiments** - Species pool is defined - Different numbers of species are drawn from the pool at random (1, 2, 4, 8 etc...) - Artificial communities are set up - Ecosystem functions (e.g., production, decomposition, nutrient fluses) are then measured - Assumption: *Species are lost at random, or if they are not lost at random then their traits that drive ecosystem functioning are independent of those that determine susceptibility to extinction.* - **Ecosystem function and biological diversity** - Ecosystem function: resource capture, biomass production, decomposition, nutrient recycling - Biological diversity: variation in genes, species, functional traits - In general : higher function with higher diversity - but: high productive monoculture is mostly still higher in biomass than mixture - Conclusion: in general diversity = higher function, but some monocultures have higher function **Why is biomass greater with more plant species?** - Plants that coexist partition resources(resource partitioning) - Plants that coexist help each other(facilitation) **Resource partitioning** - Use different resources, use resources differently, get resources from different places, etc. - Enhances total biomass **Facilitation** - Plant species helping each other also promotes total biomass 1. **Indirect Biotic Facilitation**:\ Species **a** and **b** complement each other, increasing spatial and resource use efficiency by combining different structures (horizontal and vertical growth). 2. **Abiotic Facilitation---Nutrient Enrichment**:\ Species **a** enhances nutrient availability (through root processes), benefiting both species in the mixture. 3. **Abiotic Facilitation---Amelioration**:\ Both species contribute to reducing environmental stress and improving microclimate (e.g., shading or microclimate improvement), creating a more favorable environment for growth. **Trophic scale interactions** - Loss of plant diversity leads to loss of consumer diversity - Loss of diversity by loss of hosts **Ecosystem services** - Underpinned by Ecosystem functions - we depend on them (e.g., production of food and fibre, crop pollination, C storage, water purification etc. **Diversity and environmental harshness** - Diversity changes with increasing env harshness dependend on starting point  **Effects of Biodiversity on Ecosystem Services: Evidence from Swedish Forests** all services **Biodiversity loss and ecosystem functioning scale problems** - Many studies used local scale biodiversity manipulation experiments to understand how biodiversity loss at global scales affects ecosystem functioning - assumes that global diversity loss is reflected at local scales - Many studies show increased and reduced diversity over time on local scales - Invasive species - No local decrease- studies done on wrong scale **Changes in the different scales of diversity** - Community alpha-diversity shows variable changes over time, with strong declines only with intense land use - Communities are becoming more homogenized (beta-diversity declining) - Climate, invasion, etc **Native-nonnative- diversity development** - native species dying - this is conquered with even more increasing nonnative species **Land use dependence (small local scale)** - Grassland, agriculture: increase diversity - City: net loss **Species loss effects** - greatest when the species lost from the ecosystem has a unique role that other species cannot compensate for - Key examples involve loss of apex predators, top carvivores, large herbivores (and big things generally) - Examples: - Big trees: - Have most carbon - Drive ecosystem functionunb - Drive c seq - More likely to be removed (money, material) - Plants that are first lost by N-deposition are those that produce poor litter which promotes OM build-up - Trophic downgrading of planet earth **Discussion: How does biodiversity loss affect ecosystem functioning and ecosystem services?** 1. **Forest stays intact and doesn't lose any species** - Balance - No change - Diverse communities are more productive because they contain key species that have a large influence on productivity, and differences in functional traits among organisms increase total resource capture. - Berry and game production - 2. **Forest loses all but one tree species, but that one tree species is the most productive one (turning into pine monoculture)** - **Functions:** - **High productivity, might turn into less over time** - **Higher nutrient cycling, might turn into less over time** - **Lower resilience, high vulnerability** - **Services:** - **Less food provision** - **First higher wood then less wood production** - **Less c sequestration** - Cant support diverse other trophic - Lost resources for herbivores and therefore also for predators - Declining herbivore and predator populations - Lost soil fertilization by manure, nutrient cycles - Lost disturbance - More c above ground but a lot les below 3. **Forest loses all the predators** - **Functions:** - **Less productivity** - **More or less nutrient cycling (dependent on circumstances and looked at part)** - **Less resilience** - **Services:** - **Plus for meat and minus for berry production** - **Less wood profvision** - **Less c sequestration** - Loss of predators increases vegetation, fueling wildfires - Predator declines raise prey populations, spreading diseases - enables invasive species dominance (e.g., brown tree snake on Guam). - reduces diversity, altering forests and landscapes. - 4. **Forest loses all the herbivores ( as well as the predators)** - **Functions:** - **Higher productivity, later less** - **More or less nutrient cycling (dependent on circumstances and looked at part)** - **+/0/- in resilience (dependent on circumstances and looked at part)** - **Services:** - **Minus in meat- and plus in berry-production** - **First, more wood production, then less with time** - **Less c-sequestration** - - Increase in vegetation that is usually eaten by herbivores Biological invasions -------------------- **Non- native, introduced, alien species** - Species that are introduced to new environment - Most of these don't do much and remain subordinate - small portion (ca. 1%) have the capacity to spread and have significant ecological impacts; these are '[invasive]' species **Invasion steps** - Introduction (it has to get there) - Dispersal (it has to spread beyond the point of introduction) - Establishment (once it has spread beyond the point of introduction it needs to form new populations) - Impact (these new populations need to cause some influence on native species and/or ecological processes **Accidental invasion** - transport, trade - removal of natural barriers - escape of domesticated animals, plants (including ornamentals) - ballast water - introduction of hosts - examples: - melting of arctic ice and expansionon the northern sea routes facilitates invasion of arctic - Spread of non-natives facilitated by 2011 Tohuku tsunami and floating garbage **Deliberate invasion** - species for hunting/fishing - species for restoration or biological control - species for cultural reasons - illegal transport of organisms - examples: - trout for fishing, problem because predator **Establishment** **(1) *Abiotic factors* \-- theory of fluctuating resource uptake** - When a big supply of resource is possible but not taken up (eg. Early succession) invasions play a big role **(2) *Biotic factors* -- biotic resistance** - Paradox: (depending on study/environment/etc.) - With higher number of native species, there is higher number of exotic species as well, because of heterogeneity and more diverse niches that could be invaded - With higher number of native species, there are less invasions because there is better use of resources, therefore higher biomass and therefore higher resistance to invasions **Islands/Mainland -- Invasions** - More invasions on islands, because they have less native diversity **Global vs. local diversity development** - Global : decrease - Local: - increase, because the addition of invaders cancels out loss of native species - Species loss and biological invasions occur concomitantly **When do non-native species exert impacts?/ What do invasive species need to have?** - Traits different from native species - Meaning they could have a potential foreign effect in the new environment - Traits have to do something (not some random useless function, etc.) - Species have to be abundant **No large consistent (unidirectional) effects could arise either because:** - (Invaders are unimportant, or) - More This:Effects of invaders are context-dependent (large positive and large negative effects both common) **Traits of native versus non-native plant species** - Higher SLA - Higher N% - Higher maximum photosynthetic rate **Examples of invasive species effects on soil processes** - positive: N-input through n-fixation - negative: Diverse dryland invaded by singular tree species, less diversity, Undecomposed thick layer of litter, Complete ecosystem change **Invasive plants and fire regimes** - invasive grasses in Hawaii like buffelgrass are highly flammable - leading to extensive, more frequent fires **Invasive consumers** - Effects occur through \(1) invader removing other organisms that are ecosystem drivers, and \(2) non-consumptive effects - Ecosystem consequences studied less than for invasive plants - but examples exist for all major trophic groups (e.g., herbivores, predators, pathogens, decomposers) **Invasive browsing mammals in New Zealand** - Didn't have large herbivores - Only mammal they had was bats, before European colonization - Problems with Possums, Goats, Red deer (set there for hunting) **Invasive ants on Christmas Island** - Red Land Crabs - main macroinvertebrate herbivore and detritivore - Invasive 'Yellow Crazy Ants' eliminate land crabs - Wide ranging aboveground and belowground effects, including greater seedling density, reduced decomposition **Earthworm (*Lumbricus terrestris*) invasion** - Released left over worms after fishing - Soil turned over, homogenization, less ground vegetation **Invasive predatory flatworm** - Earthworm-eating flatworms - Adverse effects on soil porosity and drainage, leads to waterlogging, increase in *Juncus*, reduction of burrowing moles **Invasive beavers in Tierra del Fuego & their carbon effects** - Intentionally introduced to provide a fur industry - Substantial felling of *Nothofagus* forest - Has the largest impact on these forests since the last ice age - Aboveground carbon effects - Less living aboveground C - More standing dead C - More coarse woody debris C - Less total carbon C **Largest animal to have become invasive** - Hippos in Columbia - After Zoo closed down, they were just let out - Invasive hippo effects on waterways: - More transport of OM from land to water - Greater ecosystem metabolism - More cyanobacterial blooms - Problems expected to become more severe - Might become more aggressive as populations increase, risk for humans **Invasional meltdown** - All invading, help each other invade - co-invasion of pine trees, ectoycorrhizal fungi and mammals - Faster more widespread and easy invasion **Rats and seabirds** - Invasion through colonization, ships - Rats eating eggs, fewer sea birds - Reduction of seabirds by rats stops nutrient inputs to surrounding sea - Leads to food limitation, and ultimately reef fish (e.g., damselfish) growing smaller, having larger territories and displaying less aggression - Less total C, total N - Changes n isotope due to marine terrestrial n - Plant nutrient effects - Less total dry weight - Less foliar P **Invasive consumers** - Many iconic examples are now recognized - often point to substantial transformation of ecosystems - Probably stronger effects than invasive plants - Need to move from documenting a series of impressive examples to developing general principles of how, when and why invasive consumers transform ecosystems **Economic cost of invasive species - sources** - Loss of crop yields - Loss of timber production - Damage to infrastructure - Spreading of disease - Promotion of fire - Most cost comes from invasive invertebrates - Often influencing crops, agriculture **Economic cost of invasive species -- development** - Damage costs exceed management costs since around 1990 **Management of non-native species** - Best in early stages (lower cost for higher effectivity) - Focus on prevention is good Ein Bild, das Text, Screenshot, Schrift, Reihe enthält. Automatisch generierte Beschreibung **Criticisms of invasion biology as a discipline** - Impacts of invaders are not that bad and are exaggerated - Increases in non-native species increase biodiversity, and this increased diversity is a \*good\* thing - Positive (desirable) attributes of non-native species are often underplayed and are frequently important - The biogeographic origin of a species has no bearing on its ecological impact **Impacts of invasive species are generally not that bad and are often exaggerated** - Depends on - Species invaded and invasive species - Type and status of invaded environment - What state we want to protect - There are some really bad examples - So, not generally exaggerated/not that bad - invasion depth (principle like extinction depth)-- issues today might favor invasions in the future on large scale - some very concerning species, some don't really matter - But of course, we will see a gradual change in species due to climate change ("normal") **Efforts based on managing invasive organisms and mitigating their effects is often misplaced and not a good use of scarce resources** - If money is used for it, there is probably a reasonable reason due to damages (e.g. economic) - For non harming species: probably not looked at/ no expensive mitigation used - Precautionary acts: Costs would be higher if waited longer - Putting money into conservation efforts "always a good idea" **Presentations: Effects of invasive species** **Presentation - Human health** - Categories: - causing diseases or infections - pathogens/paraasites - vectors/reservoires of pathogens/parasites - favor pathogens/parasites and/or their vectors/reservoires - exposing humans to wounds from bites/stings, biotoxins, allergens or toxicants - facilitating diseases, injuries or death - inflicting other negative effects on human livelihood. - - Problems: - Mosquitos and ticks with pathogens introduced mainly by human movement - Increasing interest in exotic animals - - Mitigation: might be also negative in a different sense, e.g. use of pesticides, impact on human health - Examples - European smallpox measles spread by Spanish in 16^th^ century - HIV that spread from primates in Africa 100s of years ago - David: diseases are invasive organisms **Presentation - Ecological impacts of invasive plants** - Significant effect on a lot of ecological variables - Bottom up effects on higher trophic levels, through changes in plant availability - Similar effects on island and mainland ecosystems (bu still more species invasions on islands) - Invasive plants have higher effect on community compositions than on N-cycling - Bad paper/meta-analysis concept here wrong: - Effects cancel each other out - One plant as xy positive effect other xy negative effect: their result: no effect visible - Very depending on what is published **Presentation - Ecosystem services** - Ecological - Biodiversity loss - Alteration of ecosystem structure - Disruption of food webs - Changes in nutrient cycling - Economic - Agricultural damage - Fisheries decline - Increased management costs - Social and Health Impacts - introduce new diseases - enhance existing health issues within a community - livelihood effected -- stress? - cultural, aesthetic, recreation effected Practice Exam ------------- Regular (G) questions:\ 1. What are 'ecosystem services' and why are they relevant in a global change context?\ Services provided by ecosystems upon which humanity depends - Ecosystem services (e.g., food and fiber production, crop pollination, carbon storage, water purification) are vital for human well-being. - These services rely on underlying ecosystem functions. - Global change---climate change, biodiversity loss, and pollution---threatens these services, impacting food security, water availability, climate stability, and human well-being. - Degraded ecosystems struggle to maintain their functionality, compromising their ability to deliver these critical services. 2\. The IPCC has concluded that the global mean air temperature has been rising and will likely continue to rise during the remainder of this century. List two examples of how other ongoing changes in climate (meaning not an increase in mean air temperature) may impact the earth system. 1. **Sea Level Rise (Fastest in 3,000 years)**: - **Impact**: Flooding of coastal areas, displacement of communities, and saltwater intrusion threaten habitats and freshwater resources. - **Example**: Small island nations face land loss and freshwater scarcity. 2. **Arctic Sea Ice Loss (Lowest in 1,000 years)**: - **Impact**: Reduced ice decreases Earth\'s albedo, accelerating global warming and disrupting Arctic ecosystems. - **Example**: Polar bear populations decline due to loss of hunting grounds. 3. **Glacier Retreat (Fastest in 2,000 years)**: - **Impact**: Loss of freshwater supplies, rising sea levels, and destabilization of mountain ecosystems. - **Example**: Himalayan glacier retreat threatens water availability for rivers like the Ganges. 4. **Increased Frequency and Intensity of Extreme Weather Events**: - **Impact**: Intense hurricanes, heatwaves, and wildfires disrupt ecosystems, damage infrastructure, and release carbon. - **Example**: Wildfires in boreal forests release stored carbon, amplifying climate change. 5. **Ocean Acidification**: - **Impact**: Increased CO₂ absorption disrupts marine food webs and harms calcifying organisms like corals and shellfish. - **Example**: Coral reef degradation threatens biodiversity and fisheries. 3\. Why does coral bleaching occur, and why is this a problem?\ **Coral bleaching** - In response to environmental stress - Phenomenon where corals lose their symbionts - bleached corals more vulnerable to starvation and disease. -  **Loss of Vital Nutrients**: Without the algae, corals lose their main food source, making it difficult for them to survive. -  **Ecosystem Disruption**: Coral reefs support marine biodiversity, providing habitat and food for numerous species. Coral bleaching weakens these ecosystems, threatening the survival of many marine species. -  **Economic Impact**: Coral reefs support fishing and tourism industries. Their degradation can harm local economies dependent on healthy reefs for livelihoods. 4\. Describe briefly how wildfire affects ecosystem carbon sequestration. - **Short-term impacts**: - Wildfires release large amounts of carbon stored in vegetation, soil, and organic matter as carbon dioxide. - This immediate release increases greenhouse gas emissions and disrupts the natural carbon balance of ecosystems. - **Long-term impacts**: - Wildfires can reduce ecosystems\' carbon sequestration capacity. - Regrowth of vegetation may absorb some carbon, but frequent or intense fires can hinder recovery. - Fire-affected soils may lose their ability to retain carbon, further decreasing carbon storage. 5\. Are the world's permafrost regions today most likely a source or a sink for carbon? (Motivate your\ answer in maximum four sentences) **Permafrost as a carbon sink in the past:** - Permafrost regions stored large amounts of organic carbon accumulated over thousands of years. - Cold temperatures slowed the decomposition of plant and animal matter, preventing carbon release. - The freezing conditions preserved organic material, trapping carbon in the soil. - Permafrost acted as a long-term reservoir of carbon, helping regulate atmospheric CO2 levels. **Permafrost as a carbon source today:** - Rising global temperatures are causing permafrost to thaw, releasing stored carbon as methane and carbon dioxide. - The thawing process accelerates the release of greenhouse gases into the atmosphere. - This release of carbon exacerbates climate change, creating a positive feedback loop. - The thawing of permafrost is currently outpacing its ability to sequester carbon. 6\. Why are short-term melt events important for future ice sheet mass balances? 7\. Describe and explain the impact of ocean acidification on three groups of marine organisms 8\. Name one way in which human activities substantially increase vapor flows from land surfaces to the atmosphere. 9\. What is biochar and what potential do you think it has in mitigating climate change? 10\. What is the 'Haber-Bosch' process and what is its relevance to global change? 11\. Describe the difference between alpha-diversity and beta-diversity. 12\. Invasive species can be introduced to new environments by both accidental means and deliberate means. Give two examples of each. VG questions 13\. How do the direct effects of elevated atmospheric CO2 cascade through ecosystems and impact their functioning? 14\. Describe how mass balance calculations are used to understand the impacts of climate change on either the global water cycle, permafrost carbon, or ice sheets. Which of the reservoirs and fluxes are most challenging to estimate? 15\. Discuss the changes that are occurring in plant species richness at local spatial scales as a result of simultaneous local extinction of native species and entry of non-native species.