Smokin Notes Human Evolution and the Origin of Life Study Guide in word.docx
Document Details
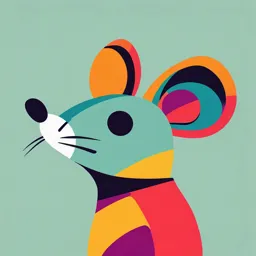
Uploaded by AppreciatedTurkey
Full Transcript
Macroevolution & The Origin of Life Humans and the Tree of Life Human beings, Homo sapiens, are a relatively new "branch" on the tree of life. We belong to the "chordate" branch, which is most closely related to echinoderms (sand dollars, sea urchins, and sea stars). Scientists are continuing to...
Macroevolution & The Origin of Life Humans and the Tree of Life Human beings, Homo sapiens, are a relatively new "branch" on the tree of life. We belong to the "chordate" branch, which is most closely related to echinoderms (sand dollars, sea urchins, and sea stars). Scientists are continuing to shape and reshape the tree of life as we learn more about evolutionary relationships between organisms, including humans. If we follow this branch, we can see how human evolution occurred and which evolutionary steps came first. • First, the craniates (animals with skulls) diverged. • Then, the vertebrates (animals with backbones or spinal columns) diverged. • Then, jawed vertebrates (vertebrates with jaws) diverged. • Then, tetrapods (animals with four legs) diverged. • Then, amniotes (animals with a terrestrially adapted egg) diverged. The group of amniotes includes reptiles, birds, and mammals. Two groups of early ancestors that came before craniates are the cephalochordates and the urochordates (including lancelets and sea squirts). These are small, fishlike marine invertebrates that have a hollow, dorsal nerve cord that was the precursor to our backbones and nervous system. Like all chordates, they have a notochord, nerve cord, gill slits, and a postanal tail at some stage in their lives. (Urochordates have all of these in their juvenile state but lose them in their adult form.) Cephalochordates appeared in the fossil record for the first time about 500 million years ago, about the same time as the Cambrian Explosion. Mammals, which arose about 175 million years ago, are characterized by mammary glands, sweat glands, hair or fur, three middle ear bones, specialized teeth, a neocortex brain region and four-chambered hearts. (Note that animals other than mammals-such as birds and alligators-have four -chambered hearts as well .) Mammals generally use internal fertilization for reproduction and generally give birth to live young. They ore worm-blooded thermoregulators, meaning they control their body temperatures. Evolution of Primates Evidence shows that there was a huge adaptive radiation of primates beginning approximately 65 million years ago, after the mass extinction during the Cretaceous Period (when the dinosaurs went extinct). During this period, a number of new primate species emerged; one of these species gave rise to modern humans. Scientists believe that primates' most ancient ancestor, which lived about 65 million years ago, was an arboreal insectivore, that is, it lived in trees and ate insects. Dr. Gillooly presented a picture of what one of these animals might have looked like. The skeletal reconstruction of these animals gives us insight into how humans evolved. For example, vertical climbing required these animals to orient their bodies in a way similar to modern bipedal walking. The Evolution of Homo sapiens Humans are members of the species Homo sapiens. DNA evidence indicates that humans originated in East Africa approximately 200,000 years ago. Below, we will discuss the evolution of primates in general and then briefly discuss the evolution of Homo sapiens. The Impact of Selective Forces The evolution of primates occurred due to the impact of selective forces on primate phenotypes. It is believed that, approximately 23 million years ago, a change in climate turned the African continent from a warm, wet forest to a cool, dry grassland during the Miocene Epoch (23 MYA to 5 MYA). As a result, the arboreal animals that lived in African forests had to adapt to the changing conditions. Without these climatic changes, the human species would have probably never evolved. History of Human Evolution A common misconception is that Homo sapiens evolved from chimps. Our direct ancestors were not chimps or any other modern apes. The evolution of primates has not been linear; that is, we have not gradually evolved from our earliest ancestor until now. Rather, there has been a mini-adaptive radiation of primates over the past five million years. As a result, a number of primate species have evolved. Some of them- including Australopithecus boisei and Australopithecus robustus were evolutionary dead ends; these species simply died off. Moreover, different species of humans coexisted with one another at different points in time. Scientists are continuously making important discoveries that inform our understanding of human evolution. Two important discoveries were as follows: • Ardi - In 1994, scientists discovered the remains of Ardipithecus ramidus in Ethiopia. The individual they found has been affectionately named "Ardi." He was older than Lucy, dating back 4.5 million years. He clearly walked upright and had long arms and weird feet. He walked on two legs but could still climb trees, and he was physically suited for an arboreal lifestyle. He was only about 4 feet tall and about 85 pounds. • Lucy - In the 1970s, scientists discovered the remains of Australopithecus afarensis in North Africa. The individual they found has been affectionately named "Lucy." She lived about 3.2 million years ago. Lucy had hands and feet like ours and walked upright, but she was only three or four feet tall. (Males of this species were about 75 pounds, and females were about 60 pounds.) Approximately 150 other individuals of this species have been found since Lucy. The Evolution of Homo Sapiens Homo erectus was a primate that evolved in Africa about 2 million years ago and survived until about 53,000 years ago. (No "human" fossils this old have been found anywhere except Africa.) It was taller than a more distantly related species called Homo habilis, which existed 2.3 to 1.3 million years ago, and it was a creative toolmaker and perhaps the first fully monogamous species closely related to humans. This species began to use fire, and it made more sophisticated use of tools than did Homo habilis. Homo sapiens (modern man) emerged approximately 200,000 years ago, and Homo habilis eventually died out. Homo sapiens were hunter-gatherers until 10,000 years ago, with the advent of farming. The most "modern" forms of man emerged between 2,000 and 3,000 years ago. Dr. Gillooly pointed out that this was relatively recent, in terms of evolutionary time. Homo sapiens had a number of important characteristics that distinguished it from its more primitive cousin, Homo erectus: Its teeth and jaws were smaller. Its facial bones were smaller. Its skull was able to hold a larger brain and could engage in symbolic thought. The overwhelming evidence is that humans arose in Africa, as the oldest human fossils were found there. Over time, humans migrated north and then both east and west. They eventually made it to all of the parts of the Earth. The impact of different environments imposed important selective pressures, which furthered the phenotypic differences among us. (In this case, microevolution was driven by the founder effect, as human explorers migrated to new habitats.) Interestingly, a UF professor is currently researching the migration of humans through North and South America by studying the evolution of lice in native cultures, and applying molecular clock concepts to them. (When he recently went to Ecuador, Dr. Gillooly was nice enough to bring some lice samples back for his colleagues!) Dr. Gillooly pointed out that human migration to some parts of the world is very recent. The Evolution of the Human Phenotype In this section, we will discuss the evolution of the human phenotype, including structural and behavioral changes that have occurred in relatively recent evolutionary history. We will focus on changes in dentition, brain size, and bipedal movement. We will then see how some of these phenotypic changes impact and are impacted by changes in family structure and cultural evolution. Changes in Dentition and Mouth Structures I Compared to our primate ancestors, humans have a rounder mouth with reduced molars and thicker, more mineralized teeth. Our jaw muscles are much smaller than our primate ancestors, reflecting the smaller size of our jaws and dental structures. Changes in Brain Size Humans have larger brains than chimpanzees, Australopithecus africanus, Homo habilis, and Homo erectus and the brain size of hominids has increased by more than threefold in species that arose over the course of the past several million years. Note that Neanderthals actually had slightly larger brains than humans. Dr. Gillooly highlighted the fact that brain size growth has been exponential in hominids. The increase in brain size corresponds with an increase in social behavior, the use of language, and the development of tools. Brain tissue is energetically expensive to maintain, so the significant share of energy devoted to the brain has to be justified by the additional benefits that a larger brain affords. Changes Relating to Bipedal Movement The evolution of bipedal movement provided a number of important benefits to early hominids, including efficient locomotion, predator avoidance, food gathering, freeing the hands, tracking migrating herds, and provisioning of offspring. One theory holds that bipedal movement freed up energy that could be used for brain function. In class, Dr. Gillooly showed a picture of the Laetoli footprints, preserved footprints made by an early hominid-most likely Australopithecus afarensis-about 3.7 million years ago. These footprints were made in volcanic ash and were thus well-preserved. They have provided scientists with valuable information about how these early humans walked, among other important pieces of information. Cultural Evolution It is interesting to correlate the evolution of the human phenotype with cultural evolution. In this lecture, we have seen how fire, tools, agriculture, dietary changes, sociality, and monogamy have impacted the human phenotype. Interestingly, our ability to extract and use energy may also have evolutionary consequences. Dr. Gillooly's colleague has done research on the relationship between energy use and annual fertility rates for females. She has found that fertility rates tend to be lower for organisms that use more energy. The interesting thing is that this relationship also holds true for humans: societies that use more energy tend to have lower annual fertility rates. The results of this research have led some to ask whether cultural evolution and the evolution of society are a direct result of biological evolution. Key Dates to Remember These are the key dates from this lecture that you should remember for the exam: • Mammals arose about 175 million years ago. • Adaptive radiation of mammals occurred about 65 million years ago. • Great apes (hominoids) arose about 25 million years ago. • Hominids arose about 5 million years ago. • Homo sapiens arose about 200,000 years ago. How Do We Define Life? You have now gone through a semester of introductory biology. Given this background, you might assume that you should be able to define what life is. In reality, however, even well-known, highly respected scientists have difficulty coming up with an all-encompassing definition. You could ask five of the most distinguished evolutionary biologists in the world the simple question, "What is life?" and you would get five slightly different answers. Dr. Gillooly asked the students in class to define life. They could not come up with a definition; rather, they listed a number of features of life. The general features of life include the following: • Homeostasis - Living things have an internal environment that is different from the outside world. They must maintain this internal environment at some constant, regular level. • Organization - Living systems use information to organize themselves. • Metabolism - Living things require energy for survival, growth, and reproduction. All biological processes require energy, so metabolism is essential for life. • Adaptation - Living things are under natural selection; they become better adapted to the environment over time. Even living things that evolve slowly, such as the horseshoe crabs that we discussed earlier in the semester, evolve. • Response to stimuli - Living things take in sensory input and respond to it, both on a micro and a macro level. • Reproduction - Living things self-replicate. This is a key feature that separates living things from inanimate objects. Together, these features of life characterize life, but they don't define life. Scientists debate as to whether life is possible without one of these characteristics. For example, is a virus, which does not have metabolism, alive? Scientists are split on this issue. The best definition of life that Dr. Gillooly has been able to find is as follows : "Life is a dynamic system composed of elements, that self-organizes, responds to the environment, and replicates." Three Requirements for Life All of the characteristics of life listed above share three commonalities: they require energy, materials, and information. • Energy - All organisms require a constant flow of energy for survival, growth, and reproduction. • Materials - Living things are constructed with chemical elements. Survival, growth, and reproduction require a supply of materials to act as the building blocks of biomass. Our bodies require a lot of materials, even just for the purpose of maintenance. Interestingly, the ratio of major elements (such as nitrogen and phosphorus) is roughly the same in all living things. • Information - Living things use information to respond to stimuli, organize themselves, and replicate. This includes the information in genes, but it also includes the sensory information that we process every day. We can't do anything without information, and cells can't either. Note that information is just as important a requirement for life as energy or materials, but we are only beginning to understand this important component. Whereas energy and materials are easily quantifiable, information is not. Scientists studying information theory have tried to quantify information, such as by converting humans' ability to convey information into a bit transfer rote (just like a computer). When and How Did Life Originate? The following diagram illustrates the current thought as to the events that led up to life on Earth, along with their corresponding time frames: Life began sometime between 3.5 billion years ago and 3.9 billion years ago. Asteroids struck the earth 3.9 billion years ago. Carbon fixation was 3.7 billion years ago The earliest fossils come from 3.5 billion years ago. Evidence shows that Earth was formed approximately 4.5 billion years ago, as the materials in a cloud of dust resulting from the Sun's formation came together and formed a planet. There was likely no life on Earth for at least half a billion years. Approximately 3.9 billion years ago, the Earth suffered severe asteroid strikes that boiled Earth 's water and would have killed all life if it had existed. Life on Earth began between 3.5 billion years ago and 3.9 billion years ago (though for the purposes of the exam you can simply remember that life definitely emerged by 3.5 billion years ago). Radioisotope data indicates that carbon fixation began 3.7 billion years ago, though this calculation is imprecise. The oldest known fossils date back to around 3.5 billion years ago. Early Theories about the Emergence of l.ife As recently as a few hundred years ago, humans held archaic notions about the origins of life. The dominant theory from the time of the ancient Greeks until the 1800s was spontaneous generation (abiogenesis)-that life simply sprung up from inanimate matter. Aristotle, who developed the theory of spontaneous generation, observed that mice emerged from dirty hay, aphids from morning dew, and crocodiles from rotting logs. As silly as it sounds, scientists clung to Aristotle's theory for thousands of years. In 1665, for example, Robert Hooke-the "father of microscopy"-claimed that evidence from microorganisms supported the theory of spontaneous generation. In the 1800s, a number of experiments discredited the theory of spontaneous generation. Francesco Redi, for example, performed experiments that demonstrated the link between the emergence of maggots and the presence of adult flies. He observed that maggots appeared on raw meat when exposed to populations of adult flies. However, maggots did not appear when flies were denied access to meat because the meat was covered. Since the 1800s, the prevailing theory as to how life came about has been biogenesis-the biochemical process by which the earliest organisms were created. Charles Darwin and J.B.S. Haldane described the conditions of the early earth as a "primordial soup" in which the ingredients for complex organic compounds were present. According to these scientists, something happened to turn these compounds into life. When they introduced their ideas, they were ridiculed by the scientific community. Since then, their ideas have largely been validated. The current theory is that biogenesis required four steps: 1. A biotic synthesis of organic molecules from inorganic molecules - Somehow, the inorganic molecules present in Earth's early atmosphere had to be converted into organic molecules that could act as the building blocks of life. When life emerged 3.5 billion years ago, the Earth was very different than it is today. There was little oxygen, and the atmosphere was highly reducing. The lack of oxygen meant that there was no ozone layer, so UV light could penetrate the atmosphere freely. There were, however, the basic elements of life: carbon and hydrogen. In 1953, Miller and Urey performed experiments in which they attempted to replicate the conditions of early Earth to see whether organic raw materials could be formed from inorganic substances. They circulated water through an apparatus that contained the inorganic elements of life. By applying a spark (to replicate lightning), Miller and Urey were able to turn inorganic substances into simple amino acids and other organic raw materials. Miller and Urey's experiment showed that it was possible to achieve abiotic synthesis of organic compounds. In fact, the scientists were not just able to create one amino acid; they created 22 of them! Although evidence suggests that Miller and Urey's experiment was flawed because their "simulated" environment was different from Earth's early environment, later experiments confirmed that organic compounds can result from abiotic synthesis. 2. The creation of macromolecules from small organic molecules - Once organic compounds formed, they must have been able to "spontaneously" polymerize in an abiotic environment. Scientists have been able to achieve this result in a laboratory. They drip solutions with organic monomers onto "hot rocks," and the heat causes a dehydration reaction that vaporizes the water and turns the aggregations of monomers into long polymers. 3. Packaging of macromolecules in membranes - Once organic polymers formed, they must have been able to abiotically cluster together. In this way, they create their own "mini-environment" that is separate from the surrounding environment. Scientists have been able to achieve clustering in an abiotic environment, as protobionts, the much-smaller precursors to prokaryotic cells, spontaneously cluster in such a way that their internal environment is different from their external environment. This is probably how the earliest membranes formed. Because they maintain an internal environment that differs from their external environment, protobionts exhibit basic metabolism. 4. The origin of self-replication - Once clusters of complex organic polymers form, they must be able to replicate to be considered "living." Of the four requirements for life, self-replication is the only one scientists have been unable to create in the laboratory. Scientists are still not sure how the mechanism for replication come about. Some believe that extremely rare conditions prevailed, giving rise to the ability to self replicate. Many of the mechanisms that underlie the ability to self-replicate are still unknown. Development of Life After Formation The earliest life-forms on Earth were prokaryotes, which lacked membrane-bound organelles. The term "prokaryotes" refers to a grouping of Bacteria (also known as Eubacteria) and Archaea. There are significant differences between Archaea and Bacteria, and humans are more closely related to Archaea than to Bacteria. The earliest life forms had to have some sort of genetic material to pass on to future generations. Scientists have concluded that the earliest genetic material was RNA, not DNA, for the following reasons: RNA performs more functions than DNA. For example, ribozymes are RNA molecules that act as catalysts. They can make copies of their own sequence. NADH and FADH2-key molecules in respiration-are modified ribonucleotides. DNA itself is a product of an RNA precursor. Scientists used to believe that RNA played a limited role in the cell. Today, genome analyses point towards RNA "genes" as being more important than we previously thought. RNA is capable of doing many of the things DNA currently does, indicating that it may have had a more important role in early organisms. As we have mentioned, Earth's atmosphere had very low levels of oxygen when life first emerged. However, when photosynthesis evolved, living organisms began emitting oxygen-a by-product of the process-into the atmosphere. This changed Earth's environment drastically and created conditions necessary for aerobic respiration to evolve approximately 2.2 billion years ago. The following diagram lists the major events you should be familiar with for the exam: We will discuss each of these major events in the following pages. The Importance of Atmospheric Oxygen Prokaryotes were the Earth's first living organisms, and they were the only organisms on Earth from about 3.5 billion years ago to about 2.1 billion years ago. Dr. Gillooly mentioned that we still have organisms that look like those early prokaryotes. Cyanobacteria in eutrophied lakes, for example, resemble early prokaryotes. These organisms played an important role in preparing the Earth for eukaryotes. Early prokaryotes developed the ability to use the prodigious amounts of ultraviolet light in Earth's early atmosphere to fix carbon via photosynthesis. At the time, organisms were likely anaerobes, meaning they did not use aerobic respiration. This is because oxygen levels in Earth's early atmosphere were very low. However, over time, photosynthetic organisms increased oxygen levels in the atmosphere, and aerobic respiration evolved. Most of the oxygen in today's atmosphere comes from these bacteria; in this sense, we wouldn't be here if early bacteria had not existed. As oxygen increased in the atmosphere approximately 2.7 billion years ago and aerobic respiration took hold, a number of new possibilities-and challenges-emerged for life on Earth. Recall that eukaryotes are different from prokaryotes in two major respects: • They have a nucleus. • They have membrane-bound organelles. We have fossils of eukaryotes that date back 2.1 billion years; it is at this time that scientists believe the earliest eukaryotes, which were unicellular, emerged. The earliest hypothesis about how eukaryotic cells came to be was simple: it stated that the cell membrane folded in to form specialized organelles, such as the endoplasmic reticulum and the nucleus. This early hypothesis has been replaced with another view, called the endosymbiosis hypothesis, which says that eukaryotic cells emerged as one prokaryotic cell engulfed another and, instead of digesting it, lived with it in a symbiotic relationship. According to this theory, plastids (such as chloroplasts) and mitochondria are the evolutionary remnants of early prokaryotic endosymbionts, which are organisms living inside other organisms. The following graphic demonstrates how scientists believe endosymbiosis occurred: All cells began as their prokaryotic ancestors with nucleic acids free-floating in the cytoplasm and without a nucleus. As the cells grew in size, they needed to increase their surface area so they could interact with their environment. This caused an infolding of their plasma membrane. The infolding of the plasma membrane eventually caused the development of endoplasmic reticulum, a nuclear envelope, and a well-defined nucleus. The cell eventually engulfed aerobic heterotrophic prokaryotes, the precursors to mitochondria. This resulted in the ancestral heterotrophic eukaryotic cell. Some cells engulfed photosynthetic prokaryotes, the precursors to chloroplasts. This resulted in the ancestral photosynthetic eukaryote. According to serial endosymbiosis theory, mitochondria and chloroplasts were incorporated into early eukaryotes in a particular order. Specifically, mitochondria evolved before chloroplasts. The early prokaryote engulfed and incorporated a prokaryotic cell that became a mitochondrion, and then it engulfed a similar cell that became a chloroplast. When Lynn Margulis published this theory in 1981, she was laughed at. Today, it is the most widely accepted theory for the emergence of mitochondria and chloroplasts in early eukaryotes. Although it is certainly not conclusive, considerable evidence exists to support this theory: • Chloroplasts, mitochondria, and prokaryotes share similar inner membranes, both in terms of structure and function. • Chloroplasts and mitochondria have their own genomes separate from that of the host organism. They transcribe and translate their own DNA, and they divide independently. • Ribosomes in chloroplasts and mitochondria are more similar to prokaryotic ribosomes than eukaryotic ribosomes. Dr. Gillooly pointed out that scientists have seen endosymbiosis at work in the laboratory. For example Hatena arenicola is a single-celled eukaryote that starts as heterotrophic but then transitions into an autotroph when it engulfs and incorporates a photosynthetic algae cell. When it divides, one daughter cell will contain the algal cell (so it will be autotraphic), while the other daughter cell will not contain the algal cell (so it will be heterotrophic). The Evolution of Multicellularity Scientists believe that multicellularity first evolved approximately 1.2 billion years ago, though multicellularity has evolved multiple times in history. Multicellularity expanded the possibilities in terms of organismal form and function. It gave rise to a diverse array of organisms, such as algae, plants, fungi, and animals. The most widely accepted theory of multicellularity is the colonial theory, which holds that individuals of the same species began to live colonially, in symbiotic relationships. Over time, their association became closer and closer, until they became a single, autonomously acting, multicellular organism. For example, Volvox is a genus of green algae whose members are colonies of individual algal cells that work together cooperatively. The colonial theory is the theory most widely accepted among scientists, probably because of the insight we have gained from modern-day colonial organisms such as sponges and algae. Even ants, bees, and wasps live in colonies. In class, Dr. Gillooly showed a huge ant colony that biologists are currently excavating. Many consider colonies of ants as a "superorganism" that takes on a life of its own. Most of the major groups of complex animals on the Earth today arose in the Cambrian explosion approximately 525 to 535 million years ago. The data reveal that large-scale adaptive radiations commonly follow mass extinction events. It was during this time that the first predator-prey interactions likely occurred. Trilobites, for example, were predatory marine arthropods that first appeared in the early Cambrian. The Burgess Shale formation in British Columbia, Canada is famous for the fossils that are exceptionally well-preserved there. This fossil bed has provided scientists with a lot of information about the kinds of biodiversity that emerged during the Cambrian explosion. There have been five big mass extinctions in Earth's history, and each has been followed by on increase in species diversity. This is because the surviving species were able to thrive, and new species were able to exploit the same resources and habitats previously used by the species that went extinct during the mass extinction. A major contributor to the diversification of eukaryotic life was the ability to live on land, which began about 500 million years ago. Tetrapods-which along with arthropods are the most widespread and diverse terrestrial animals-evolved from lobe-finned fishes approximately 365 million years ago. Dr. Gillooly showed pictures of a coelacanth and a lungfish, evolutionarily ancient fishes that can still be found in the Earth's oceans and lakes today. (Interestingly, the coelacanth produces a single egg about the size of a golf ball, and it incubates the egg in its mouth!) Continental Drift Continental drift is the process whereby the continental plates slowly move over the planet's hot mantle. When these plates interact-such as by separating, colliding, or moving past one another-they can cause geological events, such as earthquakes, lava flow, and the formation of islands and mountains. These events have major implications for species diversity on Earth. Scientists believe that the Earth's continents have come together to form one giant landmass at least three times in history: 1.1 billion years ago, 600 million years ago, and 250 million years ago. The landmass resulting from the most recent coming together of Earth 's continents is known as Pangea. The formation and subsequent breakup of Pangea had major effects on global climate, ocean circulation, and speciation. Practice question: A homoplasy is another term for ______ _ a. divergent evolution b. convergent evolution c. homology d. parsimony e. More than one of these is correct. The answer is “b” - Convergent evolution (homoplasy) creates analogous structures with similar form or function, leaving two species with similar structures even though they are not closely related. Practice question: Neutral changes to DNA sequences and vestigial traits are good examples of ... a. acclimation b. adaptation c. nonadaptive traits d. convergent traits e. developmental homologies The answer is “c” - Neutral changes to DNA sequences are nonadaptive because they do not affect an individual's fitness. Likewise, vestigial structures have been retained but no longer have the function that they once did. As a result, they do not improve an organism's fitness and are therefore nonadaptive.