Cell Biology I PDF
Document Details
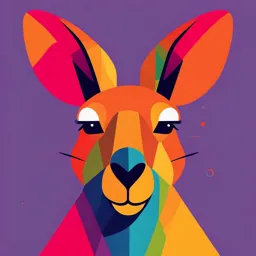
Uploaded by CalmBaritoneSaxophone
Helwan University
Dr. Rania EL-Lethy Abdelhamed EL-Lethy
Tags
Summary
This document presents lectures on “Cell Biology I” for Biochemistry Students at Helwan University. It covers the different structures and functions of cells, including the organization of organelles, their physiological properties, metabolic processes, and interactions with their environment.
Full Transcript
CELL BIOLOGY I Third level, Biochemistry Students Code: BCh3105 Dr. Rania EL-Lethy Abdelhamed EL-Lethy Lecturer of Biochemistry, Chemistry Department,.Faculty of Science, Helwan University CELL BIOLOGY I BY: DR. RANIA ELLETHY Cell biology or cytology or cytobiolo...
CELL BIOLOGY I Third level, Biochemistry Students Code: BCh3105 Dr. Rania EL-Lethy Abdelhamed EL-Lethy Lecturer of Biochemistry, Chemistry Department,.Faculty of Science, Helwan University CELL BIOLOGY I BY: DR. RANIA ELLETHY Cell biology or cytology or cytobiology, is a branch of biology that studies the different structures and functions of the cell and focuses mainly on the idea of the cell as the basic unit of life. Cell biology explains the structure, organization of the organelles they contain, their physiological properties, metabolic processes, signaling pathways, life cycle, and interactions with their environment. This is done both on a microscopicThis is done both on a microscopic and molecularThis is done both on a microscopic and molecular level as it encompasses prokaryotic cellsThis is done both on a microscopic and molecular level as it encompasses prokaryotic cells and eukaryotic cells. *Cells are the smallest form of life—the functional and structural units of all living things. *Your body contains trillions of cells, organized into more than 200 major types. *At any given time, each cell is doing thousands of jobs. Some of these tasks are so essential for life that they are carried out by virtually all cells. Others are done only by cells that are highly skilled for the work, whether it is covering up your insides (skin cells), preventing you from sloshing around like a pile of goo (bone cells), purging your body of toxic chemicals (liver cells), or enabling you to learn and remember (brain cells). Cells also must make the products your body needs, such as sweat, saliva, enzymes, hormones, and antibodies. Your body contains many different cell types, each customized for a particular role. * Red blood cells carry life-giving oxygen to every corner of your body, * white blood cells kill germ invaders, * intestinal cells squirt out chemicals that chisel away at your food so you can absorb its nutrients, * nerve cells sling chemical and electrical messages that allow you to think and move, and * heart cells constantly pump blood, enabling life itself. THE BLOOD Blood is the most important fluid in the body. Blood is bright red when its hemoglobin is oxygenated. Blood is circulated around the body through blood vessels by the pumping action of the heart. Blood accounts for 7% of the human body weight, The average adult has a blood volume of roughly 5 liters (1.3 gal). Blood pH is regulated to stay within the narrow range of 7.35 to 7.45, making it slightly basic. Blood7 that has a pH below 7.35 is too acidic, whereas blood pH above 7.45 is too basic. :FUNCTIONS OF BLOOD 1. Supply of oxygen to tissues (bound to hemoglobin, which is carried in red cells) 2. Supply of nutrients such as glucose2. Supply of nutrients such as glucose, amino acids2. Supply of nutrients such as glucose, amino acids, and fatty acids (dissolved in the blood or bound to plasma proteins. 3.Removal of waste such as carbon dioxide3.Removal of waste such as carbon dioxide, urea3.Removal of waste such as carbon dioxide, urea, and lactic acid. 4.Immunological functions, including circulation of white blood cells, and detection of foreign material by antibodies. 5.Coagulation, which is one part of the body's self-repair mechanism (blood clotting after an open wound in order to stop 8 bleeding). 6.Messenger functions, including the transport of hormones and the signaling of tissue damage. 7.Regulation of body pH. 8.Regulation of core body temperature. :CONSTITUENTS OF HUMAN BLOOD 9 Blood Blood Plasma Cells Platelets = RBCʼS = WBCʼS = Thrombocyt Erythrocytes Leucocytes es 1) ERYTHROCYTES (RED CELLS): The erythrocytes are the most numerous blood cells. Mature red blood cells lack a nucleusa nucleus and organelles in mammals. In man and in all mammals, erythrocytes are devoid of a nucleus and have the shape of a biconcave lens. In the other vertebrates (e.g. fishes, reptilians and birds), they have a nucleus. These cells are responsible for providing oxygen to tissues and partly for recovering carbon dioxide produced as waste. The mean life of erythrocytes is about 120 days. 2) LEUKOCYTES (WHITE CELLS): These are colourless, nucleated cells. Leukocytes are responsible for the defense of the organism. White blood cells are part of the body's immune system. In the blood, they are much less numerous than red cells. 12 A. NEUTROPHILS : Also known as, polymorph or microphage. The cytoplasm is full of granules and the nucleus has about 3 – 7 lobes connected by chromatin strand. They are very active in phagocyting bacteria and are present in large amount in the pus of wounds. The life13period is about 3 -5 days. B. BASOPHIL : Its cytoplasm contains small rounded granules & the nucleus is usually kidney shaped. The life period is 8 – 12 days. Secrets a chemical substance, known as heparin, which prevents the clotting of blood 14 inside the blood vessel. C. EOSINOPHILS: Also known as bilobed cells, as nucleus contains two lobes connected with chromatin strand. The life period is 8 – 10 days. It attacks parasites. 15 D. LYMPHOCYTE S: It characterized by absence of granules in the cytoplasm. The nucleus large in size & rounded in shape which occupies a major part of the cell. The cytoplasm is very reduced. The16 life period is very short, less than 24 hrs. It produces antibody. E. MONOCYTES: They are the largest of all WBCʼS. The nucleus is kidney shaped. They are the precursors of macrophages. Macrophages cooperate in the immune defense. They produce substances which have defensive functions such as lysozime and interferons. The life period is about 1 -2 days. 3) PLATELETS (THROMBOCYTES): These are the smallest of all blood cells. They are spindle shaped cells. They are responsible for blood clotting (coagulation) by changing fibrinogen into fibrin. The life period is of few hrs. 18 4. PLASMA: About 55% of blood is blood plasma. It is straw-yellow in color. It is essentially an aqueous solution containing 92% water, 8% blood plasma proteins, and trace amounts of other materials. Plasma circulates dissolved nutrients, such as glucosePlasma circulates dissolved nutrients, such as glucose, amino acidsPlasma circulates dissolved nutrients, such as glucose, amino acids, and fatty acidsPlasma circulates dissolved nutrients, such as glucose, amino acids, and fatty acids, and removes waste products, such as carbon dioxidePlasma circulates dissolved nutrients, such as glucose, amino acids, and fatty acids, and removes waste products, such as carbon dioxide, ureaPlasma circulates dissolved nutrients, such as glucose, amino acids, and fatty acids, and removes waste products, such as carbon dioxide, urea, and lactic acid. COUNTING OF RBCʼS: Hemocytometer The hemocytometer is a device used to count cellsThe hemocytometer is a device used to count cells. It was originally designed for the countingThe hemocytometer is a device used to count cells. It was originally designed for the counting of blood cells. The hemocytometer consists of a thick glass microscope slide with a rectangular indentation that creates a chamber.. The hemocytometer consists of two chambers, each of which is divided into nine 1.0 mm squares. A cover glass is supported over the chambers and cell suspensions are introduced under the cover glass. The hemocytometer is placed on the microscope stage and the cell suspension is counted. EMPTY HEMOCYTOMETER GRID AT 100X.POWER 23 *The hemocytometer chamber consists of outer four corners squares each with 1 mm (as in fig. no. 1, 2, 3, 4), and are divided into 16 small squares, 16 x 4 = 64 small squares ( that for total WBCʼS count). Total WBC count = N x 50. *The central square in same chamber (as in fig. no. 5) divided into 25 squares, each square is subdivided in 16 small squares for RBCʼS count, count the cells in each corner and one central square, i.e. total 5 square, as each square is divided into 16 small square, 16 x 5 = 80 small squares. Total RBC count = N x 10,000. N is the number of cells counted. APPLICATIONS OF HEMOCYTOMETER: Blood counts: for patients with abnormal blood cells, where automated counters don't perform well. Sperm counts. Cell culture: when subculturing or recording cell growth over time. Beer brewing: for the preparation of the yeast. Cell processing for downstream analysis: accurate cell numbers are needed in many tests (PCR, flow cytometry). Measurement of cell size. Cell, in biology, the basic membrane-bound unit that contains the fundamental molecules of life and of which all living things are composed. A single cell is often a complete organism in itself, such as a bacteriumA single cell is often a complete organism in itself, such as a bacterium or yeast. Other cells acquire specialized functions as they mature. These cells cooperate with other specialized cells and become the building blocks of large multicellular organisms, such as animals and humans. Although cells are much larger than atoms, they are still very small. Cells are structural units that make up plants and animals; also, there are many single celled organisms. What all living cells have in common is that they are small 'sacks' composed mostly of water. The 'sacks' are made from a phospholipid bilayer membrane. This membrane is semipermeable (allowing some things to pass in or out of the cell while blocking others). Elements · 59% Hydrogen (H) · 24% Oxygen (O) · 11% Carbon (C) · 4% Nitrogen (N) · 2% Others - Phosphorus (P), Sulphur (S), etc. Molecules · 50% protein · 15% nucleic acid · 15% carbohydrates · 10% lipids · 10% Other As an individual unit, the cell is capable of : metabolizing its own nutrients, synthesizing many types of molecules, providing its own energy, and replicating itself in order to produce succeeding generations. It can be viewed as an enclosed vessel, within which innumerable chemical reactions take place simultaneously. These reactions are under very precise control so that they contribute to the life and procreation of the cell. In a multicellular organism, cells become specialized to perform different functions through the process of differentiation. In order to do this, each cell keeps in constant communication with its neighbours. As it receives nutrients from and expels wastes into its surroundings, it adheres to and cooperates with other cells. Cooperative assemblies of similar cells form tissues, and a cooperation between tissues in turn forms organs, which carry out the functions necessary to sustain the life of an organism. A typical animal cell, sliced open to reveal cross-sections.of organelles Animal cell Principal structures of an animal cell Cytoplasm surrounds the cell's specialized structures, or organelles. Ribosomes, the sites of protein synthesis, are found free in the cytoplasm or attached to the endoplasmic reticulum, through which materials are transported throughout the cell. Energy needed by the cell is released by the mitochondria. The Golgi complex, important for glycosylation, secretion. Here, proteins and other molecules are prepared for shipping outside of the cell. * Digestive enzymes are contained in lysosomes. * Peroxisomes contain enzymes that detoxify dangerous substances. Use oxygen to carry out catabolic reactions, in both plant and animals. In this organelle, an enzyme called catalase is used to break down hydrogen peroxide into water and oxygen gas. * The centrosome contains the centrioles, which play a role in cell division. * The microvilli are fingerlike extensions found on certain cells. Cilia, hair like structures that extend from the surface of many cells, can create movement of surrounding fluid. The nuclear envelope, a double membrane surrounding the nucleus, contains pores that control the movement of substances into and out of the nucleoplasm. Chromatin, a combination of DNA and proteins that coil into chromosomes, makes up much of the nucleoplasm. The dense nucleolus is the site of ribosome production. CELL NUCLEUS ENDOPLASMIC RETICULUM (ER) RIBOSOME GOLGI APPARATUS Lysosome MITOCHONDRIA CENTROSOME CHLOROPLAST PEROXISOME THE NATURE AND FUNCTION OF CELLS A cell is enclosed by a plasma membrane, which forms a selective barrier that allows nutrients to enter and waste products to leave. The interior of the cell is organized into many specialized compartments, or organelles, each surrounded by a separate membrane. One major organelle, the nucleus, contains the genetic information necessary for cell growth and reproduction. Each cell contains only one nucleus, whereas other types of organelles are present in multiple copies in the cellular contents, or cytoplasm. Organelles include: mitochondria, which are responsible for the energy transactions necessary for cell survival; lysosomes, which digest unwanted materials within the cell; and the endoplasmic reticulum and the Golgi apparatus, which play important roles in the internal organization of the cell by synthesizing selected molecules and then processing, sorting, and directing them to their proper locations. In addition, plant cells contain chloroplasts, which are responsible for photosynthesis, whereby the energy of sunlight is used to convert molecules of carbon dioxide (CO2) and water (H2O) into carbohydrates. Between all these organelles is the space in the cytoplasm called the cytosol. The cytosol contains an organized framework of fibrous molecules that constitute the cytoskeleton, which gives a cell its shape, enables organelles to move within the cell, and provides a mechanism by which the cell itself can move. The cytosol also contains more than 10,000 different kinds of molecules that are involved in cellular biosynthesis, the process of making large biological molecules from small ones. Animal cells and plant cells contain membrane-bound organelles, including a distinct nucleus. In contrast, bacterial cells do not contain organelles Specialized organelles are a characteristic of cells of organisms known as eukaryotes. In contrast, cells of organisms known as prokaryotes do not contain organelles and are generally smaller than eukaryotic cells. However, all cells share strong similarities in biochemical function. PROKARYOTIC VS. EUKARYOTIC CELLS Two classes of cells exist: the PROKARYOTES and the EUKARYOTES. 1) The Prokaryotes - include the bacteria and the blue-green algae (the Monera kingdom). - These are all single-celled organisms that lack both a true nucleus and other membrane-bounded cellular substructures. - Prokaryotic DNA is usually circular. 2) The Eukaryotes - include plants, animals, protozoa, and fungi. - These cells contain nuclei and other membrane-bound organelles. The genetic material is organized into chromosomes. Virtually all forms of life fall into one of two categories: eukaryotes or prokaryotes. The eukaryotic cell The molecules of cells Cells contain a special collection of molecules that are enclosed by a membrane. These molecules give cells the ability to grow and reproduce. The overall process of cellular reproduction occurs in two steps: cell growth and cell division. During cell growth, the cell ingests certain molecules from its surroundings by selectively carrying them through its cell membrane. Once inside the cell, these molecules are subjected to the action of highly specialized, large, elaborately folded molecules called enzymes. Enzymes act as catalysts by binding to ingested molecules and regulating the rate at which they are chemically altered. These chemical alterations make the molecules more useful to the cell. Unlike the ingested molecules, catalysts are not chemically altered themselves during the reaction, allowing one catalyst to regulate a specific chemical reaction in many molecules. Biological catalysts create chains of reactions. In other words, a molecule chemically transformed by one catalyst serves as the starting material, or substrate, of a second catalyst and so on. In this way, catalysts use the small molecules brought into the cell from the outside environment to create increasingly complex reaction products. These products are used for cell growth and the replication of genetic material. Once the genetic material has been copied and there are sufficient molecules to support cell division, the cell divides to create two daughter cells. Through many such cycles of cell growth and division, each parent cell can give rise to millions of daughter cells, in the process converting large amounts of inanimate matter into biologically active molecules. ENZYME S ***All reactions in the body are mediated by enzymes, which are protein catalysts that increase the rate of reactions without being changed in the overall process. **Among the many biologic reactions that are energetically possible, enzymes selectively channel reactants (called substrates) into useful pathways. **Enzymes thus direct all metabolic events. NOMENCLATURE A. Recommended name Most commonly used enzyme names have the suffix “-ase” attached to the substrate of the reaction (for example, glucosidase and urease), or to a description of the action performed (for example, lactate dehydrogenase and adenylyl cyclase). B. Systematic name In the systematic naming system, enzymes are divided into six major classes (Figure), each with numerous subgroups. Figure: The six major classes of enzymes with examples. (THF = tetrahydrofolate). Enzymes are protein catalysts that increase the velocity of a chemical reaction, and are not consumed during the reaction. A. Active sites Enzyme molecules contain a special pocket or cleft called the active site. The active site contains amino acid side chains that participate in substrate binding and catalysis (Figure ). The substrate binds the enzyme, forming an enzyme–substrate (ES) complex. Binding is thought to cause a conformational change in the enzyme (induced fit) that allows catalysis. ES is converted to an enzyme–product (EP) complex that subsequently dissociates to enzyme and product. Figure: Schematic representation of an enzyme with one active site binding a substrate molecule. B. Catalytic efficiency Enzyme-catalyzed reactions are highly efficient, proceeding from 10³–10 8 times faster than uncatalyzed reactions. The number of molecules of substrate converted to product per enzyme molecule per second is called the turnover number, or k cat and typically is 10²–104 s-1. C. Specificity Enzymes are highly specific, interacting with one or a few substrates and catalyzing only one type of chemical reaction. [Note: The set of enzymes made in a cell determines which metabolic pathways occur in that cell.] D. Holoenzymes Some enzymes require molecules other than proteins for enzymic activity. The term holoenzyme refers to the active enzyme with its nonprotein component, whereas the enzyme without its nonprotein moiety is termed an apoenzyme and is inactive. If the nonprotein moiety is a metal ion such as Zn2+ or Fe2+, it is called a cofactor. If it is a small organic molecule, it is termed a coenzyme. Coenzymes that only associate with the enzyme are called cosubstrates. Cosubstrates dissociate from the enzyme in an altered state (NAD+ is an example). If the coenzyme is permanently associated with the enzyme and returned to its original form, it is called a prosthetic group (FAD is an example). Coenzymes frequently are derived from vitamins. For example, NAD+ contains niacin and FAD contains riboflavin. E. Regulation Enzyme activity can be regulated, that is, increased or decreased, so that the rate of product formation responds to cellular need. F. Location within the cell Many enzymes are localized in specific organelles within the cell (Figure ). Such serves to isolate the reaction substrate or product from other competing reactions. This provides a favorable environment for the reaction, and organizes the thousands of enzymes present in the cell into purposeful pathways. Figure: The intracellul ar location of some important biochemic al pathways. REGULATION OF METABOLISM: The pathways of metabolism must be coordinated so that the production of energy or the synthesis of end products meets the needs of the cell. Furthermore, individual cells do not function in isolation but, rather, are part of a community of interacting tissues. Thus, a communication system has evolved to coordinate the functions of the body. Regulatory signals that inform an individual cell of the metabolic state of the body as a whole include hormones, neurotransmitters, and the availability of nutrients. These, in turn, influence signals generated within the cell (Figure). Figure: Some commonly used mechanisms for transmission of regulatory signals between cells. A. Signals from within the cell (intracellular): The rate of a metabolic pathway can respond to regulatory signals that arise from within the cell. For example, the rate of a pathway may be influenced by the availability of substrates, product inhibition, or alterations in the levels of allosteric activators or inhibitors. These intracellular signals typically elicit rapid responses, and are important for the moment-to-moment regulation of metabolism. B. Communication between cells (intercellular): The ability to respond to extracellular signals is essential for the survival and development of all organisms. Signaling between cells provides for long-range integration of metabolism, and usually results in a response that is slower than is seen with signals that originate within the cell. Communication between cells can be mediated, for example, by surface-to-surface contact and, in some tissues, by formation of gap junctions, allowing direct communication between the cytoplasms of adjacent cells. However, for energy metabolism, the most important route of communication is chemical signaling between cells by bloodborne hormones or by neurotransmitters. TRANSPORT OF GLUCOSE INTO CELLS: Glucose cannot diffuse directly into cells, but enters by one of two transport mechanisms: A- Na+-independent facilitated diffusion transport system. B- or Na+-monosaccharide cotransporter system. A. Na+-independent facilitated diffusion transport:- This system is mediated by a family of 14 glucose transporters in cell membranes. They are designated GLUT-1 to GLUT-14 (glucose transporter isoforms 1–14). These transporters exist in the membrane in two conformational states (Figure). Extra cellular glucose binds to the transporter, which then alters its conformation, transporting glucose across the cell membrane. Figure: Schematic representation of the facilitated transport of glucose through a cell membrane. [Note: GLUT proteins contain 12 tran-smembrane helices.] B. Na+-monosaccharide cotransporter system:- This is an energy-requiring process that transports glucose “against” a concentration gradient—that is, from low glucose concentrations outside the cell to higher concentrations within the cell. This system is a carrier-mediated process in which the movement of glucose is coupled to the concentration gradient of Na+, which is transported into the cell at the same time. The carrier is a sodium-dependent– glucose transporter or SGLT. This type of transport occurs in the epithelial cells of the intestine, renal tubules, and choroid plexus. The structure of biological molecules Approximate chemical composition of a typical mammalian cell component percent of total cell weight water 70 inorganic ions (sodium, potassium, magnesium, 1 calcium, chloride, etc.) miscellaneous small metabolites 3 proteins 18 RNA 1.1 DNA 0.25 phospholipids and other lipids 5 polysaccharides 2 Light Microscopes: *The First Windows Into Cells Scientists first saw cells by using traditional light microscopes. *In fact, it was Robert Hooke (1635–1703), looking through a microscope at a thin slice of cork, who coined the word “cell.” He chose the word to describe the boxlike holes in the plant cells because they reminded him of the cells of a monastery. Scientists gradually got better at grinding glass into lenses and at whipping up chemicals to selectively stain cellular parts so they could see them better. By the late 1800s, biologists already had identified some of the largest organelles (the nucleus, mitochondria, and Golgi). Researchers using high-tech light microscopes and glowing molecular labels can now watch biological processes in real time. The scientists start by chemically attaching a fluorescent dye or protein to a molecule that interests them. The colored glow then allows the scientists to locate the molecules in living cells and to track processes—such as cell movement, division, or infection—that involve the molecules. Fluorescent labels come in many colors, including brilliant red, magenta, yellow, green, and blue. By using a collection of them at the same time, researchers can label multiple structures inside a cell and can track several processes at once. The technicolor result provides great insight into living cells—and is stunning cellular art. *Robert Hooke, the British scientist who coined the word “cell,” probably used this microscope when he prepared Micrographia. Published in 1665, Micrographia was the first book describing observations made through a microscope. THE CELL CYCLE AND CHECKPOINTS ❖ Thecell cycle or cell-division cycle is the series of events that take place in a cell leading to its division and duplication of its DNA (DNA replication) to produce two daughter cells. ❖In cells with a nucleus, as in eukaryotes, the cell cycle is also divided into three periods: interphase, the mitotic (M) phase, and cytokinesis. During interphase, the cell grows, accumulating nutrients needed for mitosis, preparing it for cell division and duplicating its DNA. During the mitotic phase, the cell splits itself into two distinct daughter cells. During the final stage, cytokinesis, the new cell is completely divided. To ensure the proper division of the cell, there are control mechanisms known as cell cycle checkpoints. CELL CYCLE PHASES G0 phase “Gap 0”: A resting phase where the cell has left the cycle and has stopped dividing. Nonproliferative cells in multicellular eukaryotes generally enter the quiescent G0 state from G1 and may remain quiescent for long periods of time, possibly indefinitely (as is often the case for neurons and may remain quiescent for long periods of time, possibly indefinitely (as is often the case for neurons). This is very common for cells that are fully differentiated. Cellular senescence occurs in response to DNA damage or degradation that would make a cell's progeny nonviable; for example, become cancerous. Some cells enter the G0 phase semi-permanently e.g., some liver, kidney, stomach cells. Many cells do not enter G0 and continue to divide throughout an organism's life, e.g. epithelial cells. Interphase Before a cell can enter cell division, it needs to take in nutrients. All of the preparations are done during interphase. Interphase is a series of changes that takes place in a newly formed cell and its nucleus, before it becomes capable of division again. It is also called preparatory phase or intermitosis. Typically interphase lasts for at least 90% of the total time required for the cell cycle. Interphase proceeds in three stages, G1, S, and G2, preceded by the previous cycle of mitosis and cytokinesis. The cell's nuclear chromosomes are duplicated during S phase. G1 Phase “Gap 1”: The first phase within interphase, from the end of the previous M phase until the beginning of DNA synthesis, is called G1 (G indicating gap). It is also called the growth phase. In this phase, the cell increases its supply of proteins, increases the number of organelles (such as mitochondria, ribosomes), and grows in size. The G1 checkpoint control mechanism ensures that everything is ready for DNA synthesis. S Phase: DNA replication occurs during this phase. The S phase starts when DNA replication starts; when it is completed, all of the chromosomes have been replicated, i.e., each chromosome has two (sister) chromatids. Thus, during this phase, the amount of DNA in the cell has effectively doubled, though the ploidy of the cell remains the same. During this phase, synthesis is completed as quickly as possible due to the exposed base pairs being sensitive to harmful external factors such as mutagens. Substances that cause DNA mutations are known as mutagens, mutagens that cause cancers are known as carcinogens. G2 Phase “Gap 2”: During the gap between DNA synthesis and mitosis, the cell will continue to grow. The G2 checkpoint control mechanism ensures that everything is ready to enter the M (mitosis) phase and divide. Mitotic “M’ phase ‘” Cell division”: Cell growth stops at this stage and cellular energy is focused on the orderly division into two daughter cells. A checkpoint in the middle of mitosis (Metaphase Checkpoint) ensures that the cell is ready to complete cell division. M phase is complex and highly regulated. The sequence of events is divided into phases, corresponding to the completion of one set of activities and the start of the next. These phases are sequentially known as: prophase, metaphase, anaphase, telophase cytokinesis (cytokinesis is not part of mitosis but is an event that directly follows mitosis in which cytoplasm is divided into two daughter cells) early prophase, the cell starts to break down some structures and build others up, setting the stage for division of the chromosomes. The chromosomes start to condense (making them easier to pull apart later on). The mitotic spindle begins to form. The spindle is a structure made of microtubules, strong fibers that are part of the cell’s “skeleton.” Its job is to organize the chromosomes and move them around during mitosis. The spindle grows between the centrosomes as they move apart. The nucleolus (or nucleoli, plural), a part of the nucleus where ribosomes are made, disappears. This is a sign that the nucleus is getting ready to break down. In late prophase (sometimes also called prometaphase), the mitotic spindle begins to capture and organize the chromosomes. The chromosomes finish condensing, so they are very compact. The nuclear envelope breaks down, releasing the chromosomes. The mitotic spindle grows more, and some of the microtubules start to “capture” chromosomes. Microtubules can bind to chromosomes at the kinetochore, a patch of protein found on the centromere of each sister chromatid. (Centromeres are the regions of DNA where the sister chromatids are most tightly connected.) Microtubules that bind a chromosome are called kinetochore microtubules. Microtubules that don’t bind to kinetochores can grab on to microtubules from the opposite pole, stabilizing the spindle. More microtubules extend from each centrosome towards the edge of the cell, forming a structure called the aster. In metaphase, the spindle has captured all the chromosomes and lined them up at the middle of the cell, ready to divide. All the chromosomes align at the metaphase plate (not a physical structure, just a term for the plane where the chromosomes line up). At this stage, the two kinetochores of each chromosome should be attached to microtubules from opposite spindle poles. Before proceeding to anaphase, the cell will check to make sure that all the chromosomes are at the metaphase plate with their kinetochores correctly attached to microtubules. This is called the spindle checkpoint and helps ensure that the sister chromatids will split evenly between the two daughter cells when they separate in the next step. If a chromosome is not properly aligned or attached, the cell will halt division until the problem is fixed. In anaphase, the sister chromatids separate from each other and are pulled towards opposite ends of the cell. The protein “glue” that holds the sister chromatids together is broken down, allowing them to separate. Each is now its own chromosome. The chromosomes of each pair are pulled towards opposite ends of the cell. Microtubules not attached to chromosomes elongate and push apart, separating the poles and making the cell longer. In telophase, the cell is nearly done dividing, and it starts to re-establish its normal structures as cytokinesis (division of the cell contents) takes place. The mitotic spindle is broken down into its building blocks. Two new nuclei form, one for each set of chromosomes. Nuclear membranes and nucleoli reappear. The chromosomes begin to decondense and return to their “stringy” form. Mitosis is the process by which a eukaryotic cell separates the chromosomes in its cell nucleus into two identical sets in two nuclei. During the process of mitosis the pairs of chromosomes condense and attach to fibers that pull the sister chromatids to opposite sides of the cell. It is generally followed immediately by cytokinesis, which divides the nuclei, cytoplasm, organelles and cell membrane into two cells containing roughly equal shares of these cellular components. Mitosis and cytokinesis together define the mitotic (M) phase of the cell cycle – the division of the mother cell into two daughter cells, genetically identical to each other and to their parent cell. This accounts for approximately 10% of the cell cycle. Mitosis occurs exclusively in eukaryotic cells, but occurs in different ways in different species. For example, animals undergo an "open" mitosis, where the nuclear envelope breaks down before the chromosomes separate, while fungi such as Aspergillus nidulans and Saccharomyces cerevisiae (yeast) undergo a "closed" mitosis, where chromosomes divide within an intact cell nucleus. Prokaryotic Prokaryotic cells, which lack a nucleus, divide by a process called binary fission. ❖The interval between each cell division is defined as a cell cycle. Each cycle consists of four ordered phases, namely G1 (gap 1), S (DNA synthesis), G2 (gap 2) and M (mitosis/meiosis) (Fig.). ❖DNA replication occurs during S phase; chromosomal separation and cell division during M phase; and G1 and G2 are gap or growth phases. Fig.: Normal cell cycle Checkpoints are pauses in the cell cycle during which the fidelity of DNA duplication and accuracy of chromosome segregation are monitored. These checkpoints allow for any defects to be edited and repaired, thereby assuring that the daughter cells receive the full complement of genetic information, identical to that of the parent cell. Any cell population is basically composed of three subpopulation of cells: active or cycling cells that continuously proliferate going from one mitosis to the other, terminally differentiated cells that irreversibly leave the cell cycle and die without dividing and resting or non-dividing cells (G0) that are not cycling and do not divide but can re-enter the cell cycle by appropriate stimulus e.g. stem cells of the bone marrow. All cycling cells pass through the four phases of the cell cycle, namely G1, S, G2 and M. The duration of the phases of the cell cycle varies depending on the cell type, the frequency with which the cells divide, and host characteristics such as the presence of appropriate growth factors. Very rapidly dividing cells can complete the cell cycle in less than 8 hours, whereas others can take longer than 1 year. Most of this variability occurs in the G0 and G1 phases. The duration of the S phase (10 to 20 hours), the G2 phase (2 to 10 hours), and the M phase (0.5 to 1 hour) appears to be relatively constant. A subset of genes encodes the “checkpoint control functions” and constitutes a surveillance system. Two main groups are identified: 1. Checkpoints for initiating S phase (G1/S): the most important members are the p53 and Rb tumor suppressor genes; 2. Checkpoints for initiation of mitosis (G2/M): this group includes the RRC1 gene (repressor of chromosome condensation) and pim-1 (premature initiation of mitosis). The G1 checkpoint or regulation point ensures two functions: adequate machinery for future events and accurate transmission of genetic information. Adequate machinery involves relief of Rb inhibition by sequential changes in gene activity, resulting in protein synthesis. Phosphorylation of serine and threonine hydroxyl groups by protein kinases is a key feature of these regulatory events. Fidelity of genetic information transfer is maintained by a specific mechanism for detecting and eliminating damaged DNA. Cells have a delay process mediated by the p53 suppressor protein, which is activated when DNA damage is detected. In fact, p53 and Rb act in concert to ensure transition through this stage of the cycle. The G2 checkpoint ensures the elimination of damaged cells that may have escaped G1 control or that have not duplicated their DNA accurately. The spindle assembly checkpoint in the M phase monitors accurate chromosome alignment and retraction into the two daughter cells. ONCOGENES Oncogene, or cancer gene, was so named to account for the properties of a viral gene that caused cancers in animal cells. Oncogenes are described by a three-letter code usually derived from their first discovery. Thus, the ras oncogene refers to a gene originally identified in rat sarcomas and the name abl derives from its first discovery in the Ableson virus. The erb gene, identified in the erythroblastosis virus, is now divided into erbA and erbB categories. Oncogenes are normal regulatory genes whose activity is increased as a consequence of genetic alteration. This gain of function can be due to qualitative or quantitative change in the protein product. Only one allele of an oncogene needs to be changed for a biological effect to occur. The effect is dominant. Oncogenes can be activated by mutation in a coding sequence that generates an altered product. The function of many metabolic pathways can be altered as a result of oncogene activation. These include membrane receptors, signal transduction and gene transcription. Oncogenes are genes that gain oncogenic or transforming potential as a result of genetic changes in either their coding region or regulatory sequences. The gene present in normal cells is called a proto-oncogene to distinguish it from the altered gene in the cancer cell. The normal gene is sometimes referred to as a cellular oncogene (c-onc) to distinguish it from its viral homologues (v-onc). The term ‘proto-oncogene’, which becomes an oncogene after its expression is altered. More than 60 oncogenes have been identified. An oncogene is contained in each of the diverse regulatory pathways that govern cell behaviour. Selected examples are shown in Table 5.3. TUMOUR SUPPRESSOR GENES Tumour suppressor genes were identified later when it became clear that normal cells contained inhibitory functions (suppressors) whose loss resulted in uncontrolled growth. Tumour suppressor genes have a more varied terminology; two- and three letter codes are used in some situations, and the size of the protein product is used in others. Thus, Rb refers to the retinoblastoma gene and Bcl2 describes a gene first identified in a B-cell lymphoma. The ‘2’ was added to distinguish it from another gene in the same tumour type. The p53 gene is so named because the protein synthesized from its coding sequence has a molecular mass of 53 000 daltons (53 kDa). Tumour suppressor genes code for inhibitory proteins whose function is lost in cancers. Both gene copies of a diploid cell usually must be lost before a biological effect is seen. This type of gene is recessive; p53 is an exception in that mutation in one allele generates an abnormal p53 that inactivates the normal product of the other allele. The first mutation is dominant-negative. Tumour suppressor proteins can be inactivated by protein phosphorylation, mutation or binding to other proteins. Carcinogenic DNA viruses code for tumour-suppressor-binding proteins or may act by indirect methods. Many pathways are affected by tumour suppressor genes, including those regulating cell proliferation and death. The basis of their importance is that in normal cells, growth and other functions are restricted by inhibitory (suppressor) proteins that must be reversibly inactivated for growth to occur. TUMOUR SUPPRESSOR GENE INACTIVATION Tumour suppressor proteins inhibit cell functions by complexing with other effector proteins and blocking their action. Inactivation of the tumour suppressor gene product and therefore the lifting of its blocking effect is achieved by preventing its binding to the effector protein, a process that can be achieved in several ways.(Figure 5.8). Figure: Methods of tumour suppressor inactivation. In quiescent normal cells, proliferation is blocked because Rb protein binds and inactivates a transcription factor. Serine/threonine phosphorylation of Rb protein disrupts this interaction, thereby releasing the cell-cycle block characteristic of this protein. Mutation resulting in altered product or loss of product is another way of escaping from this inhibition. Examples of tumour suppressor genes inactivated in human cancers are given in Table 5.5; Both p53 and Rb genes are nonessential for progression through the division cycle. Cells with no Rb or p53 divide perfectly well. However, both gene products negatively regulate progression of the cell cycle by blocking cells in late G1. Cells with normal p53 pause in late G1 for repair, while cells with mutant p53 fail to pause, enter S phase and have poor genomic stability. The Rb protein bound to the transcription factor E2F, blocks the expression of a number of genes that encode enzyme activities needed to synthesize deoxyribonucleoside triphosphates, the precursors of DNA replication. Absence of Rb results in abnormal expression of such enzyme activities at the wrong stages of the cell cycle resulting also in genomic instability. Genetic Control of Cell Cycle and Proliferation: The three fundamental components of the cell cycle are chromosomal replication (S phase), doubling of other cellular components and mitosis. Growth in size occurs throughout the cell cycle at a steady pace, but the S phase and mitosis are all-or-none actions, triggered by subtle changes in the environment (e.g. growth factors and proto-oncogenes). Several cellular proteins are necessary for completion of DNA replication. Also the machinery responsible for the detection and repair of mismatched DNA bases i.e. DNA mismatch repair genes, hMSH2, hMLH1 and hPMS2 show cyclic changes during the cell cycle. Although hMLHl mRNA remains constant, the protein level increases during late G1 and S phase. The levels of hPMS2 mRNA fluctuates and the protein level decreases in G1 and increases in S phase. Cyclins: Cellular proliferation follows an orderly progression through the cell cycle, which is controlled by protein complexes of cyclins and cyclin dependent kinases (cdks). Cyclins are a heterogeneous set of proteins that are expressed at determined phases of the cell cycle and rapidly degraded. They are encoded by different genes, e.g. CLN1, CLN2 and CLN3. Their regulatory role is achieved by phosphorylation of cellular proteins, e.g. Rb gene. Five classes of' cyclins termed A-E and their cdk co-factors (cdks 1-4) have been identified. While cyclins act as regulatory molecules, cdks act as their catalytic subunits, by forming cyclin-cdk complexes. Tumor proliferation is governed by growth-regulating genes, that have direct or indirect effect on the cell cycle. The effect may be a positive one in proto- oncogenes or a negative one in tumor suppressor genes. The following genes are allocated in the different compartments of the cell cycle: 1. Mitosis: The induction of mitosis is done through the activation of p34 by phosphorylation catalyzed by CDC2. In humans, mitosis associated cyclins have been cloned and sequenced. c-mos is important since it is involved in the phosphorylation of cyclin B which is required for mitosis. 2. G1 Phase: Early growth-regulated genes are set of transcripts neces sary for the G0 to G1 transition. The prototypes include c-myc, c-fos and c-jun. On the other hand, other genes are necessary for the G0 induction. These include gas (growth-arrested genes) that are over expressed in all G0 cells, statin genes which are over expressed in growth-arrested and senescent cells, and pro-inhibition whose mRNA inhibits entry into S phase. Cyclin D1-cdk4 complex controls passage through G1. The role of such cyclins in the control of the G1-S transition is one of the keys to future investigation for tumor growth control. 3. S Phase: DNA synthesis genes and genes coding for enzymes required for the synthesis of the DNA building blocks are required for chromosomal replication and are induced at the G1-S boundary. They encode for important products including: DNA polymerase α, replication protein A (RP-A), protein phosphatase 2A, proliferating cell nuclear antigen (PCNA), cyclin A and thymidine kinase. Chromosomal proteins (e.g. histones and non-histones) are also necessary to protect the integrity of chromosomal structure and func tion. Inhibition of the synthesis of these proteins leads to cessation of DNA replication. Tumor suppressor genes (e.g. p53 and Rb) can act on the S phase promoting an anti-proliferation action possibly by binding to cyclin E or induction of apoptosis. Cyclin E-cdk2 complex controls entry into S phase. 4. G2 Phase: This is a rather passive phase in which the cell is preparing for mitosis. The activation of p34 by cyclin B1 and cyclin B2 starts at the end of G2 to induce mitosis. The process is initiated by cyclin B1 and terminated by cyclin B2. Cell Death In each cell line, the control of cell number is regulated by a balance of cell proliferation and cell death. There are two major mechanisms of cell death-necrosis and apoptosis. Cells that are damaged by external injury undergo necrosis, while cells that are induced to commit programmed suicide because of internal or external stimuli undergo apoptosis. Apoptotic cell death involves controlled cell destruction and is involved in normal cell deletion and renewal. Necrotic cell death is a pathologic form of cell death resulting from cell injury. It is characterized by cell swelling, rupture of the cell membrane, and inflammation. 1. Apoptosis Apoptosis is a highly organized and genetically controlled type of cell death. Apoptosis is a gene-directed, active, programmed, self-inflicted cellular death; also known as programmed cell death. Programmed cell death starts by chromatin condensation and fragmentation of the nucleus into small apoptotic bodies due to action of calcium magnesium-dependent endonuclease enzyme that cleaves chromatin at internucleosomal sites (Fig.). Fig.: Illustration of the various stages of apoptotic cell death. A: depiction of the stereotypical changes including condensation changes in nuclear structure, and fragmentation of the cell into small apoptotic bodies. In vivo, the apoptotic bodies are phagocytosed by neighboring cells, whereas in vitro they undergo swelling and eventual lysis (secondary necrosis). B: photographs of LLC-PK1 cells undergoing apoptosis at the corresponding stages as shown in A. Morphologic Features of Apoptosis: Most normal cell types can be induced to undergo apoptosis if given the appropriate stimulus. Several morphologic changes are characteristic of cells dying of apoptosis. They pass through different sequential stages: 1. General Features: Cells dying of apoptosis detach themselves from their neighbors and from culture substrata. There is loss of specialized surface structures, such as microvilli and contact regions giving the cell a smooth contour. On the whole, there is cell shrinkage with reduced cell volume ending in cell break into membrane-bound "apoptotic bodies". These contain intact organelles and fragments of condensed chromatin. Apoptotic cells generally show small hyper chromatic nuclei in a markedly eosinophilic cytoplasm. 2. Nuclear Changes: There is initial condensation of chromatin into crescents along the nuclear envelope with subsequent thickening and irregularity. Later the chromatin collapses into an amorphous dark osmophilic mass with fre quent nuclear fragmentation. This causes reduction in nuclear size. 3. Cytoplasmic Changes: These follow the nuclear changes. First, there is compacting of cytoplasmic organelles like the golgi apparatus, lysosomes and mitochondria. Later, margination of such organelles towards the cell membrane occurs. 4. Cell Membrane Changes: The changes in plasma membrane also pass through stages of ruffling, blebbing and then thinning. Apoptotic cells are recognized by adjacent cells and macrophages and are rapidly engulfed before the dying cell is able to release its contents and thereby induce an inflammatory response. When phagocytosed by a macrophage, the cell is seen as an apoptotic body. In contrast, necrosis, an alternative mechanism of cell death, is characterized by a direct external stimulus that causes uncontrolled swelling of cells to be followed by rupture of plasma membrane with pouring of contents into tissues initiating an inflammatory reaction. Characteristics that distinguish apoptosis from necrosis are demonstrated in (Table) Apoptosis Necrosis Patterns of Single cells Groups of death neighboring cells Cell size Shrinkage, Fragmentation Swelling Plasma membrane Preserved continuity Smoothing Blebbed Early lysis Phosphatidylserine on surface Mitochondria Increased membrane permeability. Swelling Contents released into cytoplasm. Disordered structure Cytochrome c; Apaf1. Structure relatively preserved Organelle shape Contracted, "Apoptotic bodies" Swelling, Disruption Chromatin: Nuclei Membrane disruption Clumps & Fragmented Fragmented Internucleosomal cleavage DNA degradation Free 3' ends Diffuse & Random Laddering on electrophoresis DNA appears in cytoplasm Phagocytosis Inflammation Cell degradation No inflammation Macrophage invasion REFERENCES 1. Alberts, Bruce; Johnson, Alexander D.; Morgan, David; Raff, Martin; Roberts, Keith; Walter, Peter (2015). "Cells and genomes". Molecular Biology of the Cell (6th ed.). New York, NY: Garland Science. pp. 1–42. ISBN 978-0815344322. 2. ^ Bisceglia, Nick. "Cell Biology". Scitable. www.nature.com. 3. ^ Jump up to:a b c Gupta, P. (1 December 2005). Cell and Molecular Biology. Rastogi Publications. p. 11. ISBN 978-8171338177. 4. ^ Hooke, Robert (September 1665). Micrographia. 5. ^ Jump up to:a b Chubb, Gilbert Charles (1911). "Cytology". In Chisholm, Hugh (ed.). Encyclopædia Britannica. Vol. 7 (11th ed.). Cambridge University Press. p. 710. 1. ^ Paez-Espino D, Eloe-Fadrosh EA, Pavlopoulos GA, Thomas AD, Huntemann M, Mikhailova N, Rubin E, Ivanova NN, Kyrpides NC (August 2016). "Uncovering Earth's virome". Nature. 536 (7617): 425–30. Bibcode:2016Natur.536..425P. doi:10.1038/nature19094. PMID 27533034. S2CID 4466854. 2. ^ Lavanya, P. (1 December 2005). Cell and Molecular Biology. Rastogi Publications. p. 11. ISBN 978-8171338177. 3. ^ Jump up to:a b c d e f Cooper, Geoffrey M. (2000). "Tools of Cell Biology". The Cell: A Molecular Approach. 2nd Edition. 4. ^ McKinnon, Katherine M. (21 February 2018). "Flow Cytometry: An Overview". Current Protocols in Immunology. 120: 5.1.1–5.1.11. doi:10.1002/cpim.40. ISSN 1934-3671. PMC 5939936. PMID 29512141. 5. ^ Doble, Mukesh; Gummadi, Sathyanarayana N. (5 August 2010). Biochemical Engineering. New Delhi: Prentice-Hall of India Pvt.Ltd. ISBN 978-8120330528. 1. Kaneshiro, Edna (2 May 2001). Cell Physiology Sourcebook: A Molecular Approach (3rd ed.). Academic Press. ISBN 978-0123877383. 2. ^ Levetin, Estelle; McMahon, Karen (16 October 2014). Ebook: Plants and Society. McGraw Hill. p. 135. ISBN 978-0-07-717206-0. 3. ^ Jump up to:a b c d Nelson, Daniel (22 June 2018). "The Difference Between Eukaryotic And Prokaryotic Cells". Science Trends. doi:10.31988/scitrends.20655. S2CID 91382191. 4. ^ Griffiths, Anthony J.F.; Miller, Jeffrey H.; Suzuki, David T.; Lewontin, Richard C.; Gelbart, William M. (2000). "Bacterial conjugation". An Introduction to Genetic Analysis. 7th Edition. 5. ^ De Rooij, Johan (25 June 2019). "F1000Prime recommendation of Force Triggers YAP Nuclear Entry by Regulating Transport across Nuclear Pores". doi:10.3410/f.732079699.793561846. S2CID 198355737. 6. ^ "Nucleus". Genome.gov. Retrieved 27 September 2021. 7. ^ "Endoplasmic Reticulum (Rough and Smooth) | British Society for Cell Biology". Retrieved 6 October 2019. 1. Studios, Andrew Rader. "Biology4Kids.com: Cell Structure: Endoplasmic Reticulum". www.biology4kids.com. Retrieved 27 September 2021. 2. ^ "Powerhouse of the cell has self-preservation mechanism". EurekAlert!. Retrieved 27 September 2021. 3. ^ Pelley, John W. (2007), "Citric Acid Cycle, Electron Transport Chain, and Oxidative Phosphorylation", Elsevier's Integrated Biochemistry, Elsevier, pp. 55–63, doi:10.1016/b978-0-323-03410-4.50013-4, ISBN 978032 3034104 4. ^ Cooper, Geoffrey M. (2000). "The Golgi Apparatus". The Cell: A Molecular Approach. 2nd Edition. 5. ^ Verity, M A. Lysosomes: some pathologic implications. OCLC 679070471. 6. ^ "Ribosome | cytology". Encyclopedia Britannica. Retrieved 27 September 2021. 7. ^ The CELL A MOLEQULAR APPROACH. Geoffrey M Cooper. 2000. 1. Cooper, Geoffrey M. (2000). "Transport of Small Molecules". The Cell: A Molecular Approach. 2nd Edition. 2. ^ "What Are the Main Functions of Cilia & Flagella?". Sciencing. Retrieved 23 November 2020. 3. ^ Jump up to:a b Ahmad, Maria; Kahwaji, Chadi I. (2019), "Biochemistry, Electron Transport Chain", StatPearls, StatPearls Publishing, PMID 30252361, retrieved 20 October 2019 4. ^ Schlessinger, Joseph (October 2000). "Cell Signaling by Receptor Tyrosine Kinases". Cell. 103 (2): 211–225. doi:10.1016/s0092-8674(00)00114-8. ISSN 0092-8674. P MID 11057895. S2CID 11465988. 5. ^ Shackelford, R E; Kaufmann, W K; Paules, R S (February 1999). "Cell cycle control, checkpoint mechanisms, and genotoxic stress". Environmental Health Perspectives. 107 (suppl 1): 5–24. doi:10.1289/ehp.99107s15. ISSN 0091-6765. PMC 1566366. PMID 10229703. 6. ^ Jump up to:a b Bernstein C, Bernstein H, Payne C. Cell immortality: maintenance of cell division potential. Prog Mol Subcell Biol. 2000;24:23-50. doi:10.1007/978-3-662-06227-2_2. PMID 10547857. THANK YOU