Semiconductor Devices PDF
Document Details
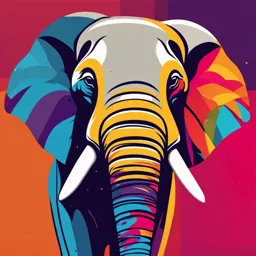
Uploaded by BetterHafnium126
IIT
Tags
Summary
This document provides an overview of semiconductor devices, covering their types, characteristics, and working principles. It details PN junction diodes, their use in rectification, and other semiconductor devices like tunnel diodes, Zener diodes, LEDs, photodiodes, and transistors.
Full Transcript
Advanced Engineering Materials UNIT 2 SEMICONDUCTOR DEVICES 2.1.INTRODUCTION In the previous chapter we have studied Band theory of solids, Fermi energy concept along with energy band structure of metals, insulato...
Advanced Engineering Materials UNIT 2 SEMICONDUCTOR DEVICES 2.1.INTRODUCTION In the previous chapter we have studied Band theory of solids, Fermi energy concept along with energy band structure of metals, insulators and semiconductors. Also, the details regarding current conduction in intrinsic and extrinsic semiconductors and their energy band diagrams at 0K and at higher temperatures were discussed. One of the important successes of the band theory of solids was theoretical understanding of semiconductors and their physical properties which we will learn in this unit. There are various devices which having wide range of applications in Electronics. All these devices are based on Semiconductor theory. In this chapter we are going to study Types of Semiconductor diode, the characteristics and working of devices like P-N junction diode, Tunnel Diode, Zener Diode, LED and Photodiode and their applications. We will also discuss Hall effect and its applications, applications of diode as rectifier, types of Transistors and applications of Transistor as amplifier in CB and CE mode. 2.2. PN JUNCTION DIODE A PN junction diode is also known as a semiconductor diode. It is formed when a P- type and an N-type semiconductor are joined metallurgically. It allows current flow in one direction only. Hence, it can act as rectifier to convert ac voltage to dc voltage. APN junction diode is schematically represented by the symbol shown in fig.2.1. Fig2.1: Symbolic representation of PN junction diode The arrowhead indicates the conventional direction of current flow when the diode is forward biased. The P-side of the diode is positive and is called anode. The N-side is the cathode and is the negative terminal when the diode is forward biased. The word ‘diode’ stands for two electrodes- anode and cathode. 2.3. FORMATION OF POTENTIAL BARRIER IN PN JUNCTION DIODE 1 Advanced Engineering Materials Fig.2.2: Illustration of depletion region in P-N junction diode A PN junction is formed when P-type and N-type semiconductor are joined metallurgically. The boundary between P-region and N-region is called PN junction. In P-region, holes are majority charge carriers and in N-region, electrons are majority charge carriers. This creates concentration gradient near the junction. Because of the concentration difference, holes nearer to the junction begin todiffuse from p-side to the n-side (see above Fig. a, b). Similarly, the electrons in the n-region aremore than the electrons in the p-region. Due to the concentration gradient, electrons nearer tothe junction begin to diffuse from n-side to the p-side. Thus, majority carriers start moving intoopposite regions. As electrons and holes are charged particles, their motion produces electron diffusion current, Jen and hole diffusion current, Jhprespectively.This motion of electrons from N-region to P-region and motion of holes from P-region move towards N-region produces diffusion current When the hole from P-region enters the N-region, it combines with electron over there and electron -hole pair disappears, this is called recombination process. Similarly, when electron from N-region enters the P-region, it is surrounded by large no. of holes, which leads to recombination process. At the junction the holes and electrons meet each other and undergo recombination. As a hole recombines with an electron, both the hole and electron disappear. This leads to the disappearance of mobile charge carriers in the junction region Further, the holes coming out of P-region leave behind negative acceptor ions and electrons from N-region leaves behind positive donor ions near to the junction. The double layer of ions around the junction is known as the space charge region. This narrow space-charge region is depleted of mobile charges and contains only the immobile uncompensated ions. Therefore, this region is also called the depletion region, Depletion region is a narrow region of oppositely charged ions on either side of PN junction which is depleted of mobile charge carriers(Fig.1). These oppositely charged fixed ions produce internal electric field E directed from the donor ions on N region towards the acceptor ions on P region at the junction. This electric field is in a direction that opposes the diffusion of majority carriers into opposite sides. The electric field establishes potential difference Vo or an energy hill of height eVo at the junction, which acts as a barrier for majority charge carriers to cross the junction, hence called as potential barrier. Thus, the internal electric field acts as a barrier to the flow of majority charge carriers. The barrier increasestill majority carriers cannot diffuse further across the junction. Due to application of internal electric field E, minority carriers i.e. electrons drift from P region to N region and holes drift from N region to P region. An electric current 2 Advanced Engineering Materials flows across the junction. This current, which is caused by electric field, is called drift current At thermal equilibrium the net diffusion current throughthe junction must be equal and opposite to the net drift current so that the total current is zero. This condition is called UNBIASED condition of PN-JUNCTION diode. QUE : Explain the formation of depletion region in a pn junction diode. (3)[Summer-04, 11, 12 &16] QUE : How is depletion region formed in a junction of the diode. (3)[Winter-16] QUE : What is meant by depletion region? (2)[Summer-17] QUE : Explain the mechanism of formation of depletion layer in P-N Junction diode. How does the potential barrier arise in the process? (5)[Winter-09] QUE: Explain the formation of potential barrier across the junction of semiconductor diode. (3) [Summer-05] 2.3.1. UNBIASED PN-JUNCTION When a PN-junction diode is not connected with any external supply it is called as unbiased. Once equilibrium is attained no net current will flow through itbecause diffusion current becomes equal to drift current, hence diode is at equilibrium. The electric field establishes potential difference Vo at the junction, which act as a barrier for majority charge carriers to cross the junction. The oppositely charged ions produces electric fieldE at the junction directed as shown in fig. 2.3(a). 2.3.2. BIASING OF PN-JUNCTION DIODE When dc voltage is applied to the diode it is said to be biased. A PN-junction diode is biased in two ways:(1) Forward biasing (2) Reverse biasing. (1)Forward biasing: When positive terminal of battery is connected to the P-region and negative terminal of source (battery) is connected to the N-region, the diode is said to be forward biased. If this external applied voltage‘V’ becomes greater than the value of the potential barrierVo,the potential barrier’s opposition will be overcome. The width of depletion region reduces. The potential barrier reduces by value (Vo – V) as shown in fig.2.3(b). Hence majority charge carriers start crossing the junction andlargecurrent starts flowing. Vo is approx. 0.7 volts for silicon and 0.3 volts for germanium. (2)Reverse biasing: When negative terminal of battery is connected to the P-region andpositiveterminalofsource (battery) is connected to the N-region, the diode is said to be reversebiased. The width of depletion region increases. The potential barrier increases to (Vo + V)as shown in fig.2.3(c). Hence majority charge carriers are unable to crossthe junction. The direction of electric field supports the flow of minority charge carriers across the junction, hence a small amount of current flows. 3 Advanced Engineering Materials Forward biasing Reverse biasing Fig.2.3 (a) Unbiased diode(b) Forward biasing (c) Reverse biasing 4 Advanced Engineering Materials 2.4 DRIFT & DIFFUSION CURRENT 2.4.1. DRIFT CURRENT The charge carriers start drifting due to the application of electric field to the semiconductor and produces drift current. Drift current is produced due to electric field. Its value depends upon the minority charge carrier concentration and electric field. More the temperature, more is the drift current. DIFFUSION CURRENT Due to the concentration gradient in a semiconductor, the charge carriers move from higher concentration level towards lower concentration level to maintain the equilibrium and this movement of carriers produces diffusion current. For diffusion current, electric field is not required. It depends upon the rate of change of carrier concentration per unit length. More the doping more is the diffusion current. QUE : What is drift and diffusion current ? (2)[Summer-14] QUE : Explain drift and diffusion current. (2) [Summer-11, Winter-14] QUE : Explain the terms (i) drift current (ii) diffusion current. (3)[Winter-17] QUE : Differentiate between drift and diffusion curent. (3) [Summer-03,Winter-18] 2.5. V-I CHARACTERSTICS AND WORKING OF PN-JUNCTION DIODE Fig.2.4 shows forward as well as reverse characteristics of PN junction diode. Fig.2.4: V-I characteristics of P-N junction diode. Forward Bias: When the junction is forward biased, practically no current flows until the barrier voltage is overcome. With further increase in voltage, current starts flowing & curve has a linear rise like an ordinary conductor. The voltage at which current starts flowing through the diode is known as cut- in voltage. It is the minimum value of voltage required to overcome potential barrier across the junction. This voltage is 0.3 for Germanium and 0.7 V for silicon. The current in forward bias mode is large (in mA) due to the movement of majority charge carriers across the junction. 5 Advanced Engineering Materials Reverse Bias: When a junction is reversed biased, junction resistance increases with the increase in reverse biased voltage(potential barrier)& very small current flow through it due to minority charge carriers. As reverse voltage increases, the minority charge carriers acquire sufficient kinetic energy. These accelerated charge carriers cross the junction and ionize the semiconductor atoms in the depletion region leading to generation of free electrons and holes. These electrons and holes in turn get accelerated and cause further ionization of atoms in P and N regions. This process is called Avalanche breakdown and leads to sharp increase in current at a reverse bias voltage called breakdown voltage. Thus, breakdown voltage is the applied reverse voltage at which large current flows through the diode. In reverse bias condition the current through diode is very small in the range of μA due to movement of minority charge carriers. 2.6 PN JUNCTION DIODE AS RECTIFIER The process in which alternating voltage or alternating current is converted into direct voltage or direct current is known as rectification. In our country domestic supply has a voltage 220V (rms) varying at a frequency 50Hz. For operating most of the electronic equipment, dc voltage is required. Hence it is necessary to convert ac voltage of main power supply to dc voltage. The device used for this conversion process is called as rectifier. The junction diode has the property of offering low resistance and allowing current to flow through it in the forward biased condition. This property is used in the process of rectification. A PN junction diode can be used as: Half wave rectifier Full wave rectifier Bridge rectifier. 2.6.1 HALF WAVE RECTIFIER: Fig.2.5: Half wave rectifier Half Wave Rectifier is a circuit which converts ac signal to dc signal by employing a single PN junction diode for rectification purpose as shown in fig.2.5. In half wave rectifier circuit, an alternating voltage is applied to a single diode connected in series with a load resistor RL. 6 Advanced Engineering Materials An ac signal full wave consists of half positive and half negative wave. PN junction diode conducts during only one half cycle of the input ac cycle. During a positive half cycle, diode D is forward biased hence conducts and transfers the half wave. Diode offers low resistance hence a large current IL flows through the load resistor RL. Output voltage Vo develops across RL and transfers the half wave. During a negative half cycle, diode D is reverse biased hence does not conduct. Therefore, IL=0 and hence gives no output (Vo=0).This implies that negative half cycle of input voltage is not utilized for delivering power to the load. As the output contains only positive half cycles i.e., unidirectional half waves, the circuit is called as half wave rectifier. 2.6.2 FULL WAVE RECTIFIER: Fig.2.6: Full wave rectifier Full Wave Rectifier is a circuit which converts ac signal to dc signal by using two PN junction diodes for rectification purpose as shown in fig.2.6. In full wave rectifier circuit, diodes conduct (One diode conduct during each half cycle) during both the half cycles of input ac voltage. A full wave ac signal input consists of half positive and half negative wave. It is provided to the full wave rectifier from a transformer with a centre -tapped secondary winding. (A center-tap transformer is designed to provide two separate secondary voltages, VA and VB with a common connection. This type of transformer configuration produces a two-phase, 3-wire supply. The secondary voltages are the same and proportional to the supply voltage, VP, therefore power in each winding is the same). During a positive half cycle of secondary voltage, diode D1 is forward biased hence conducts and transfers the half wave. Current IL flows through diode D1, the load resistor RL and the upper half-winding of the secondary. Output voltage Vo develops across RL. D2 is reverse biased. During a negative half cycle, diode D2 is forward biased hence conducts and transfers the half wave. Current IL flows through diode D2, the load resistor R L and the lower half-winding of the secondary. Output voltage Vo develops across RL. D1is reverse biased. The direction of current flows through the load resistor RL in both cases is same. During the both half cycles, current IL passes through the load resistor R L and produces output voltage Vo. Thus, in Full wave rectifier, both the half cycles are utilized to produce the output. As the output contains only positive continuous series of positive half cycles of alternating voltage i.e., unidirectional waves for each input half wave, the circuit is called as Full wave rectifier. The input and output wave forms are as shown in fig.2.6. 7 Advanced Engineering Materials 2.6.3BRIDGE RECTIFIER Fig.2.7: Bridge rectifier The bridge rectifier is constructed by using four individual rectifying diodes connected in a closed loop “bridge” configuration to produce the desired output. The main advantage of this bridge circuit is that it does not require a special centre tapped transformer, thereby reducing its size and cost. The single secondary winding is connected to one side of the diode bridge network and the load (RL) to the other side as shown in fig.2.7. The four diodes labelled D1 to D4 are arranged in “series pairs” with only two diodes conducting current during each half cycle. During the positive half cycle of the supply, diodes D1 and D3 conduct in series while diodes D2 and D4 are reverse biased and the current IL flows through the load (RL) is as shown in fig.2.7. During the negative half cycle of the supply, diodes D2 and D4 conduct in series, but diodes D1 and D3 are switched “OFF” as they are now reverse biased. The current IL flowing through the load (RL) is in the same direction as before. As the current flowing through the load is unidirectional, so the voltage developed across the load is also unidirectional, the same as for the previous two diode full- wave rectifier. 2.7 ZENER DIODE Zener diode is a specially designed ordinary P-N junction diode, which is heavily doped to have a very sharp and almost vertical breakdown. They are exclusively operated under reverse bias conditions and designed to operate in breakdown region without damage. The device was named after Clarence Zener, who discovered this electrical property. The commonly used schematic symbol for Zener diode is shown in Fig.2.8. Fig: 2.8: Symbolic representation of Zener diode 2.7.1. V-I CHARACTERSTICSAND WORKING OF ZENER DIODE 8 Advanced Engineering Materials Fig.2.9: V-I Characteristics of Zener diode. The Forward bias characteristics of Zener diode are same as that of normal PN Junction diode. When the applied forward bias voltage ‘VF’ is less than the cut in voltage, the current is negligibly small.WhenVF becomes greater than cut in voltage,current starts increasing rapidly. In reverse bias mode, current is due to minority charge carriers. Since the P and N-regions are heavily doped, the depletion layer at the junction will be very narrow. The reverse bias voltage sets up a strong electric field across the narrow depletion layer. This field is strong enough to cause rupture of covalent bonds of atoms.Therefore, there is a generation of a large number of electron-hole pairs, leading to a sharp increase in the reverse current. When reverse bias is increased, upto a certain voltage called as breakdown voltagea voltage is reached then the diode starts conducting heavily and the reverse current increases sharply. This voltage is called Zener breakdown voltage(Vz). A Zener diode maintains a constant voltage across its terminals when the reverse bias exceeds the breakdown voltage.Therefore, it is used as voltage regulator. 2.7.2. APPLICATIONS OF ZENER DIODE Zener diode is used as voltage regulator. It is used as a fixed reference voltage in a network for biasing and comparison. It is used for calibrating voltmeters. It is used for avoiding meter damage by accidental application of excessive voltage. QUE: Explain forward and reverse bias characteristics of Zener diode. (4) [Summer-13] QUE: Explain V-I characteristics of Zener Diode. (3)[Summer -15, 16] 2.7.3. DIFFERENCE BETWEEN ZENER BREAKDOWN AND AVALANCHE BREAKDOWN 9 Advanced Engineering Materials Table 2.1: Differences between Zener breakdown and avalanche breakdown Zener breakdown Avalanche breakdown 1.This occurs at junctions which are heavily 1.This occurs at junctions which are lightly doped with narrow depletion region. doped with wide depletion layer. 2.This breakdown voltage sets up a very strong 2.In this case, electric field is not strong enough electric field across this narrow layer. to produce Zener type breakdown. 3.The electric field is very strong to rupture the 3.The minority carriers collide with covalent bonds thereby generating electron semiconductor atoms in the depletion region, hole pairs. So even a small increase in reverse which breaks the covalent bonds and electron- voltage is capable of producing large number hole pairs are generated. Newly generated of current carriers. Hence the junction has a charge carriers are accelerated by the electric very low resistance. This leads to Zener field which results in more collision and breakdown generates avalanche of charge carriers. This leads to avalanche breakdown. 4.Once Zener breakdown occurs, the 4. Once avalanche breakdown occurs, junction junction gets back to its normal position, gets destroyed completely and it cannot after the reverse voltage is removed from regain back its position. the diode. 5.Zener breakdown occurs when the operating 5.Avalanche breakdown occurs when the voltage is between 5 to 8 Volts. operating voltage is greater than 8 Volts. 2.8 TUNNEL DIODE The tunnel diode is a semiconductor diode. The current in this device is largely due to tunneling of electrons through a potential barrier. The rate of tunneling or current can be controlled over a wide range by varying the height of the barrier, which is done by varying the applied voltage. Tunnel diode is a heavily doped P-N junction diode with high conductivity of about 1000 times greater than a conventional junction diode. This is because the width of the depletion layer is reduced to extremely small value of the order of 100A0. Operation of tunnel diode utilizes the phenomenon of tunneling of electrons through the potential barrier of the junction. It exhibits negative resistance i.e., current through the diode decreases with the increase in voltage. This diode is also known as Esaki diode called after the name of the inventor Dr. Leo Esaki, received Nobel Prize in physics in 1973. The commonly used schematic symbol of tunnel diode is shown in Fig.2.10. Fig.2.10: Symbolic representation of tunnel diode 10 Advanced Engineering Materials 2.8.1. V-I CHARACTERSTICS AND WORKING OF TUNNEL DIODE Fig. 2.11: V-I characteristics of tunnel diode As a forward voltage across the tunnel diode is increased from zero, electrons from N region ‘tunnel’ through potential barrier to the P region. As a result, diode current increases until the peak point ‘P’ is reached. Till then the diode exhibits positive resistance. As the voltage is increased beyond VP, the tunneling action starts decreasing & diode current decreases till valley point ‘V’ is reached. In the region between peak point & valley point current decreases and the diode shows negative resistance (i.e., When the forward bias is increased, the current decreases). When forward bias is increased beyond valley point voltage V v , the tunnel diode behaves as a normal diode. The diode current increases with increase in forward voltage. 2.8.2. APPLICATIONS OF TUNNEL DIODE When operated in the negative resistanceregion, they can be used as oscillator. They are used in low power amplifiers. They can be used as switching device in computers. Since tunnel diodes are more resistant to nuclear radiation, they are used in space applications like amplifiers for satellite communications. QUE: Explain V-I characteristics of tunnel diode. (3)[Winter-12] QUE: What is tunnel diode, draw and explain its V-I characteristics. 2.9 PHOTO DIODE A photodiode is a type of light detector, used to convert the light energy into electrical energy (current or voltage) based on the mode of operation of the device. It is also called as light sensor. Typical photodiode materials are Silicon, Germanium, Indium Gallium Arsenide Phosphide and Indium gallium arsenide. The symbol of the diode is shown in fig,2.12. 11 Advanced Engineering Materials Fig.2.12: Symbol of photodiode 2.9.1. V-I CHARACTERISTICS AND WORKING OF PHOTODIODE: Fig.2.13: Working Principle of photodiode The Photodiode works in reverse bias mode. In this mode, the depletion region has large width. When reverse biased is applied to the diode, a small amount of current due to minority charge carriers is produced. This current is obtained without the incidence of light radiation and hence called as leakage current or dark current. Working Principle: When light photons of sufficient energy h Eg are incident on the depletion layer, electrons and holes are generated. This mechanism is called the inner photoelectric effect. A photon of energy hv ≥ Eg incident in or near the depletion layer of the diode will excite an electron from the valence band to the conduction band. This process generates a hole in the valence band. Thus, an electron-hole pair is generated by the optical photon. These are known as photocarriers. The electron-hole pairs generated in the depletion layer separates and drift in opposite directions under the action of the electric field. Such a transport process induces an electric current in the external circuit in excess of the already existing dark current (reverse leakage current). If the absorption of light takes place in the depletion region, then the charge carriers are removed from the junction by the inbuilt electric field of the depletion region. Therefore, the holes move towards the cathode, and electrons move towards the anode, and a photocurrent is generated as shown in fig.2.13. Optical excitation leads to an increase in the reverse-biased current. The V-I characteristics of photodiode is shown in fig.2.14. This current depends upon the intensity of incident radiation. 12 Advanced Engineering Materials Photocurrent is proportional to the incident light intensity. The amount of photocurrent generated increases as light intensity (IL) increases. The total current through the diode is the sum of dark current and photocurrent. To maximize the sensitivity of photodiode we need to minimize the dark current. It is desirable that the depletion region be sufficiently wide so that a large fraction of incident light can be absorbed. Therefore, the diode can be used as a photo detector— using a reverse bias voltage—as the measured photocurrent is proportional to the incident light intensity. Fig.2.14: V-I characteristics of photodiode Modes of Operation: The operating modes of the photodiode include three modes, namely Photovoltaic mode, Photoconductive mode, an avalanche diode mode. Photovoltaic Mode: In this mode, the diode is not connected to power source. We do not bias the diode. When the light falls in such condition on the photodiode, it excites the electrons to higher energy state and results in electrons to move towards cathode terminal and holes towards anode terminal. This process creates a potential difference between two terminals. Photoconductive Mode: In photoconductive mode, the diode is connected to the power source and diode is reverse biased. The reverse voltage application will increase the depletion layer’s width. When the light falls on the photodiode creates a pair of electrons and holes which move in the opposite direction due to biased voltage. Avalanche Diode Mode: An Avalanche photodiode is operated at reverse bias close to the breakdown voltage. When reverse-biased, there is a creation of strong fields in the junction region. When a photon generates an electron-hole pair, the pair flows through the junction. Because of the strong fields in the junction, the electron gains enough energy to cause ionisation of neutral atoms and secondary electrons and holes are generated. Thus, a multiplication (or avalanche) process takes place (hence the name), and a substantial current is generated from few initial photons as shown in fig.2.15. The avalanche photodiodes are good for those optical systems where intensity of incoming light signal is very low. 13 Advanced Engineering Materials Fig.2.15: Avalanche photodiode 2.9.3 APPLICATIONS: We can use the photodiode in smoke detectors to sense smoke and fire, a solar panel as solar cells, barcode scanner for character recognition, the obstacle detection system, printers as page presence and page counter, Optical message transmission, Fibre optics-based communication network as photo detector. It is also used as Position sensor. 2.10 LIGHT EMITTING DIODE(LED) LED is a specially made forward biased P-N junction diode, which emits light due to electron-hole recombination, when energized. This works on the principle of electroluminescence, the process in which electrical energy is converted into light energy. Henry Joseph Round was the first person to observe the phenomenon of electroluminescence in 1907 and invent the first LED. It is operated only in forward bias. The symbol of LED is shown in Fig. 2.16. Fig. 2.16:Symbolic representation of LED 14 Advanced Engineering Materials 2.10.1. V-I CHARACTERSTICS AND WORKING OF LED Fig. 2.17(a): Working of LED diode Fig. 2.17 (b): V-I characteristics of LED When Light Emitting Diode (LED) is forward biased, the free electrons from N-side and the holes from P-side move towards the junction as shown in fig.2.17(a) Like ordinary diode, the forward current is negligible up to a certain value of forward applied voltage due to the potential barrier across the PN-junction. When free electrons reach the junction, they overcome the potential barrier and recombine with the holes. In the similar way, holes from p-side recombine with electrons in the depletion region. Because of the recombination of free electrons and holes in the depletion region, the width of depletion region decreases. The recombination of free electrons and holes leads to generation of light. The wavelength of light emitted and its color depends on Energy Gap(Eg) of material used in making of LED. The wavelength of light emitted = where = ℎ =. For light to be emitted in visible region, Energy gap should be between 1.77eV to 3.11eV. Silicon and Germanium have bandgap energies that correspond to infrared light which is not visible. Energy gap of Silicon is 1.1eV and that of Germanium is 0.72eV. They are known as indirect- bandgap semiconductors. This means, it is not possible for electrons and holes to recombine directly and form a photon. Instead, you get a phonon (type of lattice vibration) which is effectively heat. An LED requires a direct- bandgap semiconductors having Energy gap ≥ 1.8eV.This can happen very efficiently in materials like Gallium Arsenide Phosphide (GaAsP) = 1.9eV and Gallium Phosphide (GaP) = 2.26eV.The Energy released in these materials produces intense visible light. The V-I characteristics of LED is shown in fig. 2.17(b).When the voltage applied overcomes the Energy gap(potential barrier), current increases rapidly as more number of charge carriers cross the PN junction. This voltage above which the diode starts conducting is called cut-in voltage of LED. The voltage after cut-in value remains almost constant once LED starts conducting like ordinary diode, but current increases rapidly. 2.10.2. APPLICATIONS OF LIGHT EMITTING DIODE LEDare commonly used as indicator in electronic circuits. They are used in electronic calculators to display the numbers. 15 Advanced Engineering Materials They are used in digital meters & electronic clocks. Infrared LEDs can be used as a source in optical fiber communications. LEDs are used in Burglar alarms systems, Calculators, Picture phones, Traffic signals, Digital computers, Microprocessors, Digital watches, Automotive heat lamps, Camera flashes and Aviation lighting. 2.10.3 ADVANTAGES OVER FILAMENT LAMPS Low operational voltage and less power is required to operate. Fast action and no warm-up time required. Long life. Fast on-off switching capacity. Emits monochromatic light. Smaller in size and lighter in weight. QUE: What is LED? Draw and explain its V-I characteristics. 2.11. TRANSISTORS Transistor was invented in 1947 by American Physicists Bardeen, Brattain and Schockley at Bell Telephone laboratories. A transistor is a semiconductor device that contains three regions separated by two distinct PN junctions. The two junctions are EB junction and CB junctions. The central region is called base. The two outer regions are called emitter and collector. There are two types of charge carriers, electrons and holes hence transistor is called bipolar transistor. Transistor can be viewed as two PN junction diodes arranged back-to-back with base being common to both the diodes. As soon as the two junctions are formed, majority charge carriers diffuse and form two depletion layers. Depletion layer is narrow at EB junction and wide at CB junction. QUE : What is a transistor ? (1)[Winter-18] There are two types of transistors: 1.NPN transistor--P-type sandwiched between two N-type. 2.PNP transistor--N-type sandwiched between two P-type. 16 Advanced Engineering Materials Fig.2.18: Block diagram and Symbolic representation of NPN & PNP transistor 2.11.1 FUNCTIONS OF THREE REGIONS IN TRANSISTOR: Emitter, Base and Collector are three regions in a transistor. Emitter: It emits charge carriers. It is heavily doped and has moderate size. It is located at the one end of transistor. Base: The base controls the flow of charge carriers from emitter to the collector. Therefore, it acts as a gate between emitter and collector. It has minimum thickness and is lightly doped. It makes the central region of a transistor. Collector: It collects the charge carriers coming from the base. It has largest size with moderate doping. It is moderately doped. It is at the other end of a transistor. 2.11.2Biasing of transistor The Emitter-Base (EB) junction of transistor is always forward biased, and Collector- Base (CB) junction of transistor is always reverse biased. Therefore, it works in an active mode. Hence, it transfers current from low resistance region (EB) to high resistance region (CB). It is seen that almost same current flows through the two junctions. Thus, the device is called as transistor- the shortened form of transfer resistor. 2.11.3. EXPLAIN WHY: Base region is lightly doped: If base region is heavily doped, more holes would be present in the base (for NPN transistor). The incoming electrons would then undergo more recombination with holes in base. This would decrease the no. of electrons entering the collector region. Hence the collector current will decrease and base current will increase. But in transistor circuits, more collector current and less base current is required. Hence to minimize base current base region is lightly doped. 17 Advanced Engineering Materials Base region is narrow: The main function of the base is to control the number of charge carriers from emitter to collector and hence offers easy and quick diffusion of carriers into collector region. If the width of the base is more, then it would lead to more number of recombination causing an increase in base current. But in transistor circuits, more collector current and less base current is required. Hence to offer quick diffusion of charge carriers and to keep base current to a minimum amount, the base region is made narrow. Emitter is heavily doped: The main function of the transistor is to emit charge carriers. In transistor circuit it is required that a maximum number of majority carriers are injected into the base so that the emitter current IE will be large. Hence to provide large no. of charge carriers emitter is heavily doped as compared to base and collector. Collector region is larger: The main function of collector is to collect all the charge carriers coming from base. Due to the movement of minority charge carriers across the reverse biased collector base junction, large amount of heat is produced as the minority charge carriers acquire large amount of kinetic energy. Hence to collect all the charge carriers and to dissipate away the heat, the collector region is made larger. QUE: Explain why in a transistor (i) The base is thin and lightly doped (ii) The collector is large in size. (2)[Winter-12, 15] QUE : Why the base region is thin and lightly doped in a transistor? Explain. (3)[Winter-16] QUE: Explain the function of emitter, base and collector in a transistor. (3)[Winter-12, Summer-18] QUE:Explain whythe emitter is heavily doped? (1) [Winter-18] 2.12 CIRCUIT CONFIGURATION COMMON BASE CONFIGURATION: Fig.2.19: Transistor in common base configuration In this circuit, Base terminal is made common to both input and output circuits. 18 Advanced Engineering Materials COMMON EMITTER CONFIGURATION: Fig.2.20: Transistor in common emitter configuration In this circuit, Emitter terminal is made common to both input and output circuits. COMMON COLLECTOR CONFIGURATION: Fig.2.21: Transistor in common collector configuration In this circuit, collector terminal is made common to both input and output circuits. 2.13. TRANSISTOR ACTION Fig.2.22 (a): Working of Transistor in common base mode 19 Advanced Engineering Materials Fig.2.22 (b): Working of Transistor in common base mode 1. Consider an NPN transistor biased by using two batteries VEE & VCC as shown in fig.2.22The emitter base junction is kept at forward biased by battery VEE & collector biased junction is kept as reverse biased junction by battery VCC. 2. As emitter base junction is forward biased the electron from emitter (E) moved towards base (B) & holes from base (B) moves towards emitter (E). Since emitter is highly doped & base is lightly doped, the emitter current IE is practically due to electron moving from emitter to base. 3. On entering the base region, electrons recombine with small no. of available holes in the base region, causing base current IB. Since the base region is light doped, the recombination is very less & hence IB is very less. 4. The electrons experience a very strong electric field due to reversed biased CB junction & get swept into the collector region. This constitutes collector current IC. Maximum number of electrons flow from emitter into the collector. This is possible due to light doping & small size of base region. 5. The total emitter current IE is given by I =I +I ---------- (1) E B C 2.14APPLICATIONS OF TRANSISTOR It is used in switching electronic circuits. It is used as an amplifier. It is used in integrated circuits. 2.14.1Application of Transistor as amplifier Transistor can work in three different regions like active region, cutoff region & saturation region. They are turned off while working in the cut-off region and turned on while working in the saturation region. Transistors work as an amplifier when they work in the active region. The main function of a transistor as an amplifier is to enhance the input signal. The input of the amplifier is a voltage otherwise current, where the output will be an amplified input signal. I. Common Emitter transistor amplifier: A transistor in which the emitter terminal is made common for both the input and the output circuit connections is known as common emitter configuration. When this configuration is provided with alternating current (AC) supply and operated in between both positive and the negative halves of a.c. cycle to generate the specific output signal is known as common emitter amplifier. In this type of configuration, the input is applied at the base terminal and the output is to be collected across the collector terminal. Emitter terminal is common in both the cases of input as well as output as shown in fig.2.23. 20 Advanced Engineering Materials Fig.2.23: Common Emitter Transistor Amplifier In this circuit, the Emitter Base junction is forward biased using base - emitter battery VEB and collector -Base junction is reverse biased using collector - emitter battery Vcc. The low input signal is applied to the emitter-base junction and the amplified output is obtained across the Rc load connected in the collector circuit. The collector current is controlled by base current. When input signal is applied to the base, very small change in base current produces very large changes in collector current. Therefore, the current gain is substantially high. a) D.C current Gain: It is the ratio of collector current to base current. It is denoted by ‘β’. ∴. , = --------------- (1) b) A.C current Gain: It is the ratio of change in collector current to change in base current. It is denoted by ‘βac’. ∆ ∴. , = --------------- (2) ∆ The value of β is between 20 to 500. c) A.C. Voltage Gain: It is the ratio of the a.c output voltage to a.c. input voltage. It is denoted by AV. ∴. , = = =-β ---------------- (3) where RB is the input resistance. The negative sign indicates that the output voltage is opposite with the phase of input voltage. d) Power gain The power gain Ap is the product of the current gain and voltage gain. Ap = βac * Av The power gain is also defined as the ratio of change in output power to the change in input power. 21 Advanced Engineering Materials II. Common base transistor Amplifier: In common base configuration, emitter is the input terminal, collector is the output terminal and base terminal is connected as a common terminal for both input and output. Fig.2.24:Common Base Transistor Amplifier Fig.2.24 shows NPN transistor connected in Common Base configuration. The transistor is biased to operate in the active region. The battery VEE forward biases the EB junction and the battery VCC reverse biases CB junction. As signal source Vi is connected to input circuit and a load resistance RL is connected in output circuit. An output voltage Vo is developed across RL. The input circuit has a low resistance. Hence a small change in the signal voltage will cause a significant change in the collector current due to transistor action. Thus, a large voltage drop occurs when collector current flows through load resistor. Hence a weak signal applied in input circuit gets amplified in the collector circuit. The output voltage is in phase with the input signal. a) Current gain (amplification factor): The ratio of the change in the collector current to the change in the emitter current at constant collector to base voltage (VCB) is called the current amplification factor α. 22 Advanced Engineering Materials ∆ = ∆ --------------- (5) The value of the current amplification factor α is always less than 1.The typical current gain of a common base amplifier is 0.98. b) Voltage Gain (G): It is the ratio of output signal voltage to input signal voltage. = -------------- (6) The emitter current variation ∆ due to emitter-base voltage variation is taken as ∆ = ---------------- (7) The change in collector current ∆ due to variation in emitter current ∆ is given by ∆ = ∆ ----------------- (8) This current ∆ causes a voltage drop, =∆ = ∆ =α ∆ ∴ = = =α ∆ = as α ≅ 1 is order of kilo- ohms, hence >>.Therefore, is larger than indicating that the transistor amplifies a small input voltage to large output voltage. 2.14.2Relation between α and β: ∆ ∆ = and = ∆ ∆ Since = + ∆ =∆ +∆ ∆ ∆ ∆ Dividing by ∆ , = + ∆ ∆ ∆ ∆ ∆ = +1 ∆ ∆ or = +1= = +1 = or = ( = − 1= =1− , = ) 23 Advanced Engineering Materials Hall effect 2.15. HALL EFFECT Statement: If a metal or a semiconductor carrying a current, I is placed in a transverse magnetic field B, a potential difference VH is produced in a direction normal to both the magnetic field and current directions. This is known as Hall Effect. Fig. 2.25: Hall Effect setup Determination of Hall Voltage Consider a thin wafer of P-type semiconductor crystal with holes the majority charge carriers. Let an electric field be applied to the it which produces a current I in the x-direction in the crystal. The Current through the semiconductor wafer is given by I = peA ----------- (1) where ‘p’ is the concentration of holes,’A’ is the area of cross section area of the end face of 24 Advanced Engineering Materials Semiconductor wafer =wtand is the drift velocity of holes. The current density is given by Jx = I / A Jx = pe ----------- (2) Let the magnetic field B be applied in the Z-direction. Theholes experience a Lorentz force FL due to the transverse magnetic field B and they are deflected towards the front face and make it positively charged while the rear face becomes negatively charged. Hence, a potential VH called the Hall Voltage is developed between the front and rear face. In the equilibrium state FE = FL eEH = Be or EH = B -----------(3) Since EH = where ‘w’ is the width of the wafer. Therefore = Bvd -------------(4) Hence vd = ------------ (5) Equation (5) can be used to calculate drift velocity of holes. From Eq. (2) = -------------- (6) Therefore Eqn. (5) and Eqn. (6)we get = = or VH = w or VH = w B since curent density Jx = If ‘t’is the thickness of semiconductor plate of area, A = wt, substituting value of A weget, Hall Voltage VH = = ---------- (7) With the direction of magnetic field and current as taken above, the sign of the Hall Voltage is positive. For an N-type semiconductor, Hall Volatge will be negative for same direction of current and Magnetic field. Thus knowing the sign ofHall Voltage, the type of semiconductor and sign of majority charge carrier can be known. 2.15.1. Hall coefficient RH : Hall coefficient RH is defined as Hall electric field per unit current density per unit magnetic induction. 25 Advanced Engineering Materials RH = = = [using eqn. (4.31)] or RH =. = --------- (8) Since Hall voltage VH = or VH = ----------- (9) Equation 9 gives the relation between Hall voltage and Hall coefficient. 2.15.2. HALL MOBILITY(µH): Hall mobility is defined as drift velocity acquired by the charge carriers per unit electric field. ∴ Hall mobility µH = ------------- (10) As J= pe And J = σ E where, σ is the conductivity of the material ∴pe = σE or = ∴µH = = = RH σ ----------- (11) where RH = Thus knowingRH and σ, Mobility of electrons and holes can be calculated using eqn.11. 2.15.3. CONCENTRATION OF MAJORITY CHARGE CARRIERS: RH = Hence hole concentration p = for p- type semiconductor. For N-type Semiconductor, n = -. Thus Carrier concentration in P type and N-type semiconductor can be calculated using RH value. 2.15.4. DRIFT VELOCITY(vd): According to the equilibrium condition, FE = FL, we have = ∴ = --------------- (12) Drift velocity can be calculated by using Hall voltage VH & magnetic field B and width of the wafer w. 26 Advanced Engineering Materials 2.15.5. HALL ANGLEθH: The net electric field E in the semiconductor is a vector sum of Ex and EH. it acts at an angle θH to the x-axis, where θHis called as Hall angle. tan = ------------------ (13) But = = and = since = Thus, eqn. (12)becomes tan = or tan = 1 1 But = = ∴ tan = --------------- (14) or tan = as = --------------- (15) QUE: Explain the phenomenon of Hall Effect and obtain an expression of Hall voltage developed in rectangular specimen of conductor at equilibrium.(4)[Winter-13] QUE: Explain the induction of hall Voltage in a semiconductor carrying current and placed in transverse magnetic field. Also obtain an expression for Hall Coefficient. (4)[Summer-14] QUE: What is Hall Effect? Obtain an expression for Hall coefficient. (4) [Winter-14] QUE: What is Hall Effect? Derive the relation for Hall voltage and Hall coefficient. (4)[Summer -15] QUE: Derive the expression for Hall voltage and Hall coefficient for extrinsic semiconductor. (4) [Winter-15,Summer-19] QUE:What is Hall Effect? Obtain an expression for Hall coefficient for a specimen having only one type of charge carriers. (4)[Summer-16] QUE: Explain the formation of Hall voltage developed in an extrinsic semiconductor and obtain the expression for Hall coefficient. (4)[Winter-16] QUE: What is Hall Effect? Obtain an expression for Hall voltage if p type semiconductor material is used. (2+3)[Summer-17,Winter-18] QUE: What is Hall Effect? With a well labeled diagram obtain an expression for Hall coefficient for an extrinsic semiconductor. (4) [Winter- 08,09 &17, Summer-08] QUE: Explain the phenomenon of Hall Effect and obtain an expression for Hall voltage for an extrinsic semiconductor. (4) [Summer-18, Winter-06, 07] QUE: Explain Hall Effect. Obtain an expression for Hall coefficient and Hall mobility in case of extrinsic semiconductor. (6) [Summer-11, 12] QUE: What is Hall Effect? Derive the relation for Hall voltage and Hall coefficient. (5) [Winter-12] 2.15.6. THE IMPORTANCE OF HALL EFFECT In the field of semiconductor Hall effect helps 1. To determine the drift velocity of charge carriers. 27 Advanced Engineering Materials 2. To determine the sign of charge carriers. 3. To determine the charge carrier concentration. 4. To determine the mobility of charge carriers if conductivity of the material is known. 5. To measure variable magnetic fields up to frequency of 1012Hz. 6. To measure extremely heavy currents, where conventional ammeter cannot be used. 2.15.7. EFFECT OF TEMPERATURE ON HALL COEFFICIENT In metals, Hall coefficient does not depend on temperature. Hence concentration of free electrons does not vary with temperature. In semiconductors, Hall coefficient decreases with the rise in temperature. Thus, the concentration of free electrons increases with temperature. 1.14.8. SIGNIFICANCE OF HALL EFFECT Hall Effect experiment showed that it is the negative charge carriers namely electrons are responsible for electrical conduction in metals. It also showed that there exist two types of charge carriers in semiconductors. QUE : Explain Hall effect and its significance. Give its application. (5)[Summer-07] QUE : What is Hall effect ? What are its applications? What is the effect of temperature on Hall coefficient for semiconductors? (6) [Summer-09] QUE : Explain Hall effect and its importance. (3)[Summer-05, Winter-11] List of formulae: 1.Hall voltage VH = RH = where ‘t’ = thickness of sample. 2.Hall coefficient RH = − for N-Type and RH = forP-Type. where ‘n’ or ‘p’ = Electron or hole (carrier) concentration. 3.Drift velocity = E or 4.Drift velocity = 5. Hall mobility = RH σ and w= width of the sample. 6. Hall Angle = tan ( ). 7.Current gain (CB mode) α =. 8. Current gain (CE mode) β =. 9.Emitter current = +. 10. Collector current, IC = Solved Problems: 1. In Hall coefficient experiment a current of 0.25 A (2.5mA) is sent through a metal strip having thickness 0.2 mm and width 5 mm. The Hall voltage is found to be 0.15 mV when a 28 Advanced Engineering Materials magnetic field of 2000 Gauss or 0.2 T is used. Calculate (a) carrier concentration (b) drift velocity of the carriers. (4)[Summer- 03,07,08,10 &13] Ans. Given: I = 0.25A, thickness t= 0.2mm= 0.2×10-3m, w= 5mm= 5×10-3m, VH = 0.15mV=0.15×10-3V. B =2000Gauss = 2000×10-4T. n=? and =? × ×. Solution: VH= = ×. × ×. × × ×. or 0.15 × 10 = ×. × ×. × ×. Hence Carrier concentration ‘n’ = = 1.04 × 10 /. × ×. × ×. ×. × Drift velocity = = = 0.15m/s. × × × 2.The resistivity of doped silicon crystal is 9.27 ×10-3 ohm-m and Hall coefficient is 3.84 ×10-4 m3/C. Assuming that the conduction is by a single type of charge carrier, calculate the density (carrier concentration) and mobility of carriers. (4)[Winter-09,12 &17, Summer-09] Ans. Given: Resistivity ρ = 9.27 ×10-3 ohm-m, Hall coefficient RH = -3.84×10-4 m3 /C, Density (carrier concentration) n =? Mobility of carriers μ=? Solution: Density (carrier concentration) = n= = = 1.6 × 1022/m3.. × ×. ×. × = Hall mobility = RH σ = = = 0.0414 m2 /V.s.. × 3. A specimen of a semiconductor is 1mm thick and 1cm wide. A magnetic flux density of 0.5 T is applied parallel to 1mm edge and Hall voltage contacts are attached to measure the voltage across the width of the sample. The current flowing length –wise through the sample is 10mA.If the Hall coefficient of the material is 3.66×10-4m3 /C, compute the voltage between the Hall contacts. ii) If the resistivity of the material is 8.93×10-3Ω.m, find the Hall angle. Ans. Given: Thickness‘t’= 1mm =10-3m, width ‘w’=1cm=0.01m, B=0.5T, I =10mA= 10×10-3A, RH = 3.66×10-4m3/C, ρ= 8.93×10-3Ω.m, find Hall voltage. × × Solution: VH = RH = 3.66×10-4 = 1.86×10-4 volts. θ = tan (μ B) = tan ( ) = tan (3.66 × 10 × × 0.5). × = tan (0.0205) =1.17o 4. An electric field of 100 V/m is applied to a sample of n-type semiconductor whose Hall coefficient is -0.0125 m3/C. determine the current density in the sample assuming e = 0.36 m2 /Vs. (3)[Winter-06] 29 Advanced Engineering Materials Ans. Given: E=100V/m, RH = 0.0125 m3/C, e = 0.36 m2 /V.s Find J.. × Solution: Current density J = σE = = = 2880A/m2.. 5. A specimen of a semiconductor is 1mm thick is placed in transverse magnetic field of 0.5T. If the current flowing through the sample is 10mA, find (i) Hall angle(ii)Hall voltage if the Hall coefficient of the material is 3.66x10-4 m3/C and the resistivity of the material is 8.93x10- 3 Ω. Ans. Given: t = 1 mm= 10-3m w = 1 mm = 10-3 m B = 0.5 T I = 10 mA = 10 x10-3A RH = 3.66x10-4 m3/C ρ = 8.93x 10-3 Ω-m. VH=? θH=?. × Solution:VH = RH = 3.66×10-4 = 1.83mV. θ = tan (μ B) = tan ( ) = tan (3.66 × 10 × × 0.5). × = tan (0.0205) =1.17o 6. A semiconducting plate of thickness 2mm is placed in a magnetic field of 0.6 wb/m2 normal to its thickness. If the current of value 1.5x10-2A flows along its length, calculate the Hall voltage if the Hall coefficient is 3.6x10-4 m3C-1. Ans. Given: t = 2 mm= 2x10-3m B = 0.6 wb/m2 I = 1.5x10-2A RH = 3.6 x10-4 m3/C VH=?. ×. × Solution: VH = RH = 3.6×10-4 = 1.62 mV. × 7.A semiconductor of width 4 mm is subjected to transverse magnetic field. The drift velocity of electrons under this magnetic field is 3x103 m/sec. Calculate Hall voltage, if the applied magnetic field is 4 Wb/m2. Ans. Given: w=4mm=4 x10-3 m vd=3 x103m B= 4 Wb/m2 : = = 4 × 3 × 10 × 4 × 10 = 48 V 30 Advanced Engineering Materials 8. The Hall coefficient of certain silicon specimen is found to be -7.5×10-5 m3/C at a certain temperature. If the conductivity is found to be 200mho/m, calculate density of charge carriers and their mobility. (3M, W-2013) Ans. Given: Hall coefficient RH = -7.5×10-5 m3/C, Conductivity = 200mho/m. (1) n=? (2) μ =? (1) RH = − or n = − =− = 8.32×1022/m3. (. × ×. × (2) = Hall mobility = RH σ = 7.5 × 10 ×200mho/m = 0.015 m2/V.s ___________________________________________________________________________ 9. N-type germanium sample has a donor density of 1021 / m3. It is arranged in a Hall experiment having magnetic field of 0.5 T and the current density is 500 A/m2. Find the Hall voltage if the sample is 3 mm wide. (3M, S-2014) Ans. Given: n = donor density of 1021 / m3, B= 0.5T, Current density ‘J’= 500 A/m2, width of sample ‘w’= 3mm = 3×10-3m. Hall Voltage VH =? RH = = = = or = × × ×. = ×. × = 4.68×10-3Volts= 4.68mV 10.Calculate the value of Hall angle for a given semiconductor from given data. RH = 3.66 ×10-4 m3/C, ρ = 8.3 ×10-3 Ω-m, B= 0.5 Wb/m2. (2M, W-2012) Ans.: Given: RH = 3.66 ×10-4 m3 /C, Ρ = 8.3 ×10-3 Ω-m, B= 0.5 Wb/m2. Hall angleθ =? Hall angle θ = tan (μ B) 1 = tan ( ) ρ = tan (3.66 × 10 ×. × × 0.5) = tan (0.02205) =1.263o 31 Advanced Engineering Materials 11. Find d.c. current gain for a PNP transistor in a common emitter mode if collector current is 2mA and base current is 20μA. (3M, W-2016) Ans. Given: Ic = 2mA, IB = 20μA, β =? Solution: β= = × = × = 100. 12. In an NPN transistor in a common base configuration emitter current is 2mA and base current is 20μA.What are the values of collector current and current gain? (3M, W-2011, 2017) Ans.: Given: IE = 2mA, IB = 20μA =20×10-3mA = 0.02mA, (1) IC =? (2) α =? Solution: (1) IC = IE -IB = (2-0.02) mA = 1.98 mA. (2) α = = = 0.99 13.When a diode is forward biased a current of 50 mA flows through it. When it is reverse biased the current drops to 20 nA. What is the ratio of forward to reverse current? Ans.: Given: Forward current, = 50 = 50 × 10 Reverse current, = 20 = 20 × 10 × Solution: = = 2.5 × 10 × 14. A transistor is connected in the CE configuration. The collector supplies voltage of 10V and the voltage drop across 500 ohms is 0.6V. If α = 0.96, find the (i) Collector-emitter current (ii) emitter current (iii) base current. Ans. Given: VCC = 10 V Voltage drops across collector VC = 0.6 V RC = 500 ohms Current gain = α = 0.96, (1) VCE =? (2) IE =? (3) IB =? Solution: i) VCE = VCC - VC = 10-0.6 = 9.4V ii) α = 32 Advanced Engineering Materials. Collector current, IC = = = 1.2. rIE = = = 1.25 mA. iii) IB = I E - IC = 1.25mA - 1.2 mA = 0.05mA. 15. For a transistor working as a common base amplifier, current gain is 0.96. If the emitter current is 7.2mA, then calculate the base current. Ans. Givenα = 0.96 IE = 7.2mA IB =? IB =? Solution: Current gain α = IC / IE Therefore IC = αIE = 0.96 x7.2 = 6.91 mA IB = IE – IC IB= 7.2 mA - 6.91 mA IB= 0.29 mA. 16. In a npn transistor circuit, the collector current is 15 mA. If 95% of the electrons emitted by the emitter reaches the collector, what is the base current? Ans. Given: Collector current, IC = 15 mA IC = 95% IE IB=? Solution: Since IC = 95% IE, IC = 0.95 IE =. Since IE = IB + IC Base current, IB = IE – I C IB = IE – IC =. − = − 15 = 0.79 mA. 17.The base current of a transistor is 105 μA and collector current is 2.05 mA. Determine the value of β, IE, and α. A change of 27 μA in the base current produces a change of 0.65 mA in the collector current. Find βa.c. Ans. Given: IB = 105 μA =105 × 10-6 A, IC = 2.05 mA = 2.05 × 10-3A (1) IE =? (2) α =? (3) β =? (4) βa.c.=? Solution: (1) IE = IB + IC = (105 × 10-6 +2.05 × 10-3 ) A = 2.155 × 10-3 A (2) α = IC / IE= 2.05 × 10-3A /2.155 × 10-3A = 0.95 (3) β = IC / IB = 2.05 × 10-3A /105 × 10-6 A =19.52 (4) ΔIB = 27μA = 27 × 10-6 A ΔIC = 0.65mA = 0.65 × 10-3 A β = ΔIC / ΔIB = 0.65 × 10-3 A/27 × 10-6 A =24.1 33 Advanced Engineering Materials 18.A transistor has current gain factor α = 0.95. the transistor is connected in common- emitter configuration. Calculate the change in collector current when the base current is changed by 0.1 mA. Ans. Given: α = 0.95 Change in base current ΔIB = 0.1 mA ∆ =? Solution: ΔIC = β ΔIB But, = ∴ ∆ = ∆ 0.95 ∆ = (0.1) = 1.9 1 − 0.95 Question Bank Q1. What is PN junction diode. Explain the characteristics of PN junction diode in forward and reverse bias mode. Q2. What do you understand by avalanche breakdown process in reverse bias PN junction diode? Q3. What is rectification? Q4. Explain how PN junction diode can be used as half wave rectifier. Q5. What is bridge wave rectifier? Explain its working. Q6. Explain the working of full wave rectifier. Q7. What is the advantage of bridge rectifier over full wave rectifier? Q8. How is half wave rectification different from full wave rectification, explain with the help of diagram. Q9. Draw circuit symbol of Zener diode. Q10. What is Zener diode. Explain its VI characteristics in forward and reverse bias. Q11. State the applications of Zener diode. Q12. What is Zener breakdown process? Explain. Q13. Write a note on LED. Q14.What are uses and applications of LED? Q15. Explain the construction and working of LED. Q16. Why Silicon and Germanium cannot be used in fabrication of LED. Q17. Draw V-I characteristics of photodiode. Explain dark current. Q18.What is a photodiode. Explain its working principle. Q19. What are the modes of operation of photodiode? Q20. State the applications of photo diode. Q21.Draw the circuit symbol of (1) Tunnel diode (2) Photodiode. Q22.What is tunnel diode. Explain its working. Q23.State the applications of tunnel diode. Q24. Write short notes on (a)Zener diode (b) Tunnel diode (c) LED (d) Photodiode Q25.How does a transistor work as amplifier in Common base mode? Q26. Define (1) current gain α (2) current gain β (3) Voltage gain Av. Q27.Define Voltage gain Av in Common emitter and common base mode of an amplifier. Q28. Give the relation between current gain α and β. Q29. Explain the formation of energy bands in solids on the basis of band theory of solids. Q.30 Distinguish between conductors, semiconductors and insulators. Q.31 Prove that the probability function is symmetrical about EF at all temperatures except zero. Q.32 Explain in brief the concept of Fermi energy. Derive an expression for Fermi energy in intrinsic semiconductor. 34 Advanced Engineering Materials Q.33 Define Fermi level. Q.34 Draw energy band diagram for N- type semiconductor at 0 K and T K. Q.35 Draw energy band diagram for P- type semiconductor at 0 K and T K. Q.35When donor impurities are added to a semiconductor, the concentration of holes decreases. Explain with reasons. Q.36 Distinguish between intrinsic and extrinsic semiconductors with suitable examples. Q.37 Explain the detailed mechanism of current conduction in N-type and P-type semiconductors. Q.38Explain Hall effect. Obtain an expression for Hall coefficient and density of charge carriers for a P- type semiconductor. Q.39 Explain the formation of potential barrier across the junction region of semiconductor diode. Q.40 Explain the concept of diffusion current and drift current in a P-N junction diode. Q.41 Draw the energy band diagram for symmetrically doped P-N junction when it is (i) Forward biased (ii) Reverse biased. Q.42 Explain why in a transistor: (i) Base is thin & lightly doped (ii) Collector region has large area (iii) Emitter is heavily doped. Q.43 Explain the action NPN and PNP transistor when operated in Common base mode. Q.44 Explain the action NPN and PNP transistor when operated in Common emitter mode. Q. 45 Explain the input and output characteristics of NPN transistor when operated in Common base mode. Q.46 Explain the input and output characteristics of NPN transistor when operated in Common emitter mode. Q.47 What is current gain in a transistor? Derive its relation for common base mode and common emitter mode transistor. Q.48 What is tunnel diode, draw and explain its V-I characteristics. Q.49 What is Zener diode, draw and explain its V-I characteristics. Q.50 What is LED? Which factor decides the colour emitted out of it. Draw and explain its V-I characteristics. Q.51. A strip of n-type germanium semiconductor of width 1mm and thickness 1mm has a Hall coefficient 102m3/C.If magnetic field used is 0.1T and current through the sample is 1mA, determine the Hall voltage produced and also find carriers concentration of electron. (3M Winter-2015, Summer-2019) (Ans. VH = 1mV, concentration of electron = n = 6.24X1016 /m3.) Q.52. In Hall coefficient experiment a current of 2.5mA is sent through a metal strip having thickness 0.2 mm and width 5 mm. The Hall voltage is found to be 0.15 mV when a magnetic field of 200 Gauss is used. Calculate (a) carrier concentration (b) drift velocity of the carriers. (3M, Summer-2016)(Ans. n= 1.04×1022/m3, vd = 1.5m/s.) Q.53.Calculate the value of Hall voltage if observed Hall coefficient 5m3/C for a current of 1mA flowing through a specimen of 20 mm thickness placed suitably in a magnetic field of flux density 1.0 Wb/m2. (3M, Summer-2018) (Ans. VH =0.01 V) Q.54. If the emitter current is 6 mA and collector current is 5.75mA, calculate the value of D.C. current gain in common base mode. (3M, Summer-2018) (Ans. α = 0.958) Q.55. In a common base connection, current amplification factor is 0.9. If the emitter current is 1mA, determine the value of base current. (Ans.0.1 mA) Q.34.In a common base configuration, IC = 0.95 mA and IB = 0.05 mA. Find the value of α. (Ans. 0.95) Q.56.In a common base configuration, α = 0.95. The voltage drops across 2 kΩ resistance which is connected in the collector is 2V. Find the base current. (Ans. 0.05 mA) Q.57.Calculate IE in a transistor for which β = 50 and IB = 20 μA. (Ans.1.02 mA) 35