Earth Science: Nebular Hypothesis, Plate Tectonics & More
Document Details
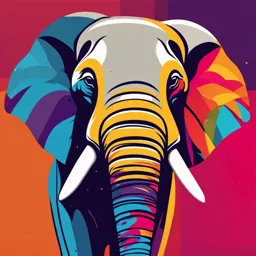
Uploaded by LuxuryJubilation9972
Tags
Summary
This module discusses the nebular hypothesis, formation of the lithosphere, Plate Tectonics Theory, and the origins of the Earth's atmosphere and hydrosphere. It covers topics such as elemental distribution, differentiation within protoplanets, continental drift, and the evolution of the atmosphere and hydrosphere.
Full Transcript
Nebular hypothesis Recall that the nebular hypothesis states that a swirling cloud of gas and dust provided the materials for the formation of the protoplanets, which are the “seeds” from which the main planets of the solar system came to be. You also learned that elemental distribution is a functi...
Nebular hypothesis Recall that the nebular hypothesis states that a swirling cloud of gas and dust provided the materials for the formation of the protoplanets, which are the “seeds” from which the main planets of the solar system came to be. You also learned that elemental distribution is a function of the distance of a planet from the sun, with rocky, denser terrestrial planets being nearer to the sun, and large, less dense jovian planets being farther from it. As the protoplanets grew larger, their gravitational force also strengthened, and they became denser as heavier elements sank towards the center of each protoplanet. Differentiation describes the segregation of elements within a protoplanet. The processes of accretion and differentiation led to the creation of the geosphere. Nebular hypothesis (recall this from Module 3) is the most widely accepted explanation of the formation of the solar system. This hypothesis proposes the simultaneous origin of the sun and the planets, unlike earlier notions stating that the planets are derived from materials from a preexisting sun (Dott and Prothero, 2004). Although distance from the sun is one factor in elemental availability, we must note that elemental segregation does not fully segregate the elements such that light elements are found only in the jovian planets and heavy elements, such as iron and nickel, are found only in the terrestrial planets. Chemical theory suggests that heavy elements decrease in abundance as distance from the sun increases. This is governed by the temperature of the solar nebula, as most of the heavy elements condense in hotter environments. The density of the Earth is greater than the density of the rocks found in the crust. Therefore, there should be heavier materials inside the Earth. This led to the speculation that the Earth’s interior is zoned. Further evidence of the Earth’s internal structure is presented by differences in the behavior of waves as they travel through the Earth, and the existence of the magnetic field. The compatibility of elements in certain phases determines the components available for each zone in the Earth’s layers. The differentiation of the Earth’s compositional layers allowed the segregation of elements and thus influenced the availability of materials on the surface. As gravitational strength increases near the center, the behavior of the Earth’s layers also differs even if some of these zones are of similar composition. Thus, the interior of the Earth is classified based on its composition and its mechanical properties. Formation of lithosphere We now focus on the uppermost mechanical layer of the Earth which includes the crust and the uppermost mantle – the lithosphere. The mantle is relatively heavier in composition (magnesium and iron) compared to the crust and is further differentiated based on its mechanical property. The upper mantle is more ductile in nature and can flow; the crust “rides” on top of the uppermost mantle. The crust is further divided into two based on composition – the continental and oceanic crust. Their composition has implications on the density and distribution of the two kinds of crusts on the lithosphere. You may have noticed that the discussions concerning the lithosphere focus mainly on the Earth’s crust rather than the whole lithosphere. It is the crust that is the most affected by the surface dynamics of the Earth. The segregation of the more brittle crust and the more ductile upper mantle is discussed by Dott and Prothero (2004) in terms of chemical differentiation. The relatively lighter elements (e.g., oxygen, silicon, aluminum) are retained in the continental crust while the heavier elements (e.g., iron, magnesium) are concentrated in the oceanic crust. Condie (1989) presented three models to explain the origin of the Earth’s crust. The inhomogeneous model suggests that the last elements to condense in the solar nebula were the lightest, which may have produced the first crust rich in silicon, oxygen, and aluminum. Although this model adheres to the notion of chemical differentiation of the elements, the model states that the crust was formed from the condensation of the solar nebula. The impact model proposes that asteroids with a similar composition to the crust produced the first continents, with the impact creating intense heat that led to the melting of the Earth’s crust, which resulted in the production of both oceanic and continental crusts. But while it is true that innumerable asteroids have plummeted towards the Earth’s surface, clues from the rocks in the Earth’s moon suggest that basalt, a key component of the Earth’s oceanic crust, formed on the surface of the moon after the impacts. The exponential increase in pressure for a very short time caused by impacts from asteroids should result in a peculiar form of the minerals exposed to the event – however, this is absent in rocks of similar age. Finally, the terrestrial model points to processes that operate within the Earth, which corroborates Dott and Prothero’s (2004) discussion of the formation of the crust. Our understanding of the first hundred million years of the Earth’s history is speculative and based on limited evidence. Only isolated exposures of Hadean (~4.60 – 4.00 billion years old) rocks have ever been found, and most information is obtained through the study of undifferentiated meteorites. When the geosphere and lithosphere were formed cannot be determined exactly, which is why the mechanisms of their formation are subject to much research and debate. Current models will surely be improved as more data become available through scientific investigation. Continental drift and seafloor spreading You may have learned in your earth science class in high school that the dynamics occurring in the Earth’s crust is primarily due to plate tectonics. Plate Tectonics Theory includes the movement of the continents, creation of mountain ranges, and occurrence of hazards such as volcanic eruptions and earthquakes. Although this theory is now called the “unifying theme of geology”, earlier works that bolstered the idea of plate tectonics were faced with great skepticism. Early ideas on surface dynamics came from observations that igneous rocks are usually found parallel to the axis of mountain ranges. Scientists like James Hutton and Leopold von Buch believed a so-called vertical force pushed the rocks from the interior. Others argued that the contraction of the crust caused folding and the creation of mountains, which are horizontal forces. However, two of the most important concepts in laying the foundation for plate tectonics are the Continental Drift Theory and the Seafloor Spreading Theory by Alfred Wegener and Harry Hammond Hess, respectively. We will take a close look at these two theories. Wegener’s Continental Drift Theory explained several phenomena that puzzled scientists during his time, such as (1) the fit of the South American and African coastlines; (2) the presence of identical fossils across continents; (3) the presence of similar mountain ranges across continents; and (4) the presence of coal deposits near the poles (which are otherwise cold places) which is based on past climate data. Wegener proposed that all of the continents were joined into one single landmass, which he called Pangea, and the movement of the continents plowed against oceans. Some of Wegener’s suggestions for explaining the movement of the continents include tidal friction and the Earth’s rotation. Although the exact mechanisms for these drifts were not yet understood at that time (as acknowledged by Wegener himself), he presented unequivocal evidence suggesting that continents were probably once joined together in the past and they subsequently drifted to their present-day location, which resulted in such features as mountain ranges and mid-ocean ridges, among others. However, despite the evidence, the mechanisms proposed by Wegener were rejected by physicists because these forces were thought to be too small/weak to drive crustal movement. It was only in the 1960s that seafloor exploration began, providing new data on the oceanic crust. Hess (1962) published a paper proposing the mechanism for continental drift that Wegener failed to provide in his paper, namely, seafloor spreading. Instead of continents plowing through the oceanic crust, it was proposed that the continents “rode passively on a convecting mantle”. In Hess’s paper, mid-ocean ridges are postulated as areas representing the rising limbs of mantle convection cells, the area where mantle materials come to the surface, pushing the continents away from each other at a particular rate. This is supported by the age and thickness of the sediments on top of the ridge, which become older and thicker towards the edge of the basins. This contrasts with the circum-Pacific region, which represents the descending limbs of the convection cell, and where deformation and volcanism are prevalent. The relatively thin cover sediments on the seafloor, the absence of rocks older than the Cretaceous period, and the relatively small number of seamounts suggest a young seafloor, contrary to the idea of “ocean permanence,” which was also addressed by Wegener (1912; 2002). Plate tectonics Since the publication of the papers by Wegener (1912; 2002) and Hess (1962), numerous studies have provided compelling evidence of continental drift and sea floor spreading, now collectively known as the Plate Tectonics Theory. Geomagnetism, the study of the Earth’s magnetic field, provides support for the idea of magma generation along mid-ocean ridges as predicted by Hess. The simplified illustration in Figure 17 shows how magnetic imprints are developed along mid-ocean ridges. As the magnetic field of the Earth reverses in direction (i.e., the magnetic north is transferred to the south), minerals within the rocks will align themselves to that field. It has been shown that these magnetic reversals are strikingly symmetrical (albeit not perfect) parallel to the mid-ocean ridge axis, providing strong evidence that magma generation does happen along these ridges. Figure 17. Magnetic Reversals Recorded on the Seafloor Seismology, the study of earthquakes, provides information on constraints to the geometry of the plates as well as the Earth’s internal structure. Conspicuous concentrations of seismic activities are manifested along trenches and mountain ranges. The Plate Tectonics Theory suggests that these are zones of plate collision causing some crust to subduct beneath another or to be deformed intensely and rise in height. Finally, the development of the Global Positioning System (GPS) paved the way for a more precise documentation and measurement of plate motion and their internal deformation. These methods solidified the status of Plate Tectonics Theory as one of the most important ideas in geology, which will be improved and refined as more data becomes available. The Plate Tectonics Theory describes the creation, motion, and destruction of the upper most portions of the Earth. It is also a good example of how a scientific method works – i.e., Plate Tectonics Theory is a revolutionary idea in geology that synthesized earlier hypotheses regarding the mechanisms for large-scale movements of continents and the formation of ocean basins through seafloor spreading, and it now provides the framework within which geologic phenomena like earthquakes and volcanic eruptions can be understood. Origins of atmosphere and hydrosphere As you know, water exists in other planetary bodies within our solar system but in the solid phase (ice) or the gaseous phase (water vapor). It is only on Earth where water exists in liquid form and this is because of the Earth’s distance from the sun. This module discusses the origins of the Earth’s oceans which, together with lakes, rivers, and streams, comprise the hydrosphere. The module also discusses where the Earth’s atmosphere came from. More specifically, we will learn about the early condition of the hydrosphere and atmosphere and how they changed through the early history of the Earth. As you go through the module, you will realize that most of the changes in the atmosphere and hydrosphere happened in the “young” Earth and they stabilized afterwards. Be sure to read the key text or required reading and answer the study questions to maximize your learning through discussions. Early Atmospheric and Hydrospheric Composition and Climate As you know, water exists in other planetary bodies within our solar system but in the solid phase (ice) or the gaseous phase (water vapor). It is only on Earth where water exists in liquid form and this is because of the Earth’s distance from the sun. This module discusses the origins of the Earth’s oceans which, together with lakes, rivers, and streams, comprise the hydrosphere. The module also discusses where the Earth’s atmosphere came from. More specifically, we will learn about the early condition of the hydrosphere and atmosphere and how they changed through the early history of the Earth. As you go through the module, you will realize that most of the changes in the atmosphere and hydrosphere happened in the “young” Earth and they stabilized afterwards. Be sure to read the key text or required reading and answer the study questions to maximize your learning through discussions. The Rise of Oxygen and the Effect of Life on the Atmosphere The evolution of the atmosphere was not only affected by the geosphere and extraterrestrial influences, but also shaped by the emergence and proliferation of life. Oxygen was not immediately able to increase in concentration because of its incorporation in mineral and rock phases (see Banded Iron Formation – BIF). Gradual evolution of life from 3.5 to 2.5 billion years ago must have brought about changes in the metabolism of microorganisms, which later acquired the ability to perform photosynthesis. Free oxygen began to accumulate at the end of the Archean Eon (2.5 billion years ago), coinciding with the abundance of photosynthetic bacteria. Hence, this discussion intersects heavily with discussions about the origin of life. It is difficult to separate the evolution of life from the formation of the atmosphere because organisms profoundly influenced changes in the composition of the air that we are now breathing. As Decker and van Holde (2011) pointed out, we owe our existence to cyanobacteria, the first photosynthesizers; they produced the free oxygen in the atmosphere and hydrosphere today. We also learned that the effect of the biosphere on the Earth is enough to cause radical changes not only in the composition of the atmosphere and hydrosphere but also in the shape of the landscape itself. The increase of oxygen was not straightforward as abundant oxidants in the oceans (such as free iron ions) were ready to capture the oxygen created by the cyanobacteria. This can be correlated to the start of the rise in oxygen levels (Stage I) as discussed by Kasting (1993). He describes Stage I as a rarity of early Archean red beds, which implies that oxygen was present only in small amounts. You may think of these “red beds” as rusted rocks, reacting with the iron content in the material to form iron oxide. Thus, the abundance of BIFs may be related to increased production of oxygen, causing more iron to be oxidized. It was not until the free iron ions were depleted that oxygen began to increase in concentration. This is correlated to Stage II of Kasting’s (1993) notion of oxygen evolution. As the amount of oxygen increased, major changes in the biota and chemistry of the oceans and atmosphere were recorded. It was not until this increase of oxygen that multicellular life evolved. This is the final stage of the oxygen evolution (Stage III) as described by Kasting (1993). In effect, the availability of certain ions changed because of the introduction of free oxygen to the system. Notably, the early and pioneering works were in conjunction with modern studies regarding the formation of the atmosphere and hydrosphere. Origin of the Biosphere According to Vladimir Vernasky as cited by Levchenko et al. (2012), “biosphere is a specific envelope of the Earth, comprising the totality of all living organisms and that part of planet matter which is in constant material exchange with these organisms. Virtually, it is a spherical layer 6 – 12 km thick.” In simpler terms therefore, the biosphere is a narrow zone of the earth in which all three essentials required for sustenance of life are found: land (lithosphere), air (atmosphere) and water (hydrosphere), which were the topics in Modules 7 and 8. Scientists believe that the study on the “origin of living organisms and biosphere are two aspects of the same process” (Levchenko and Starobogatov, 2010). This means that the conditions on the early Earth have guaranteed the origin and survivorship of organic life. In addition, the primary organisms incorporated in natural geological processes, have accelerated and transformed surroundings in directions favorable for the creation of higher forms of life (Levchenko et al., 2012). This module has provided an overview of how the atmosphere and hydrosphere might have been formed. The discussion from Decker and van Holde (2011) suggests that a combination of factors contributed to the emergence of these two spheres. You will notice that most of the discussion tended to be more about the formation of the atmosphere than the formation of the hydrosphere. This is because we have a clearer picture of the dynamics of change in the Earth’s atmosphere. Furthermore, it is very difficult to pinpoint when water appeared on the Earth’s surface. Our understanding of the first hundred million years of the Earth’s history is speculative and based on limited evidence. Only isolated exposures of Hadean rocks have ever been found, and most of the information on hand has been obtained through the study of undifferentiated meteorites. When exactly the nonliving spheres were formed cannot be pinpointed, and the mechanisms of their formation are the subject of much research and debate. The current models will be improved as more data become available through scientific investigations. To conclude, the formation of the non-living spheres provided the conditions for life to emerge. We reiterate, however, that life, for which the atmosphere, hydrosphere, and geosphere are prerequisites, influences to a significant degree the composition and shape of the non-living spheres of the Earth.