Rockets PDF
Document Details
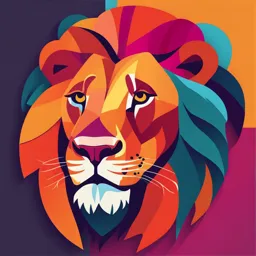
Uploaded by SustainableEllipse5299
Tags
Summary
This document provides a detailed exploration of rocket technology, covering its history, types, and the principles behind rocket propulsion. It includes insights into the evolution of rockets from ancient times to modern applications in space exploration.
Full Transcript
1 Space Technology: Chapter 1 – Rockets | 1 ROCKETS Rocket in simple words is a type of vehicle which is used to take spacecraft to space by producing huge amount of thrust. The principles of rocketry were tested more than 2,000 years ago, but it's...
1 Space Technology: Chapter 1 – Rockets | 1 ROCKETS Rocket in simple words is a type of vehicle which is used to take spacecraft to space by producing huge amount of thrust. The principles of rocketry were tested more than 2,000 years ago, but it's only been used in the past 70 years for space exploration. Earlier rockets were used for war but today, rockets take satellites to space, astronauts to the International Space Station (ISS), carry supplies to ISS, land on their own and are proposed to be reused. Rockets routinely take spacecraft to the Moon and other planets in our Solar system. Here, we will learn how Rockets' concept evolved over the time and get to know about a few of the brightest minds behind the making of rockets. We will also go through a few of the missions which paved the way to a whole new world and enhanced the capabilities of humankind. Rockets in History: One of the early devices that used the rocket propulsion principle was a small bird-shaped apparatus. The bird's belly had a boiler setup that would heat the water and convert it into steam. The steam would escape from the nozzle at the rear end, which would propel the bird. Archytas (428 - Fig. 1: The steam-powered pigeon of 347 BC), a Greek philosopher, mathematician, and Archytas, the flying machine astronomer, was said to have constructed and of antiquity. (Credits: Reese, M. R. 2014) flown this device. Another device invented by Hero of Alexandria used the principle of rocket propulsion. Aeolipile, also known as Hero's engine, consisted of a metal sphere mounted on top of a water kettle. A fire underneath the kettle heated the water, and the steam produced would travel through the tubes and escape in opposite directions. The rapidly escaping jet of steam produces a force on the sphere, which caused it to rotate. Fig. 2: Illustration of Hero’s Engine Another important invention is gunpowder. The origin of gunpowder is unknown. However, based on scriptures, historians suggest that the Chinese used a mixture of saltpetre, sulphur and charcoal for their rituals. The mixtures produced sparks and bright smoke when ignited. Bamboo tubes were filled with this black mixture and sealed at both ends. The tubes would then be tossed into the flame. The tubes would explode with a bright flash of light and a loud noise, which was part of their ritual. Sometimes, one of the sealed ends would rupture, and instead of an explosion, the hot gases escaped through the open end, thereby sending the tube into the sky. Observing this phenomenon, the Fig. 3: The oldest known Chinese attached these bamboo tubes to arrows and were used against depiction of rocket arrows, the Mongols in the battle of Kai-Keng in 1232 AD. Thus, the rocket from the Huolongjing. was born. 2 Space Technology: Chapter 1 – Rockets | 2 Birth of Modern Rocketry: In the 17th century, Sir Isaac Newton laid the foundations of modern rocketry. Newton published his understanding of the physical motion of objects in the form of three scientific laws that could explain the motion of everyday objects, including rockets. During the 18th and early 19th centuries, the rockets that had been used as a weapon of war had an upgrade. In 1792, Tippu Sultan used rockets against the British during the Anglo-Mysore wars. The rocket as a weapon was destructive not because of its accuracy but of its sheer number. This caught the interest of an artillery expert, Colonel William Congreve. Later the British re-designed rockets for their military and used this to be successful in their battles. The technology of rockets spread across the Fig. 4: Painting of 1780-Battle of Guntur, world as a weapon of choice, yet the idea of using Confrontation of East India company with rockets for space exploration was far ahead. Tippu Sultan's Mysorean rockets. (Credits: Charles Hubbell) Between 1880 and 1930, the interest in using rockets for space exploration grew among the public. Inspired by the fiction writers such as Jules Verne, the Russian school teacher Konstantin E. Tsiolkovsky proposed the idea of space travel using rockets. In a report published in 1903, he suggested using liquid propellants for rockets to improve their range. He also stated that the speed and range of a rocket were limited only by the exhaust velocity of escaping gases. This was a revolutionary idea and helped would be in the coming decades and would help other pioneers in the field to improve rocket Fig. 5: Konstantin Tsiolkovsky (right) is one of designs. the founding fathers of rocketry and space travel. (Credits: Science Photo Library) Robert H. Goddard, an American Scientist, followed the works of Konstantin Tsiolkovsky and conducted various experiments from 1908 to 1945. In 1915, he began experimenting with various types of solid fuels to increase the exhaust velocities of the burning gases. With the results he obtained while working on solid-propellant rockets, Goddard became convinced that a rocket could be propelled better by liquid fuel. His works included the development of the world's first liquid propellant rocket in 1926. The rocket-powered by liquid oxygen and gasoline, flew for just two and a half seconds, climbing Fig. 6: Robert H. Goddard with 12.5 metres, and landed 56 metres away in a cabbage patch. his first liquid rocket engine. Even though by today's standards, the flight was unimpressive, it paved the way for other pioneers in the field to contribute to the rocket design. 3 Space Technology: Chapter 1 – Rockets | 3 Hermann Oberth of Germany also made equal contributions to the theory and design of rockets. Hermann played a vital role in advancing the practical application of rocket propulsion that led to the development of rockets in Germany during the 1930s. Due to these pioneers' contributions and many others in unwritten history, the evolution of rocket design took a leap during the Second World War. The most notable achievements in rocket technology happened in Germany. On October 3, 1942, German rocket scientist Wernher von Braun, a student of Hermann Oberth, developed and launched the V-2 rocket from Peenemunde, an island off Germany's Baltic coast. The V-2 rocket was one of the most advanced of its time. Even though the technological leap was huge from the Chinese era black powder rockets to the most advanced liquid fuel V-2 rockets, people still used them for war applications. Fig. 7: A German V-2 rocket ready for launching at Cuxhaven in Luneburg district, Lower Saxony (1945). By the end of the Second World War and the fall of Nazi Germany, many unused V-2 rockets and their components were captured by the Allies (mainly the United States). German Scientists, including Wernher von Braun, surrendered to the Allies and later played a vital role in developing American rockets. Missiles to Launch Vehicles: An Intercontinental Ballistic Missile (ICBM) is a missile capable of delivering nuclear warheads from one continent to another. The first and practical design of an ICBM came from the V-2 program of Germany. With the success of V-2 rockets and the fall of Nazi Germany, the need for an ICBM grew larger in fear of another World war. The capture of unused V-2 rockets from fallen Germany by its allies led to the faster development of the missiles. From 1954 to 1957, Soviet rocket designer Sergei Korolëv headed the development of the R- 7, an ICBM that would later be modified to launch Sputnik. Successfully flight tested in August 1957, the R-7 missile was powerful enough to launch a nuclear warhead or send a spacecraft into orbit. On October 4, 1957, the R7 missile was modified to launch the world's first human-made object to enter space, the Sputnik satellite. With the launch of Sputnik, our journey to space began. This event would be so pivotal in the history of humanity that the perspective of our existence in this vast expanse changed forever. 4 Space Technology: Chapter 1 – Rockets | 4 Fig. 8: (Left) A Soviet R-7 rocket lifts off from the Baikonur Cosmodrome in Kazakhstan, sending Sputnik into orbit and kicking off the space age. This view comes from a 1967 documentary film titled "Ten Years of the Space Age." (Right) Sputnik -1 Satellite This event led to an important period in the history of space exploration, the Space Race, between the U.S. and the Soviet Union. Following the launch of Sputnik, the United States launched their satellite, Explorer 1, on January 31, 1958; The same year, NASA came into existence. In 1961, the Soviets launched the first crewed spacecraft - Vostok, which carried cosmonaut Yuri Gagarin. Humans reaching space was not fiction anymore. Eventually, more countries were added to the list of space-faring nations. Fig. 9: Explorer 1 (Credits: NASA) Physics behind the Rockets: After understanding the brief history on evolution of Rocket Technology, let us now explore the fundamental principles behind the motion of a Rocket and its ability to lift off against Earth's gravity. Anatomy of a Rocket First, let us know the various parts and systems of a Rocket, then learn about them in the upcoming sections. Rockets that launch up into space comprise four significant systems (Fig. 10). They are: I. Structural System: This makes up the frame/body that holds the entire Rocket together. The cylindrical body comprising motor cases, inter structures, nose cones, nozzles, propellant tanks, heat shields, engines and other frames make up the structural system. The structural systems contribute to the total integrity of launch vehicle holding propellants and other subsystems. All the structures are fabricated and machined to the required accuracies. II. Propellants: This occupies the most amount volume of the Rocket and is responsible for providing the required force for the Rocket to lift off and travel against gravity. The solid propellants will be cast into the segments and stored before launch, whereas earth storable/liquid and cryogenic (cryo) propellants are filled into the stages before the launch. Cryo propellants are continuously cooled through chillers just before the launch. 5 Space Technology: Chapter 1 – Rockets | 5 III. Navigation, Guidance and Control system: Automatic inflight stabilising of the attitude, the angular orientation of the vehicle, and steering its path from launchpad to orbit injection constitute the functions of the control and Payload guidance system. The system enables its sensors to detect the instantaneous velocity and position of the vehicle and charts out the remaining path by continuously recalculating the vehicle attitude as well as the central NGC propulsion system. The navigation system is generally based on high precision acceleration, high speed, and high accuracy computations. IV. Payloads: Payloads are the objects carried by the Rockets and injected into the orbits to Structural derive the applications in space. Payloads such System as satellites, astronauts, spacecraft, and telescopes depend on the type of mission. Other satellites may contain different payloads Propellants depending on the application, such as optical, communication, navigation, scientific instruments, sensors, etc. For a Rocket to be launched into space, all these systems must work together in order to overcome the force of gravity and complete a mission. Fig. 10: Rocket Systems of GSLV MK-III Thrust and Propulsion To understand Thrust and propulsion in Rocketry, let us use the analogy of a balloon (Fig. 11a). An inflated balloon is a flexible chamber containing pressurised gas. The moment we release the pressurised gas through the opening, the gas rushes out, propelling the balloon in the opposite direction. Thrust is defined as the force acting along the longitudinal axis of the balloon, provided by exhaust gases to propel the balloon forward. Fig. 11a - Comparison of Balloon and 11b - Rocket propulsion 6 Space Technology: Chapter 1 – Rockets | 6 In the case of Rockets (Fig. 11b), the pressurised gas is produced by burning propellants inside the Rocket. Propellants are usually a combination of fuel and oxidisers that burn when ignited, paving the way to hot pressurised gases which exit the nozzle and generate the Thrust required to propel the Rocket. Newton’s Laws of Motion – Applied to a Rocket 1st Law: Objects at rest will stay at rest and objects in motion will stay in motion in a straight line unless acted upon by an unbalanced force. Applying the first law to a Rocket, we can say that: A Rocket standing on a launch pad is at rest and remains in the same state unless acted upon by the thrust produced due to the exhaust gases. We say that the Rocket is in a balanced state when it is on a launchpad, it is because the gravity acting on the Rocket is balanced by the force with which the launch pad pushes against the Rocket. Fig. 12: PSLV Rocket on Launch Pad Once the engines ignite and the Rocket lifts off, the thrust from the exhaust gases unbalances the Rocket and propels it forward from its initial state of rest. The Rocket continues to be in the state of motion until the propellants are completely utilized. Once the propellant is over, the Rocket loses its propelling force and hence stops from further increase in velocity. Fig. 13: PSLV Rocket Lift-off 2nd Law: Force is equal to mass times acceleration. (F = ma) Applying the second law to a Rocket, we can say that: The Thrust (F) produced by a Rocket engine is determined by the mass of the burnt propellant (m) and the acceleration of the exhaust gases (a) F exiting the nozzle. m a Another way of interpreting the 2nd law is by saying that Force is equal to the rate of change of momentum. This interpretation will make us understand the Rocket Thrust equation discussed further in this section. 3rd Law: For every action there is always an opposite and equal reaction 7 Space Technology: Chapter 1 – Rockets | 7 This is the most important law in Rocketry. Applying the third law to a Rocket (Fig.14), we can say that: For the action of hot pressurised gases expelling from the Rocket, the reaction is the Rocket lifting off to space. In our case, we have a Rocket that we want to launch into space. The third law tells us that if we can get the Rocket to push against the ground with enormous force, then the ground will respond by pushing the Rocket upwards with Fig. 14: PSLV Rocket Lift-off an equivalent amount of force. (Action and Reaction) Conservation of Momentum in a Rocket (Time for some Math!) The law of conservation of Momentum happens to be the outlying principle behind Rocket propulsion. The law states that: “For two or more bodies in an isolated system acting upon each other, their total momentum remains constant unless an external force is applied”. This law can also be stated as: Net momentum before acting upon each other = Net momentum after acting upon each other The momentum of a body is defined as the product of mass (M) and velocity (V) of the body. i.e., Momentum = M x V. When we apply the law to a Rocket system, Consider, Body 1 = Propellants (Mass = Mp, Velocity = VP) Body 2 = Rocket (Mass = Mr, Velocity = Vr) Case 1: Before mass ejection Case 2: After mass ejection (Rocket is stationary) (Rocket is in motion) VP1 = 0 VP2 ≠ 0 Vr1 = 0 Vr2 ≠ 0 Net momentum (Pnet1) = Mp1 VP1 + Mr1 Vr1 Net momentum (Pnet2) = Mp2 VP2 + Mr2 Vr2 Since both the velocities are 0, Since both the velocities are finite, Pnet1 before mass ejection = 0 Pnet2 after mass ejection ≠ 0 According to the law, Pnet1 = Pnet2 Mp1 VP1 + Mr1 Vr1 = Mp2 VP2 + Mr2 Vr2 0 = Mp2 VP2 + Mr2 Vr2 Mr2 Vr2 = - Mp2 VP2 We can observe from the final equation that Vr2 and VP2 are in opposite directions (Negative symbol). This means the Rocket gets a net momentum equal to the momentum of the ejected propellants in the opposite direction which propels the Rocket upward. 8 Space Technology: Chapter 1 – Rockets | 8 Rocket Thrust Equation: The law of Conservation of momentum and Newton’s laws clarifies that the Thrust acting on a Rocket due to the ejected propellants, propels the Rocket upwards. In this section, let us derive an equation for the Thrust produced by a Rocket engine (Fig. 15). Ae Me V0 V M0 0 V0 Ve P0 Pe Fig. 15: Parameters involved in a Rocket thrust equation The exhaust gases and mass from the burnt propellants enter the nozzle with a momentum M0V0 and exit the nozzle with a momentum MeVe. From Newton’s 2nd law, force is defined as the rate of change of momentum. In this case, Thrust is our force. Therefore, we can write: 𝑴𝒆 𝑽𝒆 − 𝑴𝟎 𝑽𝟎 𝑻𝒉𝒓𝒖𝒔𝒕 (𝑻) = 𝑻𝟐 − 𝑻𝟏 T1 is the time when the exhaust gases enter the nozzle and T2 is the time when exhaust gases exit the nozzle. The thrust equation can be re-written as, 𝑻 = 𝒎̇𝒆 𝑽𝒆 − 𝒎̇𝟎 𝑽𝟎 Where, 𝑚̇𝑒 and 𝑚̇0 are the mass flow rates at exit and entry of the nozzle respectively. This thrust is obtained solely due to the rate of change of momentum of burnt propellants. Hence, it is defined as Momentum thrust. There is a second term in the thrust equation that is obtained due to the difference in pressures at the exit over the exit area. It is known as the Pressure thrust. Pressure Thrust = (Pe – Po) Ae Normally the magnitude of this Pressure Thrust is very less compared to the Momentum Thrust. Total Thrust = Momentum thrust + Pressure Thrust 𝑻𝒕𝒐𝒕 = 𝒎̇𝒆 𝑽𝒆 − 𝒎̇𝟎 𝑽𝟎 + (Pe – Po) Ae Question: 1. Calculate the total thrust of a Rocket if momentum thrust is 5350 kN and pressure thrust is 2470 kN. 2. If the total thrust produced by a PSLV Rocket is 4221 kN and the momentum thrust is 3995 kN, then calculate the pressure thrust produced by the Rocket. 9 Space Technology: Chapter 1 – Rockets | 9 The Trajectory of a Rocket The term “Trajectory” is widely used in discussions regarding Rocketry. It should not be misunderstood with “Path” as there is a subtle difference between both. A path is just the route taken by an object to move from point A to point B. In a trajectory, the time factor comes into the picture along with the route taken. It is defined as: A Trajectory is the path that an object in motion follows through space as a function of time. There are four important stages in a rocket’s trajectory (Fig. 16). 1. Launch 2. Powered Ascent 3. Staging 4. Cutoff The Launch begins when the Rocket's propulsion system generates a massive thrust. Once the Rocket generates more thrust than its weight, it lifts into the air to begin its Powered Ascent. During this phase of the flight, the Rocket's weight will constantly change as the fuel continues to burn off. As a result, most space-bound Rockets use Staging to reduce dead weight and increase efficiency. We will discuss staging more in the final section. As the Rocket continues into Orbit, its sophisticated guidance system maintains balance and steers to keep the flight trajectory on track. The upper stage engine Cuts off at the correct altitude and speed, completing the Rocket's journey from Earth's surface into Orbit. Fig. 16: Phases of Flight trajectory Stages: All the rockets produce Thrust, and it is generated by a propulsion system to overcome the rocket's weight and the Earth's gravitational force. The weight of the payload is a tiny portion of the total lift-off mass of a Rocket. Most of the mass is propellant (the fuel and oxidizer). Once the propellant is utilized, the empty storage tanks and engine are of no use, and they also add dead weight to the total weight of the rocket. Instead of carrying those empty propellant tanks and achieving a certain orbital velocity, we must reduce the rocket's weight; hence, we use the concept of stages. We divide the propellant's total mass into small segments and store it separately. As explained earlier, once the propellant from each stage is utilized, the small empty tanks and engines are detached and discarded from the rest of the rocket body. They fall into the ocean/water bodies. Once the first stage is detached, the next set of stage gets automatically initiated and works similarly until the payload is delivered to its intended orbit. There are two types of Rocket staging. Serial Staging - Stacking engines on one top of the other. Parallel Staging - Having the engine placed parallel to the other engine. 10 Space Technology: Chapter 1 – Rockets | 10 Stage 4 Stage 3 Serial Staging Stage 2 Parallel Staging Stage 1 Fig. 17: Types of Staging Payload of the Rocket: Payload technically means the object that has to be carried to space in a rocket. Based on the payload, we categorize the rockets as follows; I. Uncrewed Space Flight: A rocket capable of carrying a satellite, Spacecraft, cargo materials to ISS, Lander, Rover, etc., to space and inserted at the right orbit. II. Crewed Space Flight: A rocket capable and well equipped to provide the ideal conditions in order to take humans to space. The orbital module of Gaganyaan Fig. 18: (Left) GSLV Mk III with Chandrayaan 2 Lander as payload and (right) Human rated Launch vehicle for Gaganyaan Mission 11 Space Technology: Chapter 1 – Rockets | 11 The Rocket Speed! Escape Velocity We have discussed about propulsion and how the thrust generated helps a Rocket lift-off. However, the goal of a Rocket mission is to escape the Earth’s gravitational pull to put the payload in Space. This is where the concept of Escape Velocity comes into the picture. Escape velocity is the speed at which an object must travel to break free of a planet or moon's gravitational force. Escape Velocity of Earth is 11.2 km/s. If the Rocket's velocity is less than the escape velocity, the Rocket falls back to Earth without putting the payload/satellite in space. We now have a clear understanding of how the Rockets are launched against Earth’s gravity. Let us find out on what factors the size of a Rocket depends. How big should our Rocket be? Primarily, the Rocket chosen for a space mission depends on two factors. The size/volume/mass of payload to be delivered. The orbit in which the payload has to be placed. These two factors determine the amount of fuel and oxidiser (propellants) required by the Rocket to launch the payload to space which in turn decides the size of the Rocket. We know that the payload bay encapsulates our payload. This volume of the payload bay is different for different Rockets. Hence, the Rocket we choose must be such that it accommodates our payload without falling short of space. The size of the Rocket is directly proportional to the size of the payload decided for the mission. Similarly, depending on the altitude at which we would want to detach the payload or the orbit to which we intend to deliver, the fuel requirements of the Rocket vary, massively changing the size of our vehicle. For example: GSLV MK III can carry around 4000 kg payload to GEO (Geostationary Orbit – 35780 km), whereas it can carry around 10,000 kg payload to LEO (Low Earth Orbit – 200-2000 km). This is the reason behind the insane size of the Rockets, as they have to carry large and heavy payloads and travel long distances defying Earth’s gravity. How to control our Rocket during the flight? Following the launch of a Rocket, it is essential to make sure the Rocket maintains its attitude and does not deviate from its course, resulting in a mission failure. To know how this is done, one must first understand the forces (fig. 8) acting on a Rocket and the meaning of a Rocket Trajectory. 12 Space Technology: Chapter 1 – Rockets | 12 Forces on a Rocket i. Thrust: As discussed in the earlier section, this force is generated by the Rocket's propulsion system. The exhaust gases expel through the nozzle imparting a reaction force on the Rocket, propelling it upwards. This force acts about the Centre of Gravity (Cg). ii. Weight: This is the force on the Rocket due to its mass being acted upon by the gravitational acceleration of the Earth about the Centre of Gravity. iii. Lift: The component of Aerodynamic force acting perpendicular to the flight direction caused due to the airflow over the Rocket surface about the Centre of Pressure (Cp). iv. Drag: The Aerodynamic force component acting parallel to the flight direction is caused due to the airflow over the Rocket surface about the Centre of Pressure. Centre of Gravity (Cg) is the location of the point where the entire weight of the body is concentrated. Centre of Pressure (Cp) is the point in a body at which the resultant pressure acts when the body is placed in a fluid. (In our case, fluid is the atmosphere) Figure SEQ Figure \* ARABIC 5: Forces on a Rocket during Flight (Source: Design Spark) Fig. 19: Forces on a Rocket during Flight Rocket Propulsion: Rocket goes to space by producing the Thrust using the propulsion system. The propulsion system includes an Engine or a Motor and the Propellants. Propellants are a combination of Fuel and Oxidizer. An oxidizer is taken in a rocket due to the absence of Oxygen in Space. Rockets are also categorized into various types based on the propulsion system as follows. 1. Solid fuel Rocket Propulsion 2. Liquid fuel Rocket Propulsion 3. Hybrid Rocket Propulsion 4. Cryogenic fuel Rocket Propulsion 5. Ion Propulsion 6. Nuclear Propulsion Let us know more about each one in brief: 1. Solid fuel Rocket Propulsion: The solid motor will have a cylindrical body filled with solid propellant. A solid propellant is a mixture of fuel and oxidizer in its solid form. The solid propellant has a cylindrical hole in the centre which acts as the combustion chamber. A small part of the propellant surface is ignited, which will start the combustion process. As a response to combustion, a huge amount of exhaust gas is produced from the nozzle at high temperature and pressure, accelerating the rocket and pushing it upwards. 13 Space Technology: Chapter 1 – Rockets | 13 If the propellant is ignited once, we cannot turn off the engine until the whole propellant is burned completely. Hence the solid Rocket motors cannot be controlled. A solid Rocket engine produces a huge amount of thrust compared to others. Due to these two reasons, solid rocket motors are primarily used in the first stage and booster stages. 2. Liquid Fuel Rocket Propulsion: A liquid fuel rocket engine utilizes a bipropellant configuration in which fuel and oxidizer are stored in separate tanks. The propellants are drawn from the tanks using separate pumps, or the propellants can also be forced into the combustion chamber with a pressure-fed system (high-pressure gases). Since the propellants are stored separately and are managed through pumps, The liquid systems are controllable, which means we can turn on and off the engines and regulate the flow of propellants in other ways to control the thrust. Liquid Engines have a higher specific impulse (energy content) and can be tested prior to use. It also has several parts in the engine, which makes it structurally less efficient. 3. Hybrid Fuel Rocket Propulsion: A hybrid rocket engine (HRE) is a type of rocket engine that uses both solid and liquid propellants. The fuel will be in a solid-state like in our solid motors without an oxidizer that acts as the combustion chamber, and then the oxidizer will be in a liquid state that could be regulated using pumps. Fig. 20: Types of Rocket Engines 4. Cryogenic Engines The cryogenic engines use Liquid Oxygen (LOX) and Liquid Hydrogen (LH2) as propellants liquefied at -183 deg C and -253 deg C, respectively. LOX and LH2 are stored in separate tanks, and they are pumped individually to ensure a controlled flow rate of propellants inside the combustion chamber. A cryogenic rocket engine consists of a combustion/thrust chamber, igniter, fuel injector, fuel cryopumps, oxidizer cryopumps, gas turbine, cryo valves, regulators, fuel tanks and a rocket engine nozzle. Cryogenic fuels are fuels that require storage at extremely low temperatures in order to maintain them in a liquid state. Fig. 21: Cryogenic Engine of ISRO 14 Space Technology: Chapter 1 – Rockets | 14 5. Ionic Propulsion System: Ionic propulsion is a new type of propulsion system under electronic propulsion. This setup creates thrust by accelerating ions using electricity. A neutral gas is ionized, i.e. the gas is stripped of its electrons using some form of external energy (e.g. heat), creating a cloud of ions which are then accelerated using electricity due to Coulomb force. Ion thrusters are ideal for long-range vacuum trajectories and not for lift- Fig. 22: The illustrative image of NASA's off stages due to their low thrust capacities. This Dawn spacecraft arriving at the dwarf is one of the significant fields of research in the planet Ceres (lower right). (Credits: field of propulsion. NASA/JPL-Caltech) 6. Nuclear Propulsion System: A process in which a highly radioactive element is used to extract energy in a controlled setup is called nuclear fission. The controlled setup includes control rods, moderators, lead casings, etc. This extracted energy is called nuclear energy, which is used as a form of thrust in nuclear thrusters. Nuclear thrusters are still under research and development. This type of propulsion has very little loss of energy and hence high efficiency. One of the major challenges in having such thrusters is core designing and redundant systems to prevent radiation leaks and other hazards. Humans to Space: Towards the end of World War II, Russia acquired the technology of the German V2 rockets from the captured manufacturing plants. Russia studied and soon made replicas of the V2 rocket called R-1, which helped improve its missile and rocket technology. The improved version R-1, called R-7, was modified to become the first satellite launch vehicle called Sputnik 727 and launched the Sputnik satellite. Whereas another version of their rocket - Vostok, launched the first man - Yuri Gagarin to space. Russia went on to develop multiple launch vehicles which are even used to this day, such as Proton, Soyuz, etc. Proton was used to launch the first-ever module of ISS called the Zarya, and Soyuz was used to launch humans to space. From the time America's Space Shuttle Program retired until the crew dragon was introduced, Soyuz was the only rocket capable of launching humans to the ISS. Russia is now developing the next generation of Fig. 23: Soyuz Rocket being Assembled. reusable rockets called Amur. 15 Space Technology: Chapter 1 – Rockets | 15 After launching a set of first satellites, all the space nations went on to send animals and humans to Space. The next step after reaching space was exploring other worlds. On May 25, 1961, U.S. President John F. Kennedy, in his famous speech, announced the ambitious goal of sending an American safely to the Moon before the end of the decade. NASA established the Apollo missions to achieve the goal of landing a human on the lunar surface. The rocket that accomplished this feat was Saturn V. The Saturn V rocket was a pinnacle of rocket engineering in the 1960s. It was 111 meters tall, about the height of a 36-storey building. Fully fuelled for lift-off, the Saturn V weighed 2.8 million kilograms, comparable to the mass of about 400 elephants. At lift-off, the engines of the Saturn V rocket generated enough power equivalent to 160,000 sports cars. On July 20, 1969, as Neil Armstrong took his first steps towards the surface of the moon, he uttered the famous words, "That's one small step for a man, one giant leap for mankind." Fig. 24: Saturn V rocket soaring up to the space. (Credits: NASA) With new goals and technological advancements, the design of the rockets further evolved across the world. One such advancement is the space shuttle. It was intended to provide NASA with a low-cost way to send humans and payloads* to Low Earth Orbit (LEO). Space shuttles launched vertically like a regular rocket and returned to Earth like a glider and landed like an aircraft on a runway. Space shuttles made the construction of ISS (International Space Station) possible. They were also used to launch and service the Hubble Space Telescope, visit Mir, the Russian space station and launch numerous probes* and satellites. Fig. 25: NASA’s first space shuttle mission, STS-1 Although we read at the beginning of the chapter, Rockets were first used in China (900 A.D.), they did not participate in the Space Race. But in 1950, China made an effort to pursue the Chinese National Columbia. Space Program. Initially, through the Missile program and later by a cooperation agreement with Soviet Union and gained enough (Credits: Space Centre) knowledge about rocket technology through the Soviet R-2 program. Later in 1960, China began to work on its Rocket Program and worked on the Crewed mission program. Today China, through China National Space Administration (CNSA) stands as the only third country to have the capability to launch humans to Space. Fig. 26: CNSA Rocket – Long March 16 Space Technology: Chapter 1 – Rockets | 16 Rockets of India: The launch of the US-made ‘Nike Apache’, the first sounding rocket of India from Thumba near Thiruvananthapuram, Kerala, on November 21, 1963, at 0625 pm IST, marked the beginning of the Indian Space Programme. Later days saw the launching of two-stage rockets imported from Russia (M-100) and France (Centaure). From 1967, ISRO started launching its own series of sounding rockets under the Rohini series from TERLS, which was made in India. The sounding rocket programme was indeed the strong base on which the edifice of launch vehicle technology was built. Fig. 27: (Left) First Launch of Nike Apache and (right) Rohini series – Indian Sounding Rockets Evolution of ISRO Launch Vehicles: After gaining experience with the sounding rockets, ISRO went on to build the rockets capable of launching satellites from India. From the below picture, Satellite Launch Vehicle (SLV) and Augmented Satellite Launch Vehicle (ASLV) are not operational at present. Polar Satellite Launch Vehicle (PSLV) and Geosynchronous Satellite Launch Vehicle (GSLV Mk II and Mk III) are the Rockets which ISRO using till date. Fig. 28: Genesis of Indian Rockets 17 Space Technology: Chapter 1 – Rockets | 17 Satellite Launch Vehicle (SLV): SLV-3 is a four-stage rocket launched during 1979- 1983 by ISRO. It weighs around 17 tonnes (1 tonne = 1000 kg) and can carry 40 kg of payload to LEO. SLV used solid propellant for all four stages during its flight time. The first two stages used PBAN (Polybutadiene acrylonitrile), and the last two stages used HEF-20. SLV-3 program successfully put the RS-1(Rohini) Satellite in its intended orbit from Satish Dhawan Space Centre (SDSC), Sriharikota, on July 18, 1980. RS-1 became the first Indian satellite indigenously built and launched from India. With this success, India became the 6th nation to join the list of Space faring nations. Fig. 29: SLV -3 on Launch Pad Augmented Satellite Launch Vehicle (ASLV): ASLV was the intermediate step before achieving indigenous launcher capability for heavier communication and remote sensing satellites. ASLV differed from SLV mainly in its increased payload capacity of 150 kg, which helped to carry a lot more sensors onboard. To achieve this higher payload capability, ASLV had five stages and used solid propellant in all its stages. ASLV had two Strap on boosters in the first stage, which helped to carry heavy payloads. ASLV served between the period 1987 to 1994. During this period, ISRO launched four missions to deliver satellites to Low Earth Orbit (LEO). Out of four, only two were successful. Though ASLV usage in the Indian space history is less, it paved a new path for space engineers to explore new technologies such as vertical integration, closed- loop guidance, strap-on technology, inertial navigation, and bulbous heat shield. These developments helped India to reach farther in Space Technology. Fig. 30: ASLV coming out of Launch Vehicle Assembly Building 18 Space Technology: Chapter 1 – Rockets | 18 Polar Satellite Launch Vehicle (PSLV): PSLV also known as the workhorse of ISRO, was initially designed to launch satellite into Sun Synchronous Orbit (SSO) / LEOs. As the years progressed, there were many developments that enhanced the payload capacity of PSLV from 1000 kg to 1900 kg and widened the capability of transferring satellites to LEO, SSO, Sub-GTO, and GTO. PSLV is a four-stage rocket launch vehicle excluding boosters. The number of boosters used by the PSLV launch vehicle is dependent on mission requirements. Based on the number of strap-on motors, there are different versions in PSLV like core-alone, XL, QL, and DL. PSLV is a four-stage rocket, with 320 tonnes of lift-off mass and can carry 1850 kg of Payload to Sun-synchronous Polar orbits. The first and third stages and the Strap-on boosters use HTPB based solid propellant. Whereas a liquid- propellant engine propels the second and fourth stages of PSLV. The engine used in the 2nd stage of PSLV, known as the VIKAS engine, was developed in collaboration with France. The 4th stage of PSLV uses a twin pressure fed engine known as the PS4 engine. The first launch of PSLV, dated September 20, 1993, was unsuccessful. The debut failure did not make the ISRO setback. After that, ISRO came back with consecutive 25 successful launches. Some of the notable successful launches of PSLV are Chandrayaan-1, Mars Orbiter Mission (MOM) aka Mangalyaan, and record-breaking 104 satellites in a single launch. Fig. 31: PSLV Geosynchronous Satellite Launching Vehicle (GSLV): GSLV is a three-stage launch vehicle with four boosters with the capability of 414 tonnes of lift-off mass, can carry 2500 kg of Payload to GTO and 5000 kg to LEO. The part of GSLV which makes it unique from other launch vehicles is the final stage; it uses a cryogenic stage which was very new to ISRO. For the GSLV MK-I version, the cryo stage was imported from Russia. After developing the Indian-made cryogenic engine CE-7.5, the GSLV series was named GSLV MK-II. Parallelly ISRO put efforts for developing indigenous Cryo Upper stage (CUSP) and qualified C12.5 stage and inducted it into GSLV Mk II programme. The first launch of GSLV was on April 18, 2001, and the last GSLV launch happened on August 12, 2021. Fourteen launches were made during this period, including MK-I and MK-II series. Out of them, eight were successful, two were partially successful, and four were failures. From 2014, GSLV marked six continuous successful launches. Fig. 32: GSLV MkII Did you know? 19 Space Technology: Chapter 1 – Rockets | 19 GSLV Mk III GSLV MK-III is the fourth generation launch vehicle for lifting 10- ton category satellites to LEO and 4-ton category satellites to GTO. Moreover, GSLV MK-III is planned to be the first human-rated launch vehicle developed by ISRO for the Gaganyaan mission. GSLV Mk III has many unique features like it has two boosters with solid propellant, are considered the first stage that uses 200-ton HTPB based solid propellants on each. In contrast, the second stage is powered with two Vikas engines and uses a 110-ton hypergolic propellant combination UH25+N2O4. The uppermost last stage uses an upgraded C20 cryogenic engine that works with the Hydrolox combination (LOX+LH2). Subsequent flights of MK-III were used to launch GSAT-19, GSAT- 29 and Chandrayaan-II. Chandrayaan-II is the heaviest spacecraft ISRO has launched as of now. GSLV MK-III also called as Fat Boy of ISRO, has been successful in all of its four missions. The first Indian designed and built human space flight launch mission, ‘Gaganyaan’, is also planned to go on GSLV MK-III. Fig. 33: GSLV Mk III The Future Launch Vehicle: Reusable Launch Vehicle (RLV – TD): RLV is the most complex vehicle designed by ISRO. The technology demonstrator of RLV, viz, RLV – TD is a fusion of aircraft and rocket technology. It is envisaged to maintain integrity between both systems and make the mission successful. It uses the scramjet engine to achieve supersonic and hypersonic flight. Currently, ISRO completed HEX on May 23, 2016, and the mission was successful in the first attempt itself. RLV-TD reached a peak altitude of 65 km and started the descent phase in hypersonic speed. RLV-TD reached the designated area in the Bay of Bengal, 450 km away from the Sriharikota launch station. Total flight was 770 seconds, and throughout this duration, RLV-TD was tracked and the performance of autonomous* navigation and control system, thermal protection system, and re-entry mission management from ground stations at Sriharikota and ship-borne terminal – all were monitored. In the end, RLV-TD splashed down at the designated point in the Bay of Bengal. Fig. 34: RLV – TD 20 Space Technology: Chapter 1 – Rockets | 20 Small Satellite Launch Vehicle (SSLV): SSLV is the latest launch vehicle by ISRO. The objective of the SSLV project is the development of a vehicle capable of launching small, micro and nano satellites. This vehicle is capable of launching up to 500 kg satellite into a 500 km planar orbit. The vehicle is configured with three solid propulsion stages and a liquid propulsion-based Velocity Trimming Module (VTM) terminal stage. SSLV is 2m in diameter and 34m in length with a lift-off weight of ~120 tons. It will be able to launch multiple small satellites into orbit on a single mission and is designed to be low cost and reduce development time for commercial launches on-demand. It is designed to cater to the emerging global small satellite launch service market. A new launch pad is being built in Sriharikota, which will reduce the turnaround time and capability to launch 6-8 missions per year. Fig. 35: SSLV Reusable Launch Vehicle: Throughout the history of the rockets, most of the designs were expendable, which means when the rocket’s stage spent all the fuel it contains, it is discarded and falls back to earth. The only exception was NASA’s Space shuttle, which could re-use the orbiter but still, the boosters and the main fuel tanks were discarded. Elon musk’s rocket company, SpaceX wants to make space access cheaper and has designed Its rockets to be re-usable. Falcon 9, was the first orbital-class rocket to land back after being launched. The first stage of the rocket after releasing the payload lands back using steerable grid fins and deployable landing legs. Even though the technology used have been used and tested before, landing back an orbital class rocket pioneered a whole new class of launch vehicles. Fig. 36: First stage boosters of Falcon Heavy landing. (Credits: SpaceX) 21 Space Technology: Chapter 1 – Rockets | 21 After the launch of Falcon 9, the first stage carries the rocket to an altitude of 50 miles (80km), at which the stage separation occurs. After the first stage separation, it doesn’t immediately turn back, but it continues on its trajectory for a while due to its momentum. At the same time, the second stage continues to propel forward in order to complete the next phase of the mission. During the coasting phase, the cold gas thrusters flip the stage 180 degrees so that the bottom end of the booster points towards the Earth for landing. Fig. 37: Mission Flight Path of SpaceX Crew Dragon While entering the Earth’s atmosphere, the surface area of the booster creates drag force, thereby reducing the velocity of the booster. Once the booster enters the lower atmosphere, the grid fins steer the booster towards the landing pad. The velocity of the booster is further reduced by re-igniting one of the engines. Finally, the booster lands with the help of landing legs which deploy during the touchdown. With the success of Falcon 9, SpaceX went on to build falcon heavy and is in the process of designing the Starship, the first fully re-useable rocket aimed to take people to Mars. Launch Pads Rockets cannot be launched from any random place, for the same reason, we have space ports for the space agency’s where they launch their rockets from. The criteria for selecting the Spaceports/Launching stations are as follows: Fig. 38: Satish Dhawan Space Centre, Sriharikota. 1. Eastward Launches and Nearness to the Equator: We know that the Earth rotates and revolves on its axis. With that, it causes angular momentum. This angular momentum boosts the rocket flight path, which helps decrease the amount of fuel required. Earth’s maximum angular momentum is observed from the equatorial 22 Space Technology: Chapter 1 – Rockets | 22 region, and as one moves away from the equator towards poles, angular momentum decreases. 2. Inhabited Landmass / Closeness to sea: As the trajectory of the launch vehicle is towards east or south, for geosynchronous and sun-synchronous missions, respectively, the flight path should avoid the populated landmasses. There are chances for failure during the launch of a rocket. It might happen at any stage; also, it is unpredictable. Sometimes, rocket manoeuvres can be controlled even after a system failure via standby systems. In that case, destruct mode is authorised, and the rocket will be plunged into the sea. In the worst case, if the rocket is uncontrollable, it would be better to crash in the sea than in populated zones. 3. Geographical location stability/ Safety distances: Vast free land is essential to locate the facilities prone to explosion. Geographic location plays a vital role in choosing a launching station. As the amount of thrust and vibration produced during a launch is tremendous, the ground should be capable of providing that support. The landmass should be vast enough to hold all the workstations and storage areas needed. Apart from the above-discussed criteria, there are other factors such as climate variation throughout the year, geomagnetic field, etc., which is also considered while selection of Spaceport. ISRO has the spaceports in the following across India; Vikram Sarabhai Space Centre (VSSC), Thiruvananthapuram, Kerala. Satish Dhawan Space Centre (SDSC), Sriharikota, Andrapradesh. Balasore Range, Odisha (not operational) Fig. 39: Location of Spaceports of India -----------------------------------------------x—x—x—x—x-------------------------------------------- 23 Space Technology: Chapter 1 – Rockets | 23