Organic Chemistry Reviewer PDF
Document Details
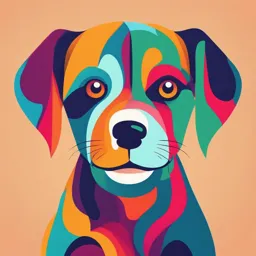
Uploaded by ExcellentLesNabis2585
Tags
Summary
This document reviews carboxylic acids and their derivatives, including their structures, reactivities, and reactions. It discusses topics such as acid chlorides, esters, amides, and nitriles, along with their properties and reactions. The document also explores the concept of acidity and how substituents influence it in carboxylic acids and related compounds. The document is a learning resource on organic chemistry.
Full Transcript
Lesson 1: **Carboxylic Acids and Their Derivatives: Structure, Reactivity, and Related Reactions** Objectives: 1\. Understand the structure and reactivity of carboxylic acids and their derivatives. 2\. Perform related reactions. Carboxylic acids and their derivatives are important functional gro...
Lesson 1: **Carboxylic Acids and Their Derivatives: Structure, Reactivity, and Related Reactions** Objectives: 1\. Understand the structure and reactivity of carboxylic acids and their derivatives. 2\. Perform related reactions. Carboxylic acids and their derivatives are important functional groups in organic chemistry, characterized by the presence of the carboxyl group (-COOH) in carboxylic acids and modified forms of this group in their derivatives. These compounds are central to both biological processes and synthetic organic chemistry. 1\. Understanding the Structure and Reactivity of Carboxylic Acids and Their Derivatives **Carboxylic Acids** - General Formula: R−COOHR-COOHR−COOH (where RRR is an alkyl or aryl group). - Functional Group: Carboxyl group (-COOH), consisting of: - A carbonyl group (C=O). - A hydroxyl group (-OH) attached to the same carbon atom. - Physical Properties: - High boiling points due to hydrogen bonding. - Solubility in water decreases with increasing chain length due to hydrophobic effects. - Acidity: - Weak acids (pKa \~4-5) due to the ability to donate a proton (H+). - Acid strength is influenced by the substituents on the RRR-group: - Electron-withdrawing groups (e.g., halogens, nitro) increase acidity. - Electron-donating groups (e.g., alkyl) decrease acidity. **Carboxylic Acid Derivatives** Carboxylic acids form derivatives by replacing the hydroxyl group (-OH) with other substituents. These derivatives have varying reactivities depending on the leaving group. Carboxylic acid derivatives are compounds that contain a functional group related to carboxylic acids. These include **acid chlorides**, **esters**, **amides**, and **nitriles**. They differ in reactivity, with acid chlorides being the most reactive due to their highly electrophilic carbonyl group. **Esters** are less reactive than acid chlorides but more reactive than **amides**, which are stabilized by resonance with the nitrogen\'s lone pair. **Nitriles** are generally less reactive than acid chlorides and esters. The reactivity order from most to least reactive is: **acid chlorides \> esters \> amides \> nitriles**. 1. Acid Chlorides (R-COCl): - Highly reactive due to the electronegative chlorine atom. - Used in acylation reactions (e.g., Friedel-Crafts acylation). 2. Esters (R-COOR\'): - Formed by reacting carboxylic acids with alcohols in the presence of an acid catalyst (esterification). - Less reactive than acid chlorides. 3. Amides (R-CONH₂, R-CONHR\', R-CONR₂): - Formed by reacting carboxylic acids or acid derivatives with ammonia or amines. - Very stable due to resonance and strong hydrogen bonding. 4. Anhydrides (R-CO-O-COR\'): - Formed by the dehydration of two carboxylic acids or by reacting acid chlorides with carboxylic acids. - Reactive, commonly used in synthesis. 5. Nitriles (R-CN): - Contain a carbon triple-bonded to a nitrogen atom. - Can be hydrolyzed to carboxylic acids. **2. Related Reactions of Carboxylic Acids and Their Derivatives** Carboxylic acids and derivatives undergo various reactions, making them versatile in organic synthesis. When ethanol reacts with acetic acid in the presence of an acid catalyst (such as sulfuric acid), the product formed is ethyl acetate, which is an ester. This reaction is known as an esterification reaction.  **Reactions of Carboxylic Acid Derivatives** **Amides** undergo [nucleophilic acyl substitution at the slowest rate compared to other carboxylic acid derivatives]. This is due to the resonance between the lone pair of electrons on the nitrogen and the carbonyl group, which makes the carbonyl carbon less electrophilic and less susceptible to nucleophilic attack. As a result, amides are less reactive in nucleophilic acyl substitution reactions. The presence of a **methyl group (-CH₃)** on the carbon chain of a carboxylic acid generally [makes the acid less acidic.] This is because the methyl group is an electron-donating group through both inductive and hyperconjugative effects, which increases the electron density on the carboxyl group (-COOH). This increased electron density makes it harder for the carboxyl group to lose a proton (H⁺) and thus decreases the acidity of the compound. **Fischer Esterification: Synthesis of Ethyl Acetate from Acetic Acid and Ethanol** [To convert acetic acid into an ester], [**react acetic acid** (ethanoic acid) with an alcohol] (e.g., ethanol) in the presence of an acid catalyst (such as sulfuric acid). This esterification reaction forms ethyl acetate (an ester) and water. **Acid-Catalyzed Hydrolysis of Ethyl Acetate to Acetic Acid** To convert **ethyl acetate** into a **carboxylic acid**, perform **acid-catalyzed hydrolysis**. [Add water to ethyl acetate in the presence of an **acid catalyst**] (such as **HCl** or **H₂SO₄**), which breaks the ester into **acetic acid** and **ethanol**. **Applications in Organic Synthesis** - Polymers: Esters (polyesters) and amides (nylons) are common in synthetic materials. - Pharmaceuticals: Many drugs are carboxylic acid derivatives. - Food Industry: Esters are used as flavoring agents. **IUPAC Nomenclature of Carboxylic Acids** The International Union of Pure and Applied Chemistry (IUPAC) system provides a systematic method for naming carboxylic acids. The rules for naming carboxylic acids involve identifying the longest continuous chain of carbon atoms containing the carboxyl group (-COOH) and modifying the base name accordingly. **Rules for Naming Carboxylic Acids** 1. **Identify the Parent Chain**: - Select the longest carbon chain containing the carboxyl group (-COOH). - The carboxyl carbon is always part of the parent chain and is assigned the number **1**. 2. **Replace the Alkane Suffix**: - Replace the **-e** ending of the corresponding alkane with **-oic acid**. - Example: Methane (CH4CH\_4CH4) → Methanoic acid (HCOOHHCOOHHCOOH). 3. **Add Prefixes for Substituents**: - Indicate any substituents on the chain with their position numbers and names. - Example: 3-methylbutanoic acid. 4. **Special Cases**: - If there are two carboxyl groups, the suffix becomes **-dioic acid**, and the name includes both groups in the parent chain. - Example: Ethanedioic acid (HOOC−COOHHOOC-COOHHOOC−COOH). 5. **Common Names**: - Some carboxylic acids have widely recognized common names in addition to their IUPAC names. These are often derived from their natural sources. **Examples** 1. **Simple Carboxylic Acids**: - HCOOHHCOOHHCOOH: Methanoic acid (common name: Formic acid). - CH3COOHCH\_3COOHCH3COOH: Ethanoic acid (common name: Acetic acid). - CH3CH2COOHCH\_3CH\_2COOHCH3CH2COOH: Propanoic acid. 2. **Branched-Chain Acids**: - (CH3)2CHCOOH(CH\_3)\_2CHCOOH(CH3)2CHCOOH: 2-Methylpropanoic acid. - CH3CH(CH3)CH2COOHCH\_3CH(CH\_3)CH\_2COOHCH3CH(CH3)CH2COOH: 3-Methylbutanoic acid. 3. **Dicarboxylic Acids**: - HOOC−COOHHOOC-COOHHOOC−COOH: Ethanedioic acid (common name: Oxalic acid). - HOOC−(CH2)2−COOHHOOC-(CH\_2)\_2-COOHHOOC−(CH2)2−COOH: Butanedioic acid (common name: Succinic acid). 4. **Aromatic Carboxylic Acids**: - C6H5COOHC\_6H\_5COOHC6H5COOH: Benzoic acid. - HOC6H4COOHHOC\_6H\_4COOHHOC6H4COOH: 2-Hydroxybenzoic acid (common name: Salicylic acid). **Common Prefixes for Substituents** - **Halogens**: fluoro-, chloro-, bromo-, iodo-. - **Alkyl Groups**: methyl-, ethyl-, propyl-, etc. - **Hydroxy Groups**: hydroxy-. - **Nitro Groups**: nitro-. **Naming Priorities** - The carboxyl group has the **highest priority** in naming. If other functional groups are present, they are treated as substituents. - Example: CH3CH(OH)COOHCH\_3CH(OH)COOHCH3CH(OH)COOH is 2-Hydroxypropanoic acid. **Simple Carboxylic Acids** **Formula** **IUPAC Name** **Common Name** --------------- ---------------- ----------------- HCOOH Methanoic acid Formic acid CH3COOH Ethanoic acid Acetic acid CH3CH2COOH Propanoic acid Propionic acid CH3CH2CH2COOH Butanoic acid Butyric acid **Lesson 2: Amines and Amides** **Amines:** - **Structure:** Amines are organic compounds with the general formula **R-NH₂ (primary), R₂NH (secondary),** or **R₃N (tertiary)**. The nitrogen atom is bonded to one or more carbon atoms and has a lone pair of electrons. - **Properties:** - **Basicity:** Amines are weak bases because nitrogen has a lone pair of electrons that can accept a proton (H⁺). - **Boiling Points:** Amines have higher boiling points than hydrocarbons of similar size due to hydrogen bonding, especially in primary and secondary amines. - **Solubility:** Small amines are soluble in water because they can form hydrogen bonds with water molecules. Larger amines are less soluble. - **Odor:** Many amines have a characteristic fishy odor due to their volatility. - **Reactions:** - **Acid-Base Reactions:** Amines react with acids (e.g., hydrochloric acid) to form **ammonium salts** (e.g., R-NH₂ + HCl → R-NH₃Cl). - **Nitration:** Amines undergo electrophilic aromatic substitution to form **substituted aniline** derivatives when reacted with nitric acid. - **Reduction:** Amines can be reduced from nitro compounds or imines using reducing agents like **LiAlH₄**. **Amides:** - **Structure:** Amides have the general structure **R-C(=O)-NH₂** (primary amides), **R-C(=O)-NHR** (secondary amides), or **R-C(=O)-NR₂** (tertiary amides), where the nitrogen is attached to a carbonyl group (C=O). - **Properties:** - **Basicity:** Amides are less basic than amines because the lone pair on nitrogen is delocalized through **resonance** with the carbonyl group, making it less available to accept protons. - **Boiling Points:** Amides have high boiling points due to strong hydrogen bonding between the nitrogen and oxygen atoms. - **Stability:** Amides are more stable than amines due to resonance stabilization between the nitrogen lone pair and the carbonyl group. - **Reactions:** - **Hydrolysis:** Amides can be hydrolyzed under acidic or basic conditions to form **carboxylic acids** and **amines** (or ammonia) (R-C(=O)-NH₂ + H₂O → R-C(=O)-OH + NH₃). - **Reduction:** Amides can be reduced to amines using powerful reducing agents like **LiAlH₄**. - **Acylation:** Amides can undergo reactions with **acyl chlorides** to form substituted amides. - Amides can be synthesized from amines through several methods. The most common and efficient approach is reacting an amine (primary or secondary) with an acyl chloride in the presence of a base, such as pyridine, to neutralize the HCl byproduct. This reaction produces the corresponding amide. Another method involves combining an amine with a carboxylic acid and a dehydrating agent like DCC or EDC under heat, which facilitates the removal of water and forms the amide. Additionally, esters can react with amines under heat to yield amides and alcohol as a byproduct. It is important to note that primary and secondary amines are suitable for these reactions, while tertiary amines cannot participate because they lack an N-H bond. **Reduction of an Amide to an Amine:** Amides are reduced to amines by reacting them with a strong reducing agent like **lithium aluminum hydride (LiAlH₄)**. This process involves the cleavage of the C=O bond and the conversion of the carbonyl group into a CH₂ group. **IUPAC Nomenclature:** - **Amines:** - **Primary amines:** Named by replacing the \"e\" in the parent alkane with \"amine\" (e.g., **methylamine** for CH₃NH₂). **Methylamine (CH₃NH₂):** Highly soluble in water due to its ability to form hydrogen bonds with water molecules and its small size. - **Secondary amines:** Named with the prefix for the two groups attached to nitrogen (e.g., **dimethylamine** for (CH₃)₂NH). **N,N-Dimethylamine (CH₃N(CH₃)₂):** Moderately soluble, as the nitrogen can still form hydrogen bonds with water, though steric hindrance reduces the solubility slightly. - **Tertiary amines:** Named with the prefix for the three groups attached to nitrogen (e.g., **trimethylamine** for (CH₃)₃N). **N,N-Dimethyl ethanamide (CH₃CON(CH₃)₂):** Least soluble because the bulky methyl groups attached to nitrogen reduce hydrogen bonding potential, and the molecule is more hydrophobic overall compared to the other compounds. - **Amides:** - **Primary amides:** Named by replacing the "acid" of the carboxylic acid name with \"amide\" (e.g., **ethanamide** for CH₃CONH₂). - **Secondary and tertiary amides:** The nitrogen atom is named by adding the appropriate **N-** prefix (e.g., **N-methyl ethanamide** for CH₃CONHCH₃). **Summary of Key Concepts:** - **Amines** are characterized by their basicity, ability to form hydrogen bonds, and higher boiling points compared to hydrocarbons. They are reactive with acids and can form salts. - **Amides** are less basic than amines, highly stable due to resonance, and have high boiling points due to strong hydrogen bonding. They can be hydrolyzed to produce carboxylic acids and amines. - Both compounds undergo various reactions, including hydrolysis, reduction, and acylation, with distinctive properties due to their structure and functional groups. **Lesson 3 Aromatic Compounds** Aromatic compounds are a class of chemical compounds that contain a benzene ring or a structure similar to it. These compounds are characterized by their stability due to the delocalization of electrons in their conjugated π-system. The most common example of an aromatic compound is **benzene (C₆H₆)**, which consists of six carbon atoms in a hexagonal ring, each bonded to a hydrogen atom. Key characteristics of aromatic compounds include: 1. **Planar structure**: The atoms in the ring are arranged in a flat, planar structure. 2. **Conjugated π-system**: The alternating single and double bonds between the carbon atoms create a conjugated system where electrons are delocalized over the entire ring. 3. **Resonance**: The structure of aromatic compounds is best represented by resonance, meaning the actual structure is a hybrid of several possible configurations. 4. **Stability**: Aromatic compounds are more stable than alkenes or other unsaturated compounds due to the delocalized electrons. Examples of aromatic compounds include: - **Benzene (C₆H₆)** - **Toluene (C₆H₅CH₃)** (a methyl group attached to a benzene ring) - **Aniline (C₆H₅NH₂)** (an amino group attached to a benzene ring) - **Naphthalene (C₁₀H₈)** (two fused benzene rings) Aromatic compounds are commonly used in the production of various chemicals, pharmaceuticals, plastics, and dyes. **Naming aromatic compounds follows specific rules established by the International Union of Pure and Applied Chemistry (IUPAC).** **1. Basic Aromatic Compounds (Benzene Derivatives)** - The simplest aromatic compound is **benzene (C₆H₆)**. - When a substituent is attached to the benzene ring, the compound is named by adding the substituent\'s name as a prefix to **benzene**. **Examples:** - **Toluene**: Methyl group (CH₃) attached to benzene (C₆H₅CH₃). - **Aniline**: Amino group (NH₂) attached to benzene (C₆H₅NH₂). - **Phenol**: Hydroxyl group (OH) attached to benzene (C₆H₆OH). **2. Common Names for Substituted Benzene Compounds** Certain aromatic compounds have common names that are often used instead of IUPAC names. These names are derived from the compound's structure or historical usage. **Examples:** - **Xylene**: A benzene ring with two methyl groups (C₆H₄(CH₃)₂). - **Toluene**: A methyl group attached to a benzene ring. - **Benzaldehyde**: Benzene ring with an aldehyde group (C₆H₅CHO). **3. Numbering the Benzene Ring** When there are two or more substituents on the benzene ring, IUPAC rules dictate numbering the carbons in the ring to give the substituents the lowest possible numbers. - If there are two substituents, you can use **ortho (1,2-)**, **meta (1,3-)**, or **para (1,4-)** to describe the positions. **Examples:** - **1,2-Dimethylbenzene** (Ortho-dimethylbenzene) --- Two methyl groups on adjacent carbons. - **1,3-Dimethylbenzene** (Meta-dimethylbenzene) --- Two methyl groups on carbons 1 and 3. - **1,4-Dimethylbenzene** (Para-dimethylbenzene) --- Two methyl groups on carbons 1 and 4. **4. Substituted Aromatic Compounds** - If there are more than two substituents, the ring is numbered to give the substituents the lowest possible numbers, and their positions are indicated by numbers. **Example:** - **3-Bromo-1-methylbenzene**: Bromine and methyl groups are positioned at carbons 3 and 1, respectively. **5. Polycyclic Aromatic Compounds** These compounds consist of multiple benzene rings fused together, such as **naphthalene**, **anthracene**, and **phenanthrene**. - **Naphthalene**: Two benzene rings fused together (C₁₀H₈). - **Anthracene**: Three benzene rings fused together in a linear arrangement (C₁₄H₁₀). - **Phenanthrene**: Three benzene rings fused together but in a non-linear arrangement (C₁₄H₁₀). **6. Heterocyclic Aromatic Compounds** These are aromatic compounds that contain atoms other than carbon in the ring, such as nitrogen, oxygen, or sulfur. These are named by combining the name of the heteroatom with the suffix "-azole," "-ine," or other related terms. **Examples:** - **Pyridine**: A six-membered ring with one nitrogen atom (C₅H₅N). - **Furan**: A five-membered ring with one oxygen atom (C₄H₄O). - **Thiophene**: A five-membered ring with one sulfur atom (C₄H₄S). **Lesson 4 Environmental Organic Chemistry** Environmental Organic Chemistry is the study of organic chemicals in the environment, their sources, behavior, fate, and impacts on the environment. It focuses on how organic compounds (such as pesticides, pharmaceuticals, industrial chemicals, and pollutants) interact with the atmosphere, water, soil, and living organisms. This field is critical for understanding the dynamics of chemical pollutants and their effects on ecosystems and human health. **Key Areas in Environmental Organic Chemistry** 1. **Sources of Organic Pollutants:** - **Natural Sources:** Organic chemicals that originate from natural processes like plant decomposition, wildfires, and volcanic eruptions. - **Anthropogenic Sources:** Human-made sources such as agricultural runoff (e.g., pesticides, herbicides), industrial effluents, waste disposal, and the use of fossil fuels. 2. **Fate of Organic Pollutants:** - **Degradation:** Organic pollutants may degrade through biological, chemical, or photolytic processes. Microbial degradation in soils or water can break down pollutants, but some chemicals can persist for years. - **Persistence:** Some organic chemicals, like persistent organic pollutants (POPs), resist degradation and accumulate in the environment, causing long-term harm. - **Transport:** Organic compounds can be transported through air, water, and soil. Some pollutants can travel long distances from their source (e.g., airborne pollutants, waterborne chemicals). 3. **Chemical Reactions in the Environment:** - **Hydrolysis:** The reaction of organic compounds with water leading to breakdown. - **Photodegradation:** Chemical decomposition of pollutants caused by sunlight, particularly UV radiation. - **Oxidation/Reduction:** Chemical reactions involving the transfer of electrons that can affect the stability and mobility of pollutants. - **Sorption:** The process by which organic chemicals bind to solid particles in soil or sediments. 4. **Environmental Impact of Organic Pollutants:** - **Toxicity:** Organic pollutants can be toxic to plants, animals, and humans. For example, many pesticides or industrial chemicals are known to cause developmental issues, cancer, or endocrine disruption. - **Bioaccumulation and Biomagnification:** Some organic chemicals accumulate in living organisms over time. As you move up the food chain, concentrations of these chemicals may increase, leading to harmful effects on top predators. - **Ecological Disruption:** Organic chemicals, especially POPs, can disrupt ecosystems by harming species, reducing biodiversity, or altering nutrient cycles. 5. **Analytical Methods in Environmental Organic Chemistry:** - **Gas Chromatography (GC):** Used for detecting volatile organic compounds in air and water samples. - **Liquid Chromatography (LC):** Applied to the analysis of organic pollutants in water, soil, or biota. - **Mass Spectrometry (MS):** Often used in conjunction with GC or LC for identifying and quantifying pollutants. - **Spectroscopy:** Used to detect specific chemical bonds and functional groups in organic pollutants. 6. **Case Studies of Organic Pollutants:** - **Persistent Organic Pollutants (POPs):** These include chemicals like DDT, PCBs, and dioxins, which have been banned due to their persistence, bioaccumulation, and toxicity. - **Pharmaceuticals and Personal Care Products (PPCPs):** These emerging contaminants have been detected in water systems due to improper disposal or inefficient removal during wastewater treatment. - **Plasticizers and Endocrine Disruptors:** Chemicals like phthalates and bisphenol A (BPA) found in plastics, which can leach into the environment and disrupt hormonal systems. 7. **Solutions and Management:** - **Bioremediation:** The use of microorganisms or plants to degrade or neutralize pollutants in the environment. - **Advanced Oxidation Processes (AOPs):** Techniques that use strong oxidants like ozone or UV radiation to break down organic pollutants in water. - **Regulations and Policies:** Many countries have established regulations (e.g., the Stockholm Convention on POPs) to limit or eliminate the use and release of harmful organic pollutants. - **Green Chemistry:** An approach focused on designing chemical products and processes that reduce or eliminate the use of harmful substances in the environment. **Importance of Environmental Organic Chemistry** Environmental Organic Chemistry is crucial for addressing environmental pollution, ensuring safe drinking water, and protecting public health. By understanding how organic chemicals behave in the environment, scientists can better assess risks, develop treatment technologies, and create policies to minimize harm to ecosystems and humans. Lesson 1: Microbial Metabolism Microbial Metabolism refers to the chemical processes that occur within microorganisms to maintain life, including energy production, nutrient assimilation, and waste elimination. Microbes, being highly diverse, employ a wide range of metabolic pathways that enable them to thrive in various environments. These processes are essential for growth, reproduction, and adaptation to changing environmental conditions. Key Components of Microbial Metabolism: 1. Enzymes in Metabolism: - Enzymes are specialized proteins that act as catalysts, speeding up biochemical reactions without being consumed in the process. In microbial metabolism, enzymes facilitate the breakdown and synthesis of molecules involved in metabolic pathways. - - Enzymes exhibit high specificity, meaning that each enzyme catalyzes only a particular reaction or set of reactions, contributing to the regulation and efficiency of metabolic processes. - 2\. Metabolic Pathways: - Metabolic pathways are a series of interconnected biochemical reactions. These pathways can be classified as catabolic (breaking down molecules to release energy) or anabolic (building complex molecules from simpler ones, requiring energy input). - - Catabolic pathways include: - Glycolysis: The breakdown of glucose into pyruvate, yielding ATP and NADH (a carrier of electrons). Citric Acid Cycle (Krebs Cycle): The further breakdown of pyruvate to produce high-energy electron carriers (NADH, FADH₂) and carbon dioxide. Electron Transport Chain (ETC) and Oxidative Phosphorylation: The final step in aerobic metabolism, where electrons from NADH and FADH₂ are passed through a series of enzymes, ultimately leading to the production of ATP via ATP synthase. - Anabolic pathways include: - Biosynthesis: The creation of complex molecules like proteins, lipids, and nucleic acids from simpler precursors. Gluconeogenesis: The synthesis of glucose from non-carbohydrate sources. Amino Acid and Nucleotide Synthesis: The formation of amino acids and nucleotides used for protein and nucleic acid production. 3\. Energy Production and Utilization: - Microorganisms generate energy primarily through the conversion of chemical bonds in nutrients (e.g., sugars, lipids, proteins) into ATP. - - ATP (Adenosine Triphosphate) is the primary energy currency in microbial cells. ATP is produced in various metabolic pathways, including: - Fermentation: An anaerobic process that breaks down glucose into smaller products (like ethanol or lactic acid) while producing a small amount of ATP. Aerobic Respiration: A process that requires oxygen, in which glucose is completely oxidized to carbon dioxide and water, generating large amounts of ATP. Anaerobic Respiration: Similar to aerobic respiration, but instead of oxygen, other electron acceptors (e.g., nitrate, sulfate) are used to generate ATP. - Energy utilization: ATP is used by microorganisms to power various cellular functions, such as active transport of nutrients, biosynthesis of macromolecules, movement (flagella or pili), and cell division. - 5. Diversity of Metabolism in Microorganisms: Microorganisms exhibit remarkable metabolic diversity, allowing them to survive in a wide range of environments, including extreme conditions. Some examples include: - Heterotrophs: Organisms that obtain carbon by consuming organic molecules (e.g., glucose). Most bacteria, fungi, and animal cells are heterotrophic. - - Autotrophs: Organisms that use carbon dioxide as their carbon source. They can be photoautotrophs, which use light energy (e.g., cyanobacteria), or chemoautotrophs, which use chemical energy from inorganic compounds (e.g., sulfur bacteria). - - Fermentative Microbes: Some microorganisms, like certain bacteria and yeast, can survive without oxygen through fermentation, producing energy by converting glucose into products such as ethanol or lactic acid. - 6. Nutrient Uptake and Assimilation: - Microbial metabolism also includes the uptake of essential nutrients like carbon, nitrogen, sulfur, and phosphorus. These nutrients are used for the synthesis of macromolecules and cellular maintenance. - - Microbes utilize different transport mechanisms, including passive diffusion, facilitated diffusion, and active transport, to acquire these nutrients from their environment. - - Nitrogen fixation in certain bacteria (e.g., Rhizobium) is an example of a unique metabolic process that enables microorganisms to convert atmospheric nitrogen into a usable form, supporting nutrient cycles in ecosystems. - 7. Microbial Adaptations: - Microbes can adapt their metabolism based on available resources and environmental conditions. For example, lactate fermentation is used when oxygen is absent, and aerobic respiration is used when oxygen is present. - - Some microorganisms can switch between different metabolic pathways depending on environmental signals, such as facultative anaerobes that can survive both in the presence and absence of oxygen. - Lesson 2: Profiling of Bacteria Overview of Bacteria Bacteria are single-celled microorganisms that can exist in various shapes such as spherical (cocci), rod-shaped (bacilli), or spiral (spirilla). They are prokaryotic organisms, meaning they lack a nucleus and other membrane-bound organelles. Bacteria can be found in almost every environment on Earth, from soil to water, and even inside the human body. They play important roles in processes like nitrogen fixation, decomposition, and digestion. Some bacteria are pathogenic, causing diseases like tuberculosis, cholera, and pneumonia, while others are beneficial, aiding in digestion or used in the production of food such as yogurt. Gram-Positive and Gram-Negative Bacteria The classification of bacteria into Gram-positive and Gram-negative is based on their cell wall structure, which is determined by the Gram stain procedure. The key differences are: - Gram-Positive Bacteria: - Cell Wall Structure: Thick peptidoglycan layer. - - Staining Reaction: Retain the crystal violet stain, appearing purple under a microscope. - - Examples: Staphylococcus aureus, Streptococcus pneumoniae. - - Gram-Negative Bacteria: - Cell Wall Structure: Thin peptidoglycan layer, but with an additional outer membrane containing lipopolysaccharides. - - Staining Reaction: Do not retain the crystal violet stain; they take up the counterstain (usually safranin) and appear pink. - - Examples: Escherichia coli, Salmonella spp. - The differences in the cell wall affect the bacteria's susceptibility to antibiotics and their ability to cause disease. Antibiotic Resistance Antibiotic resistance occurs when bacteria evolve mechanisms to withstand the effects of drugs that once killed them or inhibited their growth. This can occur naturally through mutation or acquired via horizontal gene transfer (such as through plasmids). Resistance is a significant public health concern because it renders many antibiotics ineffective, making infections harder to treat. Factors contributing to antibiotic resistance include: - Overuse and Misuse of Antibiotics: Taking antibiotics for viral infections, not completing prescribed courses, or using antibiotics in agriculture accelerates resistance. - Bacterial Adaptation: Bacteria can change their genetic material to avoid the action of antibiotics (e.g., by producing enzymes like beta-lactamase to neutralize the drug, or by changing their cell wall structure). - Horizontal Gene Transfer: Resistant bacteria can share resistance genes with other bacteria, rapidly spreading resistance. Antibiotic resistance leads to longer hospital stays, more intensive care, and higher mortality rates. Preventive measures include using antibiotics responsibly, improving infection control practices, and investing in research for new antibiotics. Lesson 3: Control of Microbial Growth and Parasitic Diseases Microbial growth refers to the increase in the number of microorganisms, including bacteria, viruses, fungi, and protozoa. Parasitic diseases are caused by parasites---organisms that live on or in a host and benefit at the host's expense. Controlling microbial growth and parasitic diseases is crucial in maintaining health in humans, animals, and plants. Below is a thorough discussion, including key terms that need to be defined. Microbial Growth Control Microbial growth control can be achieved through various physical, chemical, and biological methods. The aim is to prevent infections, food spoilage, and other health risks posed by microorganisms. 2. Sterilization: The process of completely eliminating or destroying all forms of microbial life, including bacteria, viruses, fungi, and spores. Methods include: - Heat Sterilization: Using high temperatures to kill microorganisms, either through dry heat (oven) or moist heat (autoclaving). - - Chemical Sterilants: Chemicals like formaldehyde or hydrogen peroxide that destroy microbes. - - Filtration: Removing microorganisms from liquids or gases by passing them through a filter with pores small enough to trap them. - 2\. Disinfection: A process that eliminates most pathogens (except for bacterial spores) on inanimate surfaces, such as surfaces in hospitals, public places, or kitchens. Disinfectants include bleach, alcohol, or chlorine compounds. 3\. Antisepsis: The process of reducing the number of microorganisms on living tissues, such as skin. Antiseptics, like iodine or alcohol, are applied to prevent infection, especially before surgery or wound care. 5. Chemotherapy: The use of chemical agents (such as antibiotics or antivirals) to kill or inhibit the growth of microorganisms inside the body. Examples: - Antibiotics: Drugs that inhibit bacterial growth or kill bacteria (e.g., penicillin). - - Antivirals: Drugs that target viruses, reducing their ability to replicate (e.g., acyclovir for herpesvirus). - 6. Physical Control Methods: - Temperature Control: Heat (pasteurization) or cold (refrigeration or freezing) can control microbial growth by slowing or stopping microbial metabolism. - - Radiation: Ultraviolet (UV) radiation or gamma rays are used to destroy microbial DNA, preventing replication. - 7. Antibiotic Resistance: A growing concern where microbes evolve to resist the effects of medications designed to kill them, making infections harder to treat. Proper use of antibiotics, combined with infection control measures, helps mitigate resistance. Parasitic Diseases and Their Control Parasitic diseases are caused by parasites that may be protozoa, helminths (worms), or arthropods. These diseases can spread through contaminated food, water, soil, or through vectors like mosquitoes. 3. Protozoal Diseases: - Malaria: Caused by Plasmodium protozoa, transmitted by the Anopheles mosquito. It can be controlled by using insecticide-treated bed nets, indoor spraying of insecticides, and antimalarial drugs. - - Amoebiasis: Caused by Entamoeba histolytica, affecting the intestines. Control involves improved sanitation, water treatment, and the use of medications like metronidazole. - 2\. Helminthic Diseases: - Ascariasis: Caused by Ascaris lumbricoides, a roundworm. It is controlled by improving sanitation, providing deworming treatments, and promoting hand hygiene. - - Schistosomiasis: Caused by blood flukes, primarily Schistosoma species. Control measures include providing safe water sources, snail control, and mass drug administration with praziquantel. - 3\. Arthropod-Borne Diseases: - Lymphatic Filariasis (Elephantiasis): Caused by filarial worms, transmitted by mosquitoes. Control involves mass drug administration with antifilarial medications like diethylcarbamazine and vector control programs to reduce mosquito populations. - 4\. Prevention and Treatment of Parasitic Diseases: - Vector Control: The use of insecticides, bed nets, and environmental management to reduce the populations of vectors (e.g., mosquitoes, tsetse flies) that transmit parasitic diseases. - - Sanitation and Hygiene: Proper sanitation, waste disposal, and clean drinking water are critical in preventing the transmission of parasitic diseases, especially those caused by protozoa and helminths. - - Vaccination: For some parasitic diseases like malaria, vaccines are being developed and tested. For example, the RTS,S/AS01 malaria vaccine is designed to reduce the burden of malaria. - 6. Antiparasitic Drugs: - Antimalarials: Drugs such as chloroquine, quinine, and artemisinin are used to treat malaria. - - Anthelmintics: Medications like albendazole and mebendazole are used to treat infections caused by helminths. - - Anti-Protozoal Drugs: For protozoal infections, drugs like metronidazole (for amoebiasis) or sulfonamides (for toxoplasmosis) are used. - 7. Vaccines: Vaccines like the RTS,S malaria vaccine and the schistosomiasis vaccine are in development and have been implemented in specific regions. Key Terms to Define: - Sterilization: A process that eliminates or destroys all forms of microbial life. - Disinfection: The elimination of most pathogenic microorganisms from inanimate objects or surfaces. - Antisepsis: The practice of reducing the number of microorganisms on living tissue. - Antibiotics: Drugs used to treat bacterial infections by killing or inhibiting bacterial growth. - Parasites: Organisms that live on or in a host organism and derive nutrients at the host's expense. - Protozoa: Single-celled organisms that can cause diseases such as malaria and amoebiasis. - Helminths: Parasitic worms that include roundworms, flatworms, and tapeworms. - Vector: An organism, such as a mosquito or tick, that transmits a pathogen or parasite to a host. - Anthelmintics: Drugs used to treat infections caused by helminths. - Mass Drug Administration (MDA): The distribution of medications to entire populations to control or eliminate parasitic disease Lesson 4: Virology Virology is the branch of microbiology that deals with the study of viruses and viral diseases. It involves understanding the structure, classification, replication, and life cycles of viruses, as well as their interactions with host cells. Virology also explores the impact of viruses on living organisms, including humans, animals, and plants, and examines the development of vaccines, antiviral drugs, and other preventive measures to combat viral infections. a. Structure and Classification of Viruses - Structure: Viruses are composed of: 4. Genetic Material: This can be either DNA or RNA, and it contains the instructions for making new viral particles. It can be single-stranded or double-stranded, linear or circular. 5. Capsid: A protein coat that encases the genetic material. The capsid is made of protein subunits called capsomers. 6. Envelope (optional): Some viruses have an outer lipid membrane derived from the host cell membrane, which may contain glycoproteins that help the virus infect host cells. - Classification: Viruses are classified based on several criteria: 1. Nature of Genetic Material (DNA or RNA) 2. Symmetry of the Capsid (icosahedral, helical, complex) 3. Presence of Envelope 4. Host Range (e.g., animal viruses, plant viruses, bacteriophages) The Baltimore Classification System is commonly used, which divides viruses into seven groups based on their genome type and replication strategy. b. Viral Replication and Life Cycles Viral replication involves several stages: 1. Attachment: The virus attaches to a specific receptor on the host cell surface. 2. Penetration: The virus or its genome enters the host cell through fusion or endocytosis. 3. Uncoating: The viral capsid is broken down, releasing the viral genome into the host cell. 4. Replication and Transcription: The host cell machinery is hijacked to replicate the viral genome and transcribe viral mRNA. 5. Translation: Viral proteins are synthesized using the host's ribosomes. 6. Assembly: New viral genomes and proteins are assembled into new virions. 7. Budding or Lysis: New virions are released from the host cell, often destroying the host cell (lysis) or via budding to avoid immediate cell death. Types of Life Cycles: 1. Lytic Cycle: The virus hijacks the host's machinery to replicate and causes the host cell to burst, releasing new virions. 2. Lysogenic Cycle: The viral genome integrates into the host's genome (provirus), remaining dormant until triggered to enter the lytic cycle. c. Viral Diseases and Vaccines - Viral Diseases: - HIV/AIDS: Caused by the human immunodeficiency virus, leading to immune system dysfunction. - - Influenza: Caused by the influenza virus, it leads to respiratory illness and can be severe. - - COVID-19: Caused by the SARS-CoV-2 virus, leading to respiratory illness and a global pandemic. - - Chickenpox: Caused by the varicella-zoster virus, leading to skin rashes and fever. - - Hepatitis: Caused by various hepatitis viruses (A, B, C), affecting the liver. - - Vaccines: Vaccines are designed to stimulate the immune system to recognize and fight specific viruses. Some examples include: - MMR Vaccine: Protects against measles, mumps, and rubella. - - Hepatitis B Vaccine: Protects against hepatitis B infection. - - Polio Vaccine: Protects against poliovirus. - - Influenza Vaccine: Provides immunity against the seasonal flu. - - COVID-19 Vaccines: Protect against SARS-CoV-2. - Vaccines work by introducing a weakened or inactivated form of the virus, or viral proteins, to trigger the body's immune response without causing the disease. Some key terms related to Virology along with their definitions: 3. Virus: A microscopic infectious agent that can only replicate within the living cells of a host organism. It consists of genetic material (DNA or RNA) enclosed in a protein coat called a capsid, and sometimes an outer lipid envelope. 4. Capsid: The protein shell that surrounds the viral genome. It is made up of protein subunits called capsomers and protects the viral genetic material. 5. Envelope: A lipid membrane derived from the host cell membrane that surrounds the capsid of some viruses. It may contain viral glycoproteins that are important for binding to host cells. 6. Genome: The genetic material of the virus, which can be either DNA or RNA. It contains the information necessary for viral replication and assembly. 7. Bacteriophage: A type of virus that specifically infects bacteria. 8. Lytic Cycle: The cycle of viral replication in which the virus causes the host cell to burst (lysis) after producing new viral particles, releasing them to infect other cells. 9. Lysogenic Cycle: A viral replication cycle in which the viral genome integrates into the host cell's genome and remains dormant (provirus) until triggered to enter the lytic cycle. 10. Attachment: The initial step of viral infection where the virus binds to specific receptors on the surface of the host cell. 11. Penetration: The process by which the virus or its genetic material enters the host cell, typically through fusion with the cell membrane or endocytosis. 12. Uncoating: The process by which the viral capsid is removed inside the host cell, releasing the viral genome for replication and transcription. 13. Replicase: An enzyme that helps in the replication of the viral genome. 14. Reverse Transcription: A process in which the RNA genome of retroviruses, like HIV, is converted into DNA by the enzyme reverse transcriptase, allowing it to integrate into the host genome. 15. Viral Load: The quantity of virus particles in a given volume of blood or other bodily fluid. It is often used as an indicator of the severity of an infection. 16. Vaccination: The process of administering a vaccine to stimulate the immune system to recognize and fight a specific pathogen, such as a virus. 17. Immunization: The process by which an individual becomes protected against a virus, either through natural infection or vaccination. 16\. Antibody: A protein produced by the immune system in response to an antigen (like