Respirology Week 8.pptx
Document Details
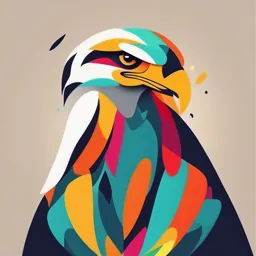
Uploaded by IndebtedPlum
Full Transcript
BMS 250 – Respirology 4 Viral and Fungal Lung Infections Pleural Disease Catchup BMS 250 Outcomes Briefly describe the pathogenesis, basic clinical manifestations, and diagnosis for the following pleural disease entities: Pneumothorax – different varieties Pleural effusion, parapneumonic effusion, a...
BMS 250 – Respirology 4 Viral and Fungal Lung Infections Pleural Disease Catchup BMS 250 Outcomes Briefly describe the pathogenesis, basic clinical manifestations, and diagnosis for the following pleural disease entities: Pneumothorax – different varieties Pleural effusion, parapneumonic effusion, and empyema Briefly describe the biology, major virulence factors, diagnosis, and clinical manifestations of infection for the following: clinically important influenza strains RSV, SARS-1, SARS-2, MERS, and Hanta virus rhinovirus, parainfluenza virus, metapneumovirus* Aspergillus species, Histoplasma capsulatum, Cryptococcus, Pneumocystis jirovecii, Nocardiosis* Atelectasis Atelectasis is a medical condition characterized by the collapse or incomplete expansion of a part or all of the lung. This collapse can occur due to various reasons, including blockage of the airways, pressure from outside the lung, or weakened lung tissue Atelectasis = collapse of the airspaces – mostly referring to gas exchange areas Major types include: ▪ collapse of previously healthy lungs (“acquired”) obstruction of an airway resorption atelectasis compression from something outside of the lungs (usually in the pleural space) compression atelectasis fibrosis or the lungs or pleura leads to reduced compliance contraction atelectasis This type of atelectasis occurs due to scarring or fibrosis within the lung tissue, which prevents proper expansion of the affected area. ▪ failure of neonatal lungs to expand, usually due to inadequate production of surfactant, could be considered a type of contraction atelectasis Atelectasis Atelectasis is not a disease, but it increases the risk for: ▪ Pulmonary infection (pneumonia) ▪ Ventilationperfusion mismatching Contraction atelectasis can be the underlying cause for restrictive pulmonary diseases Resorption Atelectasis This type occurs when the airway leading to a portion of the lung is blocked, preventing air from reaching the alveoli (air sacs) within that area. As a result, the alveoli collapse due to the absorption of air by surrounding tissues. As you reduce ventilation to an airway, any air that is “left” in the gas-exchanging areas will diffuse into the bloodstream ▪ eventually the airway/alveoli will collapse ▪ Major causes: obstruction: ▪ aspiration of a foreign body blocking a conducting airway ▪ tumour or other growth (granuloma, etc.) ▪ a mucous plug (asthma, chronic bronchitis, bronchiectasis) Resorption Atelectasis Major causes cont… ▪ a special case – think of what happens if you reduce tidal volume over long periods of time dependent (lower) airways have poorer ventilation, and are much more likely to collapse ▪ tidal volume can be reduced by reduced respiratory drive (i.e. morphine analogues) or by pain (i.e. abdominal incision) or just by lying around and general weakness in some (hospitalization) incentive spirometry can help prevent Compression Atelectasis Compression atelectasis happens when there is external pressure on the lung, preventing it from expanding fully. This pressure can be caused by a tumor, enlarged lymph nodes, fluid accumulation in the pleural cavity (pleural effusion), or even external forces such as a pneumothorax (collapsed lung) Normal pleura The pleura is a thin, double-layered membrane that surrounds the lungs and lines the chest cavity. It plays a crucial role in protecting and supporting the lungs during breathing. The pleura consists of two layers: Visceral Pleura:This inner layer is directly attached to the surface of the lungs, covering and adhering to their contours. Parietal Pleura: The outer layer lines the inner surface of the chest wall, diaphragm, and mediastinum (the area between the lungs). The space between the visceral and parietal pleura is called the pleural cavity. Normally, this cavity contains a small amount of lubricating fluid known as pleural fluid, which helps reduce friction between the two layers during breathing movements. Normal pleura The pleura serves several functions: Protection: It provides a protective barrier around the lungs, shielding them from friction and damage against the chest wall and other surrounding structures. Lubrication:The pleural fluid within the pleural cavity acts as a lubricant, allowing smooth movement of the lungs during breathing. Support: The pleura helps maintain the shape and structure of the lungs, aiding in their expansion and contraction during respiration. Disorders affecting the pleura can include pleurisy (inflammation of the pleura), pleural effusion (accumulation of excess fluid in the pleural cavity), pneumothorax (presence of air in the pleural space, causing lung collapse), and pleural thickening (abnormal thickening and scarring of the pleural membranes). Normal pleura Pleural fluid is a clear, watery fluid that fills the space between the two layers of the pleura – the visceral and parietal pleura. Normally, only a small amount of pleural fluid is present in the pleural cavity, serving to lubricate the pleural surfaces and facilitate smooth movement of the lungs during breathing. However, various medical conditions can disrupt the balance of pleural fluid production and absorption, leading to an abnormal accumulation of fluid in the pleural cavity. This excess fluid accumulation is referred to as pleural effusion. The mesothelium is a specialized type of epithelial tissue that lines the body cavities and covers the organs within these cavities. It consists of a single layer of flattened to cuboidal cells known as mesothelial cells. The primary function of the mesothelium is to provide a smooth, protective surface that allows organs to move against one another with minimal friction. Normally, Between 10 – 25 ml of pleural fluid (0.13 – 0.3 ml/kg body weight) ▪ mesothelium – parietal and visceral epithelium on both sides specialized for fluid transport (numerous microvilli) ▪ visceral layer is relatively insensitive, but the parietal is innervated by intercostal nerves and phrenic nerve (diaphragmatic areas) and tends to cause sharp chest pain when stimulated (pleuritic pain) Fluid is continually turned over – you completely replace your pleural fluid ~ 12 X/day ▪ the lymphatics can accommodate 10 – 20X normal hourly production without accumulating significant volumes of fluid in the pleural space drains into the thoracic duct, which can sometimes be obstructed by tumours, trauma fluid accumulates rapidly after “drainage reserve” is overwhelmed Normal pleura Normal intrapleural pressure is around -10 cm water at the lung bases caused by elastic recoil, surface tension, and constant suction of lymphatic drainage The two surfaces of the pleura never touch, even though the fluid layer between them is incredibly thin the negative charges on the Diseases of the pleura definitions Most diseases that affect the pleura are secondary to another underlying disorder – these can be intra- or extra-pulmonary ▪ cancer, pneumonia, non-infectious inflammation, and elevated pulmonary vascular pressures can all cause pleural effusions pleural effusion = an abnormal collection of fluid in the pleural space can be caused by pleuritis = inflammation of the pleural surface (more later) ▪ trauma and obstructive lung disease can cause a pneumothorax pneumothrax = a collection of air (always abnormal) in the pleural space Diseases of the pleura definitions Other causes of fluid in the pleural space: ▪ hemothorax = a usually large collection of blood in the pleural space due to trauma, vascular rupture, or both ▪ hemorrhagic pleuritis = a sero-sanguinous collection of blood and other inflammatory fluid (leukocytes, proteins that are found in a serous exudate) ▪ empyema = purulent inflammation within the pleural space often becomes organized into adhesions that extend from the visceral pleura to the parietal pleura Pleural effusions Two major types – transudative and exudative ▪ transudate = protein- and cell-poor fluid that accumulates due to imbalance of Starling forces (congestive heart failure, cirrhosis, nephrotic syndrome, constrictive pericarditis, atelectasis) ▪ can also be caused by excess fluid in the peritoneal cavity that “leaks up” through small defects in the diaphragm peritoneal dialysis, ascites Pleural effusions Exudative pleural effusions are rich in protein and often cells and have a wider range of causes ▪ also imperative that the cause be identified, as the lungs are the most common site of metastasis for any malignancy Causes of exudative pleural effusions: ▪ Malignant – mesothelioma, lung cancer, or metastases from a distant site ▪ Inflammatory – lupus and RA occasionally cause fibrosing parenchymal disease and pleural effusion/disease, sarcoidosis ▪ Infectious – parapneumonic effusion causes (next slide), viral infections, granuloma-forming infections ▪ Vascular – PULMONARY EMBOLI, occasionally lunginvolving vasculitides ▪ Drugs… there are a million of them ▪ Metabolic/Other – uremia is the biggest one here, radiation Frequency of pleural effusion etiologies Don’t’ look at the last slide… Which ones are exudative? transudative? Infectious pleural effusions Parapneumonic effusion = is an accumulation of fluid in the pleural space (the space between the lungs and the chest wall) that occurs as a complication of pneumonia. It typically arises when the infection spreads to the pleura, causing inflammation and fluid buildup. If left untreated, a parapneumonic effusion can progress to become an empyema, which is a collection of pus within the pleural cavity. effusion that occurs during infectious (usually bacterial, but any pneumonia can cause one) pneumonia (common, 40% of bacterial pneumonias) Stages: ▪ exudative phase – sterile fluid rich in protein, from pulmonary interstitial space due to increased pleural and vascular permeability - occurs 2-5 days from onset ▪ fibrinopurulent stage – bacterial invasion of pleural space accompanied by increased neutrophils (pus) – occurs 5-10 days from onset ▪ organization stage – fibroblasts grow into the exudates from visceral and parietal pleura – membrane is inelastic and can prevent inflation Parapneumonic effusion Left pleural effusion developed 4 days after antibiotic treatment for pneumococcal pneumonia. Patient developed fever, leftsided chest pain, and increasing dyspnea. During thoracentesis, purulent pleural fluid was removed, and the Gram stain showed gram-positive diplococci. The culture confirmed this to be Streptococcus pneumoniae. Infectious pleural effusions Three main types: ▪ uncomplicated – exudate with neutrophils, no microbes found on thoracocentesis – these don’t need to be drained, just need antibiotics ▪ complicated – bacteria invade but are cleared rapidly (may not find them during investigating fluid), more neutrophils/protein/cellular debris – the pleural fluid needs to be drained otherwise it takes longer to resolve and there’s a risk of chronic pleural complications 10% off all parapneumonic effusions become complicated ▪ empyema – pus (lots of microbes, neutrophils, other leukocytes) and may need drainage or even more invasive therapy 70% of the time due to bacterial pneumonia Pleural effusions – Clinical Features Signs and symptoms of the underlying illness? Symptoms caused by the effusion itself: ▪ dyspnea – caused by compression of the alveolar space due to enlargement of the pleural space, initially a complication of unresolved inflammation can be fibrosis of the pleura and adhesion formation = restrictive disease ▪ Chest pain – typically well localized, sharp pain worsened by cough or inspiration, can be referred to shoulder or upper abdomen if effusion is around the diaphragm larger effusions may cause less pain as the irritation to the parietal pleura is reduced (lung no longer rubs on it) Pleural Effusions – Clinical Features A couple of pearls: ▪ if, post anti-biotic treatment of pneumonia, the fever and the illness in general is not getting better, suspect an effusion ▪ “the sun cannot set on a parapneumonic effusion” ▪ a mnemonic used in medicine to emphasize the urgency of diagnosing and treating parapneumonic effusions promptly. It highlights the importance of timely intervention to prevent potential complications associated with this condition.The phrase suggests that action should be taken before the end of the day ("sunset") to address the parapneumonic effusion. late treatment of serious infectious effusions (i.e. ones that are big, or complicated, or definitely an empyema) can threaten a patient’s life and future lung function ▪ Transudates don’t necessarily need a lot of investigation… you always have to make sure an exudate is not cancer, though ▪ Transudates tend to be bilateral, exudates can be uni- or Pleural effusions diagnosis Chest radiograph is a decent initial study, both upright and decubitus Ultrasound is better than Xray for detecting effusions, and CT is better than ultrasound, likely Thoracocentesis can be diagnostic and therapeutic ▪ and then there’s a bunch of fancy stuff – can cause a pneumothorax and put a video camera into the enlarged pleural space to take a look Pleural Effusions Treatment & Prognosis ▪ Depends on the cause – malignancies that have metastasized to the pleura usually have a very poor prognosis ▪ Ineffectively treated parapneumonic effusions that progress to empyema entail a higher mortality and morbidity ▪ Treated by draining the fluid, and sometimes by putting in a mixture or clot-busting drugs (that’s right, just like a STEMI) and DNA-ase enzymes to break up loculations and improve drainage Influenza Two meanings to “influenza”: ▪ Syndrome systemic symptoms of fever, malaise, myalgia – usually in combination with respiratory symptoms ▪ Respiratory symptoms include dyspnea, cough (lower) and URTI symptoms like rhinitis, sinusitis, or otitis When the syndrome is referred to, systemic symptoms are present ▪ Microbe Influenza virus – Seems to be able to infect almost anywhere in the respiratory tract: ▪ Rhinitis, sinusitis, otitis, tracheobronchitis, pneumonia The microbe doesn’t always cause systemic symptoms Influenza – General Microbiology Two most clinically important types are influenza A and influenza B – both are orthomyxoviruses ▪ Both are negative-sense single-stranded RNA viruses that are surrounded by a lipid membrane bilayer derived from the host cell membrane ▪ Negative-sense RNA virus the viral RNA can’t be read directly by the host cell’s ribosome RNA-dependent RNA polymerase (produced by the virus) is necessary to convert the RNA genome into a form that can be read by the host’s ribosome ▪ Major clinically relevant proteins for influenza: Neuraminidase Viral hemagglutinin RNA-dependent RNA polymerase proteins Others - dependent on type Influenza – General Microbiology Hemagglutinin spike - allows virus to bind to and invade the host cell ▪ binds to sialic acid-containing glycolipids or glycoproteins on respiratory cells Neuraminadase spike – allows the virus to disengage from the cell and spread ▪ Cleaves where the hemagglutinin spike binds Influenza – General Microbiology PB protein – RNAdependent RNA polymerase ▪ Transported into the nucleus where viral mRNAs are produced “Other” proteins ▪ Influenza A – M2, influenza B – NB Both of these proteins act as ion channels ▪ Influenza A produces proteins that can block alpha interferon effects Life cycle of influenz a A and B Binding due to hemagglutini n Budding due to neuraminidas e Pathogenesis of Influenza The pathogenesis of influenza, caused by influenza viruses, involves a complex interplay between the virus and the host's immune response. Viral Entry and Replication: Influenza viruses primarily infect the respiratory epithelium, including the cells lining the nose, throat, and lungs. The virus enters the host's respiratory tract through inhalation of respiratory droplets containing the virus, direct contact with infected individuals, or contact with contaminated surfaces. Once inside the respiratory tract, the virus attaches to and enters epithelial cells via interactions between viral surface glycoproteins (hemagglutinin and neuraminidase) and host cell receptors. Viral Replication and Spread: Once inside the host cell, the viral RNA is released into the cytoplasm, where it serves as a template for viral RNA synthesis and replication. The virus hijacks the host cell's machinery to produce new viral proteins and assemble new virus particles. Infected cells then release newly formed virus particles, which can infect neighboring cells and spread further within the respiratory tract. health conditions, the infection can progress to more severe complications, such as bacterial pneumonia, exacerbation of chronic respiratory conditions, or systemic complications. Pathogenesis of Influenza Immune Response:The host's immune system responds to the viral infection by activating innate and adaptive immune mechanisms. Innate immune cells, such as macrophages and dendritic cells, detect the presence of the virus and release pro-inflammatory cytokines and chemokines to recruit immune cells to the site of infection. Adaptive immune responses, involving T cells and B cells, are also activated to target and eliminate virus-infected cells and produce antibodies against the virus. Inflammation and Tissue Damage: The immune response to influenza infection can lead to inflammation and tissue damage within the respiratory tract. Excessive inflammation and cytokine release, known as a cytokine storm, can contribute to the severity of symptoms and complications associated with influenza infection, such as pneumonia and acute respiratory distress syndrome (ARDS). Resolution or Complications: In most cases, the host's immune response is able to control and eliminate the virus, leading to recovery from influenza illness within a week or two. However, in some individuals, particularly those with weakened immune systems or underlying health conditions, the infection can progress to more severe complications, such as bacterial pneumonia, exacerbation of chronic respiratory conditions, or systemic complications. Overall, the pathogenesis of influenza involves a dynamic interaction between the virus and the host's immune response, with the balance between viral replication and immune control determining the outcome of the infection. Pathogenesis of Influenza Replicates in upper respiratory tract epithelial cells, then later migrates to lower respiratory tract epithelial cells ▪ Cleavage of the hemagglutinin receptor from sialic acid seems to only happen in respiratory epithelium Destruction of the ciliated epithelium predisposes to later development of bacterial pneumonia ▪ May take 2 – 10 weeks for respiratory epithelial cells to recover fully ▪ May have been responsible for many of the deaths seen in serious pandemics such as the Spanish flu Pathogenesis of Influenza Initial immune response is macrophagemediated and can be associated with a severe cytokine storm ▪ Increased production of typical acute proinflammatory cytokines shock and noncardiogenic pulmonary edema Eventually the virus is controlled/eradicated – likely through activation of cytotoxic T cells ▪ Abs may be helpful in clearing the virus, but are likely very important for later immunity Comparison of Influenza Types Feature Unique Proteins Reservoir Disease severity Influenza A M2 Many – swine, marine mammals, bats, avians, humans, equines Often severe Epidemic potential Epidemics and pandemics Mutation capability Antigenic drift & antigenic shift Influenza B NB Humans, seals Influenza C HEF Humans, swine Occasionally Usually mild severe Occasional Limited outbreaks epidemics & outbreaks Antigenic drift only Antigenic drift only Influenza – mutation capabilities Antigenic shift and antigenic drift are two processes by which influenza viruses undergo genetic changes, leading to the emergence of new strains with altered antigenic properties. These changes can affect the ability of the virus to infect hosts and evade immunity. Influenza – mutation capabilities Antigenic shift = large changes in RNA sequence ▪ Usually due to circulation in large animal host reservoirs ▪ Whole genes are “swapped” between virus strains ▪ Increases likelihood that previously-formed antibodies/adaptive immunity will be ineffective in protecting the host (large changes in spike proteins loss of antibody binding) ▪ Antigenic shift only occurs in influenza A – thought to be main reason why it is the more virulent strain with more severe clinical features Influenza – mutation capabilities Antigenic Shift: More Detail Antigenic shift refers to a sudden and major change in the antigenic properties of the influenza virus. It occurs when two or more different influenza viruses infect the same host cell and exchange genetic material, resulting in the creation of a novel influenza virus with a unique combination of surface proteins. Typically, antigenic shift events involve influenza viruses from different species, such as humans, birds, and pigs. When a reassortment event occurs between human and animal influenza viruses, it can lead to the emergence of a new influenza subtype capable of causing pandemics. Antigenic shift events are relatively rare but have significant public health implications due to the potential for the emergence of novel influenza strains against which the population has little to no immunity. Influenza – mutation capabilities Antigenic drift = smaller, point-mutation type changes in RNA genome smaller deterioration in Ab effectiveness RNA-dependent RNA polymerase has a high error rate (no proof-reading) Influenza – mutation capabilities Antigenic Drift: More Detail Antigenic drift refers to gradual and incremental changes in the antigenic properties of the influenza virus over time. It occurs due to mutations that accumulate in the viral genome, particularly in genes encoding the surface glycoproteins hemagglutinin (HA) and neuraminidase (NA). Antigenic drift leads to the emergence of new variants or strains of influenza virus within the same subtype. These variants may have altered antigenic properties compared to previously circulating strains, allowing them to evade preexisting immunity to some extent. Antigenic drift is responsible for the seasonal influenza epidemics that occur each year, as the virus undergoes continuous evolution to evade host immune responses. Although antigenic drift results in changes to the virus, it typically does not lead to the emergence of entirely new subtypes or pandemics, as seen with antigenic shift. Influenza A - naming “H” refers to hemagglutinin type ▪ 18 subtypes of hemagglutinin “N” refers to neuraminidase type ▪ 11 subtypes of neuraminidase Other naming conventions refer to specific genetic differences that are more minor (drifts) ▪ Examples: two H3N2 strains that differ antigenically only slightly are A/Texas/1/77(H3N2) and A/Bangkok/1/79(H3N2) In humans the most relevant subtypes are: ▪ H1, H2, H3 and N1, N2 (most common) ▪ H5 – H7, H9, H10 and N6 – N9 (less common, but some can cause severe disease) Birds (in particular aquatic birds) seem to host all or most subtypes Influenza severity Severity of epidemics/pandemics depends on: ▪ Antibody effectiveness ▪ Ability to be passed from human – human ▪ Other virulence factors Example – H5N1 (“avian flu”) ▪ Over past several years, 700 cases reported ▪ Often affects younger adults… and can have a massive mortality (60%!!!) ▪ However, since it is very poorly transmitted from person to person, no massive epidemics or pandemics Example 2 – (H1N1)pdm09 ▪ 60.8 million cases in US from 2009 – 2010, 274,000 hospitalizations, 12,500 deaths (most in young adults) ▪ Global cases could have been as high as 1 billion ▪ Easily transmitted human - human 1918-1919 1957-1958 1968-1969 1977-1978 2009-2010 (the Spanish flu) (the Asian flu) (Hong Kong flu) (Russian flu) (Swine flu) Shifting and drifting impacts Periodic shifts in major antigenic components result in major epidemics ▪ populations with little immunologic experience with the subtype As the population of susceptible individuals is exhausted the subtype circulates for a time, undergoing mutations with subtle antigenic drifts from season to season ▪ subtype-specific immunity is acquired by more people. ▪ allows transmission to continue - infectivity persists because subtype-specific immunity is not fully protective against drifting ▪ Example: may have antibodies reasonably protective against influenza A/Texas/77(H3N2), yet be susceptible in succeeding years to reinfection by influenza A/Bangkok/79(H3N2) Eventually the overall immunity of the population becomes sufficient to minimize the epidemic potential of the major subtype and its drifting strains Influenza – general microbiology Direct droplet transmission, usually (coughing) In temperate areas, transmission/infection is usually December – early March human influenza viruses are not stable in the environment and are sensitive to heat, acid pH, and solvents (i.e. H1-3, N12) ▪ Some avian influenza viruses (H5N1 and others) retain infectivity for several weeks outside the host Can be shed in respiratory secretions and feces, with prolonged survival in feces Influenza – Clinical Features Primarily a respiratory illness causing cough, sore throat and rhinorrhea, or nasal congestion ▪ Cough is typically non-productive ▪ Systemic symptoms include fatigue, myalgias, shaking chills, headache ▪ Sudden onset – symptoms develop over hours with a brief incubation period that averages 2 days (1-4) Usually improves/resolves in less than a week ▪ Can have long-lasting cough, fatigue, weakness and malaise that can continue for a Influenza - Complications Progressive infection, which can be fatal Bacterial superinfection more severe pneumonia ▪ Most common and severe complication – Strep pneumo, H. influenzae, or Staph. Aureus pneumonias Systemic involvement ▪ Viral particles and cytokine release can be toxic to a range of organ systems Can include severe myositis, myocarditis Frequency of heart attacks increase ▪ Can be associated with development of GuillainBarre syndrome and viral encephalitis/meningoencephalitis ▪ In children can be associated with Reye syndrome Fulminant hepatic failure, cerebral edema worsened by administration of salicylate drugs (why kids should never be given aspirin) Diagnosis & Treatment Diagnosis is usually clinical ▪ NAAT tests for confirmation (nasopharyngeal swab) ▪ Serology not used for diagnosis, but for epidemiological data ▪ Chest X-ray, other labs/imaging rule in/out other causes of cough or dyspnea Characterize severity General Treatment ▪ Vaccinations have a modest effect in reducing influenza symptoms, hospitalizations, or days off work in healthy adults ▪ Neuraminidase inhibitors for those with severe disease or at higher risk – benefits if given within 48 hours of onset The “Influenza Pyramid” US numbers: 2017 – 2018 flu season Most hospitalizations /mortality occurs in: elderly, children, pregnant women, immunocompromis ed patients asthma, COPD, CVD, DM, and obesity Comparison – COVID, Flu, Pneumonia mortality COVID-19 – General Microbiology Caused by SARS-CoV-2, a betacoronavirus ▪ Betacoronaviruses include SARS-CoV-1, MERS (very severe variants) which are included as “lineage B” coronaviruses ▪ “Lineage A” coronaviruses include those that cause the common cold Enveloped, positive-sense RNA viruses encoded by a viral RNA genome that is quite large ▪ single linear RNA segment of nearly 30,000 nucleotides ▪ encodes four structural proteins: S (spike), E (envelope), M (membrane), and N (nucleocapsid) proteins ▪ Replicates in the cytoplasm COVID-19 – General Microbiology single-stranded, positive-sense RNA genome bound to a nucleoprotein (helical nucleocapsid) surrounded by a lipid bilayer envelope Spikes project from the surface of the envelope giving the appearance of a crown of thorns or a solar corona. several other surface proteins, including hemagglutininacetylesterase glycoprotein, membrane glycoprotein, and small envelope glycoprotein COVID-19 – General Microbiology Transmission is via: ▪ Droplet transmission – include larger droplets that fall to the ground within seconds – minutes Smaller droplets (aerosols) that remain airborne for much longer ▪ Contact from colonized surface (hands, inanimate objects) to respiratory tract/eyes Targets for viral colonization/replication: ▪ Nasopharyngeal/oropharyngeal cells initially ▪ Bronchial epithelium, alveolar epithelial cells, vascular endothelial cells, and alveolar macrophages can all be invaded/colonized by SARS-CoV-2 ▪ Vascular endothelial cells can include those that are present in the heart and kidney ▪ ACE-2 is also expressed by enterocytes, cholangiocytes, myocardial cells, kidney cells, and bladder urothelial cells COVID-19 – General Microbiology Why is COVID-19 so transmissible? ▪ It replicates in the upper airways Both symptomatic and pre-symptomatic stages SARS-CoV-1 and MERS don’t do that… thank goodness ▪ Both of these coronavirus illnesses have much higher death rates ▪ R0 rate is between 5 and 6 (influenza is between 1 and 2) ▪ Does enteric replication have an impact on transmissibility? No one is sure (as of my last reading) – likely not? Enteric replication does seem to have an impact in exacerbating inflammation during the COVID-19 – General Microbiology Spike protein has a receptor binding domain that binds to the ACE2 enzyme on cells ▪ Cleavage of the spike protein by TMPRSS2 (transmembrane protease serine 2) allows viral entry ▪ Endosome forms after cleavage of the spike protein invasion viral genome is released in the cytoplasm translation in a polyprotein cleavage by the host and viral proteases Proteins can then perform a variety of functions, including ▪ Continued replication (RNA-dependent RNA polymerase) Relatively low rate of mutation (compared to influenza) due to exonuclease activity ▪ Viral particle assembly ▪ Inhibition of type I interferons Cytokine storm generalities Highly virulent viruses bird flu virus (H5N1) of 2006 or swine flu virus (H1N1) of 2009, COVID-19 Infected patients develop acute respiratory distress syndrome (ARDS) caused by a cytokine storm of a healthy, competent, and robust immune system Interferon-γ and other proinflammatory cytokines (mainly TNF-α, IL-1, and IL-6) are secreted that stimulate multiple organ systems Rapidly proliferating and highly activated T cells or natural killer cells, which are activated by infected macrophages. Early lung inflammation (A) In the early stage of disease, SARS-CoV2 infects the bronchial epithelial cells as well as type I and type II alveolar pneumocytes and capillary endothelial cells. The serine protease TMPRSS2 promotes viral uptake by cleaving ACE2 and activating the SARSCoV-2 S-protein ▪ During early infection, viral copy numbers can be high in the lower respiratory tract Early lung inflammation Inflammatory signaling molecules are released by infected cells and alveolar macrophages, in addition to recruited T lymphocytes, monocytes, and neutrophils. Late lung inflammation With disease progression, plasma and tissue kallikreins release kinins that activate kinin receptors on the lung endothelium leads to vascular smooth muscle relaxation and increased vascular permeability. This process is controlled by the ACE2 receptor. Without ACE2 blocking the ligands of kinin receptor B1, the lungs are prone to vascular leakage, angioedema, and downstream activation of coagulation. Late lung inflammation Dysregulated proinflammatory cytokine (TNF, IL-1, IL-6) and NO release and signalling contribute to these processes. As a consequence, pulmonary oedema fills the alveolar spaces, followed by hyaline membrane formation, compatible with earlyphase acute respiratory distress syndrome Anomalous coagulation frequently results in the formation of microthrombi and subsequent thrombotic sequelae How is the renin-angiotensin-aldosterone system involved? COVID-19 - Immunity Both cell-mediated and humoral immunity play important roles in acute SARS-CoV-2 infection ▪ Studies suggest that T cell responses may be important in controlling infection, whereas antibodies may provide longer protection ▪ T cell responses were higher in mild COVID-19 patients compared with moderate to severe patients ▪ COVID-19 patients with moderate to severe disease had more robust antibody responses than patients with mild disease – therefore it’s thought that antibodies may not reduce disease severity once the infection has set in More research is needed to determine: ▪ the duration of immunity and long-term protection ▪ cellular vs. humoral immune responses in recovered COVID-19 patients or vaccinated individuals COVID-19 – Clinical Features Incubation period of 2 to 14 (median 5-6) days ▪ Symptoms develop within 11.5 days and in some cases 5 to 6 days ▪ Approximately 30% remain asymptomatic 80% of the people who develop symptoms will have mild to moderate findings - include (several of these but not all): ▪ fever or chills ▪ Cough (can be productive), shortness of breath, fatigue ▪ muscle or body aches, headache ▪ loss of taste or smell (10%), sore throat, congestion or runny nose, conjunctivitis ▪ nausea or vomiting, diarrhea COVID-19 – Clinical Features More severe symptoms include: ▪ Dyspnea (severe), cyanosis ▪ Chest pain (could be angina) ▪ Confusion, inability to wake or stay awake COVID viral pneumonia causes impressive hypoxemia when it progresses from ALI ARDS (15%) ▪ In those that develop lower respiratory symptoms, 15% will require some degree of oxygen support 5% will have some degree of septic shock or multi-organ failure ▪ Unique to COVID-19 – development of significant hypoxemia (oxygen saturation < 90%) but limited dyspnea and respiratory discomfort Theory – COVID-19 decreases sensitivity of the oxygen-sensing chemoreceptors? COVID-19 - Complications Death (obviously) ▪ Death toll to date is approximately 7 million worldwide (most due to pneumonia) ▪ Mortality rate is estimated at just over 2% Heart attack & myocarditis Cerebrovascular disease ▪ Ischemic stroke due to large or small vessel occlusion due to hypercoagulability Acute kidney injury (aka acute renal failure) Risk factors for severe complications and viral pneumonia include: ▪ Advanced age (>80% of deaths occur in people over age 65) ▪ Male sex, racial and ethnic minorities ▪ People with chronic conditions (cardiovascular disease, chronic kidney disease, diabetes, obesity, malignancy) and those who are immunocompromised