Research Week 3 Part 1.docx
Document Details
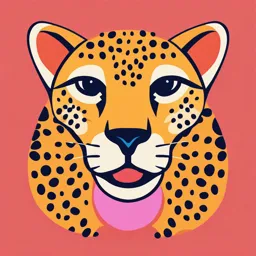
Uploaded by ImprovedNeon
Full Transcript
We finished reading Article 2.4, so let's turn our attention to Unit 2.5, Patients with Amyotrophic Lateral Sclerosis, or ALS. This is the type of patient that you will be working with in the SCI Center. We'll go over some things that the registered respiratory therapist should know about this type...
We finished reading Article 2.4, so let's turn our attention to Unit 2.5, Patients with Amyotrophic Lateral Sclerosis, or ALS. This is the type of patient that you will be working with in the SCI Center. We'll go over some things that the registered respiratory therapist should know about this type of patient subpopulation. We want to highlight the role of CRISPR in ALS research. We also want to delve into how protein misfolding in neural cells can bring about lots of different types of neurodegenerative disorders. We want to explain the role of serial VFTs in treating patients with ALS. In the document that you read, you know now that the existing evidence supports the conclusion that people that are military service members are at a greater risk of developing amyotrophic lateral sclerosis and then dying from the disease than those individuals who have never had any prior military service. That's why the Veterans Administration provides complete support for patients who develop ALS. ALS, otherwise known as amyotrophic lateral disease, is sometimes called Lou Gehrig's disease here in the United States. In Europe, it's called the motor neuron disease. In 2009, the Institute of Medicine found suggestive evidence that there was an association between military service and later development of ALS. This is a progressive muscular paralysis. Sometimes you can see spastic paralysis. Sometimes we can see flaccid paralysis. It's typically due to the fact that you have upper as well as lower motor neuron dysfunction that can lead to not just paralysis, but actual muscle wasting over time. Of course, the thing that concerns respiratory therapists most is the fact that ventilation is impacted in many cases. Ventilation isn't always the primary presentation. However, sometimes it's one of the final things that happen. We'll have an occasion to talk about that when we're on site in the SCI Center. The clinical presentation is rather variable from patient to patient. Most of the patients generally die by about an average of three years, anywhere between one to five years after they have been diagnosed. Almost invariably, this is a result of ventilatory failure. You'll be working in a unit where there will be several of these individuals who are on mechanical ventilation. About 90% of these cases are sporadic. What that means is we can't really trace their dysfunction back to some kind of inheritance pattern. 10% of these individuals are familial, in which case we can go back and we can see that there is some kind of a traceable inheritance. What is the treatment for these individuals? Well, unfortunately, we have no actual efficacious treatments at this time. What we do is we treat the symptoms, we treat the pain, and we treat the stress until the end. We also have a sizable therapeutic intervention for family members who are going through this because the stress on those family members is very, very noticeable and very pronounced. What do we know about ALS as far as its ability to cause this malady? Well, there may be some genomic reasons for ALS. There may be some epigenetic reasons for ALS. There may be some microbiomic reasons. There may be some environmental impacts that set the stage for the development of ALS. We don't know if it's dietary heritage. We don't know if it's an individual who's had a traumatic brain injury in the past. Maybe they've even forgotten all about that traumatic brain injury, but the results are still there. Is it somehow related to smoking? Is it aggravated by smoking? Is this an infectious process that possibly has been subacute? Is it possible that it's due to single nucleotide polymorphisms? And by that, I mean a change in one nucleotide. And on this slide, we're seeing all the different nucleotides that make up the DNA, and those specific colored letters are each a nucleotide. If just one of those changes, is it possible that that can induce ALS? What about something called hexonucleotide repeat expansions, a long-term that means another kind of mutation? Well, the honest answer is we simply don't know at this time. And it could be different pathological pathways for different patients all ending up in what we phenotypically see as ALS. Now, one line of research that is showing promise is research with the proteasome. What I'm showing you here is a proteasome, and the proteasome is made up of a lot of different protein subunits. It's shown here as these squiggly little curlicues that are different colors. And you can see an arrow which brings proteins to this proteasome. The proteasome is an organelle that's found in most cells, including nerve cells, just like you would find a mitochondrion, or you might find Golgi bodies, vesicles, and so forth. So this is a normal organelle that is found in cells. And the job of the proteasome is to take proteins as shown here and to break them down into their constituent amino acids. Why? Well, because sometimes a protein is no longer required in the cell. So by breaking it down to its constituent amino acids, these amino acids can then filter out throughout the entire cell and be repurposed to make brand new proteins that the cell does need. The problem occurs, however, that in some neural cells, for whatever reason, proteins simply don't get reduced to amino acids. They don't get broken down, if you will, into these amino acids. So we have these proteins that are often misfolded, accumulating inside of the neuron. And after a while, there are so many proteins, so densely packed in the neuron, that these misfolded proteins interfere with gene expression. We actually have these proteins backing up into the nucleus of the neuron and causing problems to the DNA. Well, when you do that, you bring about really significant pathologies in the cell. And what we now know is that this misfolding protein problem is at the basis of not just many cases of ALS, but also many cases of Alzheimer's and many cases of Huntington's, all of which are neurodegenerative diseases. So we're beginning to see a common pathway in the cell that may be accountable for some of the kinds of things that we're seeing, typically, in our patients in the clinic. Now, once we have that, we can then begin to think about the possibility of some possibly druggable interaction. Because it appears that there are problems with the DNA, leading to the kinds of changes that we refer to as ALS. Very importantly, recently, is the development of something called the CRISPR-Cas9. CRISPR-Cas9 is a genetic engineering tool. CRISPR stands for Clustered Regularly Interspaced Palindromic Repeats. And as you can see from this cartoon, what it may allow scientists to do, and it's already actually showing great promise, is to go into the DNA where there are either these small changes or even large changes, and to come in with the correct genetic sequence, and to replace the faulty sequences with the correct genetic sequences. Now, while we're waiting for the research to show us the way and to come up with some sort of therapeutic intervention, we still have a large number of patients. And what we also have are protocols, some of which have been produced by the American Academy of Neurology. And these protocols are based on the best available scientific evidence. One thing that we can do is we can provide a neuroprotecting drug called Rilazol. This does slow the disease a little bit. It may prolong survival by a few months for some patients, not necessarily all of them. It is something that appears to be fairly consistent in terms of its ability to work. So we refer to this as a level A recommendation, meaning this is a very strong recommendation. Any patient who has ALS should conceivably receive Rilazol. It is a level A recommendation. And as you'll see, we have other recommendations here that are level B, level C. Level A recommendations are very, very strong and should be implemented. Level B are a little less strong based on literature that's not quite as suggestive. Level C is based on information that is rather circumstantial, still worthy of possibly being tried, but certainly not we're not as confident in level C as we are in level B. We're not as confident in level B as we are in level A. So by definition, we would like all of our recommendations to be level A. What about inserting a PEG tube into an individual to be able to deliver food materials into the gastrointestinal tract of someone with ALS in order to stabilize the weight and prolong their survival? Well, there's level B evidence that that, in fact, should be done. So still fairly strong evidence that that should be done. And you'll see that all of our patients that are on mechanical ventilator have a PEG tube. What about beginning transdisciplinary clinical management to optimize their health care, to prolong survival? Well, we have level B evidence, almost level A evidence that transdisciplinary care is crucial. And I'm going to show you later on in another unit how crucial that really is because we have evidence that we've developed that shows it is actually very close to being level A now. The ability of these groups to also improve health-related quality of care is level C. Once again, that's changing, and it's going in the direction more of a level B recommendation. What about beginning non-invasive ventilation? Well, in order to treat acute and chronic ventilatory insufficiency and prolong survival, we have level B evidence from a number of studies that have been done, not only by pulmonologists, but also by registered respiratory therapists that show that this is, in fact, something important to do. Does it, in fact, help in the decline of the forced vital capacity of the individual? There is some suggestive evidence. We don't have strong evidence at this time, but there is some suggestive evidence. And for that reason, it comes in at a level C. What about providing mechanical inexaflation to help remove secretions? These patients really can't cough. Well, interestingly, there's level C evidence. And the reason for that is because it's not that mechanical inexaflation isn't a good intervention. It's that the studies that have been done have been done with very small numbers of patients. So if you're thinking, "Well, let's collect all of those studies that have been done with small numbers of patients. Let's evaluate them to see how closely related they are. And if they're closely related, then let's do a meta-analysis." I would say you're on the right track. That would be an excellent recommendation. And that is something that we need desperately. So that's a hint to those of you who are perhaps contemplating, among other things, being involved in research in the near future. We just talked about transdisciplinary clinical teams. I will have much more to say about transdisciplinary clinical teams in a couple of minutes. But for now, what I'd like you to realize is this is based on the work of Carol and his team of neurorehabilitators. And what they have determined is that there are special characteristics of the transdisciplinary team shown here at the very top of this pyramid. Years ago, back in the '80s, we used to refer to these teams as multidisciplinary. That's when the doctor was really the primary person on the team, and everyone was sort of in an ancillary position. Then, years later, along came the interdisciplinary team. And sometimes we still refer to these teams as being interdisciplinary. But today, the appropriate terminology is the transdisciplinary teams, for reasons that I will share with you later. These transdisciplinary teams are key because of their ability to impact patient morbidity and patient mortality. In monitoring the lung function of patients with ALS, there are two tools that are used in the home. And I'd like to simply refer to these briefly, because we'll be going over these again later on. One is, of course, the incentive spirometer. The incentive spirometer is helpful because if the patient has an incentive spirometry of, say, 1,000 cc's, then they may have acute ventilatory failure. And we train their caregivers, and we train the family to monitor the incentive spirometer so that if, in fact, there's a drop in incentive spirometry, they can give us a call. We also train them in how to use a peak cough flow meter. If, in fact, the liter per minute flow rate when they do a peak cough flow is less than 207 liters per minute, we want them to go ahead and start with their mechanical inexecuator. If, in fact, that peak cough flow drops down to 160 liters per minute, then we want them to go ahead and start on their noninvasive positive pressure ventilation. And again, we train the family, we train the caregivers, and of course, we train the patient to understand this. Where do these numbers come from? These numbers come from the best available scientific evidence, much of which has been promulgated by John R. Bach, whom we talked a little bit about in previous units. Very important with ALS patients, we want to do serial pulmonary function testing about once every three months or so. We want to test these individuals. We want to do all of the various spirometric values that you see there, the FEV1, horse vital capacity, etc. And very importantly, we want to be able to do two tests each time. We want to be able to do a full test with them seated upright and another full test with them supine. The reason for that is because we know from the scientific literature and also from our clinical experience that when an ALS patient reclines, very often their diaphragmatic function also declines. So we have to test both in sitting and supine position to try to evaluate if there has been a decline in diaphragmatic function. If there is, we need to begin thinking about the possibility of doing noninvasive positive pressure ventilation with these individuals in order to be able to help them when they're in a supine position, particularly when they're going to go to sleep. The evidence does show that by starting noninvasive positive pressure ventilation and possibly even chest optimization protocol, as soon as that horse vital capacity is less than 70%, which is normal, this move slows the decline in horse vital capacity. So there's an ability to impact lung function by being able to test these patients periodically. But if we don't test them periodically, we simply don't know that that functional decline is occurring. That's why it's so important to have them come to the hospital every three months and to be able to test them every three months to see what's happening to them from a pulmonary function standpoint. Well, what happens to those patients that can't come to the hospital that often? What happens to those individuals who are rural veterans? They live in rural areas and they have ALS. Well, now we know again from studies that have been done that these rural veterans are often underdiagnosed, they're undertreated, and therefore they have a significantly greater mortality. So I'm going to leave you with this question. Is there anything that can be done to help this specific subpopulation of patients? All right, this is unit 2.8, using the NM3 device to do clinical research. And we're going to explain the role of the NM3 device in doing real-time monitoring. We're going to list six cardiopulmonary parameters that can be estimated by the NM3. And please know that these are the six that we select because they're based on best available scientific evidence. It's not because these are the only six that we could conceivably select. The NM3 device has literally hundreds of variables that we could have selected from, and we may actually change to other variables in the future. We'll also explain how the NM3 estimates cardiac output and does so noninvasively. Volumetric capnography is arguably better than just N-tidal CO2 monitoring. The reason for that is because N-tidal CO2 monitoring can be coupled with a flow signal. And when you integrate CO2 monitoring with a flow signal, you get volumetric capnography, which then enables you to do all kinds of physiologic monitoring. Therefore, carbon dioxide elimination, which is one of the variables that we can measure with the NM3, is the next generation method for monitoring CO2 beyond N-tidal CO2. This carbon dioxide elimination provides continuous feedback about the condition of the lungs and the condition of the heart. How are they functioning? That's real-time information that the clinician gets right after making a ventilator change. There's no lag time. We can find out if there's been a change in perfusion. We can find out if there's been a change in ventilation. We can even use carbon dioxide data from a blood gas and insert that information into the NM3's computer so that it will monitor for us dead space ventilation in real time. Let's look at some physiologic alterations that affect carbon dioxide elimination. I want to imagine that we have a patient here who is flexing his arm muscle and therefore is producing carbon dioxide. We have a beaker into which all of that carbon dioxide is going. This is basically the carbon dioxide reservoir. It's the amount of carbon dioxide that we now have available as a result of this exercising muscle. We need to obviously get rid of that carbon dioxide from the tissue site and transport it to the lungs. How do we do that? Well, there are three physiologic functions that are involved. Number one is the circulation. The circulation acts to transport the carbon dioxide from the tissue site to the lungs. Once there, a second function, alveolar diffusion, is of tremendous importance. Alveolar diffusion across the alveolar capillary membrane occurs and therefore enables carbon dioxide to be removed from the body. Finally, ventilation. The expansion and the recoil of the pulmonary parenchyma is crucial in being able to evolve carbon dioxide from the body out into the atmosphere. Now that if two specific problems occur, we can tell that they have occurred by using volumetric capnography. If, for example, the circulation has decreased and has decreased abnormally, there will be more carbon dioxide at the tissue site and less carbon dioxide being evolved at the lungs. Also, if ventilation decreases, once again there will be more carbon dioxide left at the tissue site and less carbon dioxide will be evolved from the lungs. So we can infer that there are circulatory changes in a negative direction or ventilatory changes in a negative direction from the ability to measure carbon dioxide elimination from the body. Once again, carbon dioxide being one of the measurements that can be done by volumetric capnography. you you The transitional airways in the tracheobronchial tree have some alveolar air spaces but not very many. So a small amount of carbon dioxide will be emanating from those alveolar air spaces and for that reason during phase two of the volumetric capnogram tracing we will see an uptick in carbon dioxide. Finally during phase three of the volumetric capnogram we will see carbon dioxide from the alveolar air spaces. There are many many alveolar air spaces as you know and so as gas is evolved from the alveolar air spaces there will be a higher and higher level of carbon dioxide being read over the course of the patient's breath. This slide shows what we have referred to in the past as being the important feature of volumetric capnography and that is there is breath by breath integration of N-tidal CO2 and also flow. Let me demonstrate this for you. Here are three waveforms. Now if we were only monitoring N-tidal CO2 you can see that the N-tidal CO2 on each of these waveforms is 32 millimeters of mercury. For the first it's 32, for the second it's 32, for the third it's 32. 32 for each of those and you know that because if you look along the y-axis you can see that the top part of the curve is right at around 32 millimeters of mercury. So if that's all we were monitoring that's all we would know. However, if we were to look at volumetric capnography we don't just monitor N-tidal CO2 we also monitor the flow. And in this case what we have is a tidal volume measurement. So if you look at the tidal volume measurement for the very first waveform you can see that it's 600 cc or 600 milliliters, the next one is 800 and the final one is 1000. And what you'll notice is that for the very first waveform if we take N-tidal CO2 and we integrate that with the tidal volume what we get is the carbon dioxide elimination and the carbon dioxide elimination in this case is 50 mls per minute. So we know how much carbon dioxide we're actually removing from the body. In the next waveform it's 200 mls per minute and in the third waveform it's 300 mls per minute. So in spite of the fact that the N-tidal CO2 is not changing since we're integrating flow with the N-tidal CO2 we can definitely discern whether or not we are removing carbon dioxide from the body and how much carbon dioxide precisely we are removing from the body. And that's key information if we're going to be able to calculate the physiologic variables that we've referred to. Now here's just an overall shot of the circuit as we will put it together at the bedside. If you look at the machine on the upper left that is a Trilogy mechanical ventilator. That is the kind of ventilator that they use. It's up on the wall. It's used for the ALS patients. It can also be placed in the back of the wheelchair so that the ALS patient can be taken around on his or her mechanical ventilator. To the right of the ventilator is the NM3 device and you can see a number of tubes. You can see cables and we're going to have occasion to define each of these. I just wanted to give you an overview here at this juncture so that you could see the basics. And you can see that gas comes from the Trilogy mechanical ventilator, proceeds through the NM3's circuit, goes past the heat and moisture exchanger, and finally through a small flex tube into a lung. The analog lung that you see here in teal. So what we want to do is we want to just kind of keep that in mind because this is the setup as we will use it at the bedside. Again, we're not going to put the NM3 on top of the mechanical ventilator. You can see that both are about the same size and because the Trilogy is on the wall we don't want to put the NM3 on top of the ventilator. Here's a close-up of the NM3 circuit and toward the top right is the coupling joint for the drape tube. You can see where it says patient connection and just behind that you can see where it says the capnostat sensor. This is a sensor that senses carbon dioxide. Right behind that is a CO2 flow sensor. That's the sensor that senses flow. It is a differential pneumatic. And because both of these sensors are together we're going to measure not just carbon dioxide but also the flow. And we said that when you put those together you're going to get the waveforms that we just saw in the last slide. And we're going to be able to measure a number of physiologic variables. Behind that is the rebreathing valve which is controlled by the computer in the NM3 device. This rebreathing valve will redirect gas from the patient into that loop of the corrugated circuit and will do so for about 15 seconds during which the computer will then compute the cardiac output for the patient. More on that later. When we integrate carbon dioxide and flow using the capnostat and the differential pneumatic that I showed you in the last slide, this will provide estimates of a wide range of cardiopulmonary variables. Among these are carbon dioxide elimination, static chest compliance, alveolar minute volume, physiologic dead space, cardiac output, error resistance, and many others. On the capnography side, as you can see, we can monitor end-tidal CO2, we can generate a capnogram, and we can monitor respiratory rate. And that's really about it.