Regulation Of Breathing Notes (PDF)
Document Details
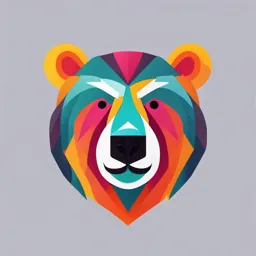
Uploaded by FruitfulIntegral
WSUSOM
Tags
Summary
These notes cover respiratory physiology, focusing on the regulation of breathing. They detail chemoreceptors, stretch receptors, and irritant receptors, as well as the inputs to the respiratory control system. The document is intended for an undergraduate-level course.
Full Transcript
WSUSOM Medical Physiology – Respiratory Physiology Page | 1 of 13 Regulation of Breathing REGULATION OF BREATHING Learning Objectives 1. List the anatomical locations of chemoreceptors sensitive to changes in arterial PO2, PCO...
WSUSOM Medical Physiology – Respiratory Physiology Page | 1 of 13 Regulation of Breathing REGULATION OF BREATHING Learning Objectives 1. List the anatomical locations of chemoreceptors sensitive to changes in arterial PO2, PCO2, and pH that participate in the control of ventilation. Identify the relative importance of each in sensing alterations in blood gases. 2. Name five causes of hypoxemia. 3. Describe how changes in arterial PO2 and PCO2 alter alveolar ventilation, including the synergistic effects when PO2 and PCO2 both change. 4. Describe the respiratory drive in a COPD patient and predict the change in respiratory drive when oxygen is given to a COPD patient. 5. Give three examples of reflexes involving pulmonary receptors that influence breathing frequency and tidal volume. Describe the receptors and neural pathways involved. 6. Identify the mechanisms by which particles are cleared from the airways. Lecture Outline I. Chemoreceptors A. Peripheral chemoreceptors Location of receptors Stimuli that activate the peripheral chemoreceptors (e.g. hypoxia, hypercapnia, pH) Role of the peripheral chemoreceptors in modulating breathing B. Central chemoreceptors Location of receptors Stimuli that activate the central chemoreceptors (e.g. hydrogen ions and carbon dioxide) Role of the central chemoreceptors in modulating breathing II. Stretch Receptors Location of receptors Stimuli that activate receptor Impact of activating receptors on breathing pattern and discomfort III. Irritant Receptors Location of receptors throughout the tracheobronchial tree Impact of activating receptors on bronchoconstriction and breathing The role of clearance mechanisms in removing irritants from the tracheobronchial tree (e.g. cilia and macrophages). WSUSOM Medical Physiology – Respiratory Physiology Page | 2 of 13 Regulation of Breathing Inputs to the Respiratory Control System Inputs to the Respiratory Control System - Descending Inputs Several regions in the central nervous system above the level of the brainstem are known to influence breathing. The most important of these are the hypothalamus and the cerebral cortex. The hypothalamus can influence breathing in at least three ways. Changes in body temperature often lead to changes in the rate of breathing. These changes are elicited via the hypothalamic thermoregulatory center. Powerful emotions, such as fear, anxiety, rage etc. can profoundly affect the respiratory rhythm. These influences arise largely in the hypothalamus. Releasing hormones synthesized within the hypothalamus influence breathing. These include thyrotropin releasing hormone, which ultimately influences T3 and T4 levels, and gonadotropin releasing hormone, which ultimately influences progesterone levels. Both thyroid hormones and progesterone stimulate breathing. Although the control of breathing is often involuntary it is possible to exert conscious control over breathing. We can hold our breath or deliberately hyperventilate. In addition, the cerebral cortex has other more subtle influences on the pattern of breathing during talking, singing and playing musical instruments. Many descending cortical influences bypass the brainstem respiratory centers and synapse directly on respiratory motoneurons. These voluntary pathways, originating in the pyramidal tracts, pass down the lateral spinal columns in the corticospinal tracts. Involuntary pathways from the brainstem respiratory centers, on the other hand, travel in the ventral and ventrolateral columns as the bulbospinal tracts. This separation of voluntary and involuntary control can sometimes be seen when the central nervous system is damaged, for example following a stroke. Involuntary control may be preserved but cannot be overruled consciously. In other instances, involuntary control may be lost, leaving only voluntary control, as seen in the rare condition known as congenital central hypoventilation syndrome (formerly known as Ondine’s Curse). In addition to the hypothalamic and cortical influences, the breathing pattern is also influenced via the “locomotory pattern generator” that relays through the thalamus. In this way, breathing can be entrained to various locomotory rhythms (e.g. running and swimming). WSUSOM Medical Physiology – Respiratory Physiology Page | 3 of 13 Regulation of Breathing Peripheral Chemoreceptors The peripheral chemoreceptors are located in the bifurcation of the common carotid arteries. They receive a very rich blood supply from a small artery arising directly from the external carotid artery. It is claimed that the carotid bodies have a higher blood flow per unit weight of tissue than any other structure in the body. The carotid bodies are innervated by the carotid sinus nerve, which is a branch of the glossopharyngeal nerve (cranial nerve IX). Each carotid body consists of two cell types. Other more diffuse groups of cells are found in the wall of the aortic arch and are referred to as aortic bodies. Branches of the vagus nerve (i.e. cranial nerve X) innervate the aortic body chemoreceptors. The aortic bodies do not have a significant role in the control of breathing in humans. The carotid body chemoreceptors display a low level of activity at normal levels of oxygen. This activity can be silenced by breathing increased levels of oxygen. If the blood perfusing the carotid bodies falls, carotid body activity rises abruptly once oxygen values decline to a partial pressure of 60-70 mmHg (see graph on next page). It is important to understand that glomus cells in the carotid bodies respond to the partial pressure of oxygen and not oxygen content. Thus, the carotid bodies respond to hypoxemia (e.g. high altitude or pulmonary disease) but not to anemia or carbon monoxide poisoning. WSUSOM Medical Physiology – Respiratory Physiology Page | 4 of 13 Regulation of Breathing The exact way the receptors detect changes in the partial pressure of oxygen is not completely understood. However, it appears to involve an increase in calcium ions in the glomus cells. It is also not completely understood how the glomus cell signals the carotid sinus nerve. Several neurotransmitters and neuromodulators have been implicated. For example, dopamine levels in type I cells are altered in response to changes in the partial pressure of oxygen. Additionally, acetylcholine (acting through both nicotinic and muscarinic receptors) stimulates an increase in activity in the carotid sinus nerve. Several neuropeptides, particularly adenosine and substance P also increase the activity of the carotid sinus nerve. The carotid bodies also respond to increases in the partial pressure of carbon dioxide. Carbon dioxide is detected by way of hydrogen ions generated within the glomus cells when carbon dioxide comes in contact with carbonic anhydrase located in the cells. Although the carotid bodies provide only 15 – 25 % of the hypercapnic ventilatory response, they allow for a much faster response than the central chemoreceptors. The carotid bodies also respond to hydrogen ions derived from other sources (i.e. lactic acid, ketone bodies) and potassium. There has been speculation over recent years that cells in other parts of the periphery might detect changes in local levels of oxygen, carbon dioxide or hydrogen ions. The discovery of carbon dioxide sensitive receptors in the lungs of reptiles and birds led to studies that tried to confirm the presence of similar cells in mammals. However, it appears that these lung receptors do not exist, apart from the response of J receptors to hydrogen ions. Blood vessels respond to carbon dioxide, oxygen and hydrogen ions, but this is due to the direct action of these variables on smooth muscle in the walls of arterioles and pre-capillary sphincters. Results from studies that have been designed to determine whether receptors that respond to oxygen, carbon dioxide or hydrogen ions exist in skeletal muscle have not yielded unequivocal results. WSUSOM Medical Physiology – Respiratory Physiology Page | 5 of 13 Regulation of Breathing Central Chemoreceptors The central chemoreceptors are located on the ventral surface of the medulla, where they form diffuse groupings of cells. The immediate environment of the central chemoreceptors is the brain extracellular fluid (ECF). Brain ECF is influenced by the metabolism of the brain, the rate of flow and composition of the blood perfusing the brainstem, and the composition of the cerebrospinal fluid (CSF). Because of the special non-fenestrated nature of the capillaries of the cerebral circulation, the chemoreceptors are separated from the blood by the blood-brain barrier. The blood brain barrier allows carbon dioxide to diffuse across it easily, but it excludes most ions including hydrogen and bicarbonate ions. The properties of the cerebral spinal fluid are also important in understanding the operations of the central chemoreceptors. The cerebral spinal fluid lacks protein, so it only has about half the buffering power of the blood. Hence the hydration of carbon dioxide within the cerebral spinal fluid produces excess unbuffered hydrogen ions. The pH of the cerebral spinal fluid is normally 7.3 (hydrogen ion concentration 50 nM/L), compared with the 7.4 of arterial blood (hydrogen ion concentration 40 nM/L). Following a step rise in the partial pressure of carbon dioxide there is an exponential “wash-in” of carbon dioxide across the blood brain barrier, which begins to the affect the central chemoreceptors within about 90 seconds, reaching a steady state by about 5 minutes. The carbon dioxide influences the central chemoreceptors directly by rapid diffusion through the brain tissue. Due to the buffering of carbon dioxide within the brain extracellular fluid, this direct effect of carbon dioxide may be transitory unless supplemented by changes in the cerebral spinal fluid, which is in equilibrium with the blood in regard to carbon dioxide. Hydrogen ions diffuse out of the extracellular fluid towards the chemoreceptor cells, and thus provide a second, longer lasting, stimulus. Whichever route carbon dioxide takes to stimulate the central chemoreceptors, the receptors are responding to the blood carbon dioxide. There appears to be a linear relationship between the partial pressure of carbon dioxide and the central chemoreceptor response over the normal physiological range of partial pressure of carbon dioxide in arterial blood. WSUSOM Medical Physiology – Respiratory Physiology Page | 6 of 13 Regulation of Breathing Combining the Efferent and Afferent Arms of the Respiratory Control Loop The efferent and afferent arms of the respiratory control loop are depicted on this graph above. The line labeled resting metabolic hyperbola represents the efferent arm of the respiratory control loop. The resting metabolic hyperbola represents the impact that ventilation has on carbon dioxide. Thus, as ventilation decreases carbon dioxide increases. Conversely, when ventilation increases carbon dioxide decreases. The remaining lines on the graph represent the impact of the peripheral and/or the central chemoreceptors on ventilation (i.e. the afferent arm of the respiratory control loop). For example, the line that is labeled > 150 mmHg symbolizes the impact that the central chemoreflex alone has on ventilation; since inspiring an elevated level of oxygen abolishes the input from the peripheral chemoreceptors. Note that once a threshold is achieved (i.e. approximately 39 mmHg) as carbon dioxide increases ventilation increases in a linear fashion. Note that the rate of raise in ventilation (i.e. slope) in response to carbon dioxide increases as the arterial partial pressure of oxygen (PO2) decreases (100 vs. 60 vs. 40 mmHg). Thus, the degree to which the peripheral chemoreflex is stimulated is dependent on the level of arterial PO2. Note that the line labeled 100 mmHg represents the normal level of oxygen in arterial blood. Moreover, note that the point at which the metabolic hyperbola intersects with the line (100 mmHg) represents the normal resting equilibrium point for ventilation and the partial pressure of arterial carbon dioxide (i.e. the point at which the efferent arm of the control loop is in equilibrium with the afferent arm of the control loop). Thus, the normal resting value for ventilation is approximately 10 L/min and the resting PCO2 is approximately 40 mmHg in this example. Additionally, note that below the threshold as carbon dioxide increases ventilation does not respond. However, even though the central chemoreceptors do not affect ventilation below a given threshold, ventilation does not drop to zero below the threshold. Accordingly, other stimuli must be responsible for maintaining ventilation even though the influence of the central chemoreflex is absent. More specifically, behavioral and arousal influences (i.e. wake vs. sleep, light vs. dark, quiet vs. noise) will maintain ventilation if the level of carbon dioxide is below the threshold. WSUSOM Medical Physiology – Respiratory Physiology Page | 7 of 13 Regulation of Breathing Stable Breathing during Sleep The last statement on the previous page is only true for wakefulness. In general, under conditions of sleep, arousal and behavioral stimuli do not exist. Thus, ventilation is primarily controlled by the peripheral and central chemoreceptors during non-rapid eye movement sleep. If carbon dioxide is above the chemoreflex threshold ventilation will be stable during non-rapid eye movement sleep. A variety of measures are obtained during sleep to determine if individuals are experiencing stable breathing. These measures include snoring intensity, movements of the abdomen and rib cage, oro-nasal airflow and measures of oxygen saturation. The diagram above shows the typical waveforms observed in a healthy individual with stable breathing during non-rapid eye movement sleep. Notice that oro-nasal airflow and movement of the rib cage and abdomen indicate that breathing is stable in this individual. Consequently, oxygen saturation levels are steady and maintained at a normal value (in this case 95 %). Likewise, the individual is in stable uninterrupted N2 sleep. On the other hand, ventilation will be unstable if the level of carbon dioxide is below the chemoreflex threshold as described on the following pages. Consequently, apnea occurs, oxygen levels decrease, and carbon dioxide levels increase in the blood. WSUSOM Medical Physiology – Respiratory Physiology Page | 8 of 13 Regulation of Breathing The reason ventilation becomes unstable during sleep can be explained using the graph shown above. If ventilation is high and does not match metabolic output carbon dioxide will be reduced. If the increase in ventilation is great enough it will result in carbon dioxide being reduced below the apneic threshold (see figure above). If this occurs, ventilation is not maintained during sleep (it is maintained during wakefulness because of the wakefulness drive to breath) and as a result apnea ensues. Apnea ensues because the induction of hypocapnia results in the disfacilitation of respiratory neurons resulting in a central apnea. Likewise, disfacilitation of hypoglossal motoneurons (the hypoglossal nerve innervates the genioglossus muscle which contributes to maintaining airway patency) and consequently reductions in genioglossus muscle activity may result in the development of an obstructive apnea. Given this scenario it is easy to understand how the appearance of an apnea might lead to a subsequent cluster of apneic events. If an individual experiences an apnea, the consequent hypoxia and hypercapnia leads to an arousal. During the subsequent arousal the increased ventilatory response (i.e. hyperventilation) to hypoxia and hypercapnia may lead to a reduction in carbon dioxide below the threshold. This event in conjunction with the transition to a sleep state would result in a subsequent apneic event. WSUSOM Medical Physiology – Respiratory Physiology Page | 9 of 13 Regulation of Breathing Impact of carbon dioxide set-point and sensitivity on breathing stability The above figure demonstrates the initiation of a central apneic event followed by a reduction in airway diameter (i.e. obstructive apnea event) in response to decreased levels of carbon dioxide. In this experiment, subjects were artificially hyperventilated during sleep. The period of hyperventilation is indicated by the measures of end-tidal carbon dioxide on the left side of the upper figure. In addition to artificially ventilating the participant, images of the upper airway were obtained to monitor the diameter of the upper airway (colored in blue). Note that during ventilation the upper airway was patent (panel 1 – lower figure). Once carbon dioxide levels were sufficiently reduced the ventilator was turned off (panels 2 – 4). In response to turning the ventilator off the participant did not breath spontaneously (i.e. central apnea) (panels 2 – 4), because carbon dioxide was below the apneic threshold (see explanation on the previous page). In addition, note that the diameter of the upper airway gradually decreased from panel 2 – 4. Disfacilitation of hypoglossal motoneurons due to the reduction in carbon dioxide (the hypoglossal nerve innervates the genioglossus muscle which contributes to maintaining airway patency) led to reductions in genioglossus muscle (and other upper airway muscles) activity resulting in the development of an obstructive apnea. WSUSOM Medical Physiology – Respiratory Physiology Page | 10 of 13 Regulation of Breathing Complex Apnea WSUSOM Medical Physiology – Respiratory Physiology Page | 11 of 13 Regulation of Breathing Summary of Events that Lead to Unstable Breathing If an individual experiences an apnea, the consequent hypoxia and hypercapnia leads to an increased ventilatory effort and often an arousal from sleep. During the subsequent arousal, pharyngeal muscle activity is restored, the airway opens and ventilation increases to restore blood gas values back to homeostatic levels. However, the increased ventilatory response (i.e. hyperventilation) to hypoxia and hypercapnia may lead to a reduction in carbon dioxide below the apneic threshold. This event in conjunction with the transition to a sleep state would result in a subsequent apneic event as outlined on the previous two pages. These relationships might result in the perpetuation of apneic events throughout the sleep period. Examples from individuals with complex apnea (central and obstructive events combined) and heart failure with central apnea shown on the previous page. Note that the participants experienced a series of apneic events. Each apnea was induced by hyperventilation that occurred in response to a preceding event. WSUSOM Medical Physiology – Respiratory Physiology Page | 12 of 13 Regulation of Breathing Regulation of Breathing - Other Neural Inputs Lung and airway receptors The conducting airways and the lungs themselves possess receptors that monitor the state of the respiratory system and alert the respiratory center to changes in a number of variables. Many different receptors, with a wide range of properties, exist. The pulmonary stretch receptors are found throughout the lung connective tissue. They respond to stretching brought about by rapid and sustained inflation of the lung. Vagal afferents originating form pulmonary stretch receptors inhibit the firing of neurons in the DRG, thereby halting inspiration and initiating expiration. In this way, the stretch receptors contribute to phase switching. This reflex is known as the Hering-Breuer reflex. Note that when the vagus nerve is cut inspiration will be prolonged (top and middle tracing in right hand figure). The Hering-Breuer reflex appears to operate on a “breath-by-breath” basis in a number of species, but it only operates in adult humans when tidal volume becomes at least three times the normal value (e.g. during extreme exercise or when challenged by chemical stimuli). However, in babies the reflex is important in stabilizing the breathing pattern during at least their first year of life. These receptors are found in the mucosa lining of the larynx and trachea. They respond to both mechanical and chemical stimuli. These receptors initiate the cough reflex, which results in a series of forced expirations, which function to clear the airway. WSUSOM Medical Physiology – Respiratory Physiology Page | 13 of 13 Regulation of Breathing Irritant Receptors Irritant receptors are found throughout the airway from the nose to the alveoli and as their name imply respond mainly to irritating stimuli. Their stimulation initiates various protective reflexes, which have stereotyped patterns depending on the location of the irritant receptors stimulated. A typical classification is listed on the next page. Receptor Reflex Nerve · nasal sneeze trigeminal nerve · epipharyngeal aspiration glossopharyngeal · laryngeal cough vagus · tracheal cough vagus Irritant receptors which respond to noxious gases, cigarette smoke, inhaled dust and cold air are also scattered throughout the airway from the trachea to the bronchioles as shown in the table above. Special juxtapulmonary capillary receptors (J-receptors) are located in the alveolar wall close to the capillaries. The J-receptors respond to engorgement of pulmonary capillaries and increases in the interstitial fluid volume of the alveolar. Information from the J-receptors feed back to the medulla via the vagus and cause rapid shallow breathing or apnea in response to intense stimuli. These receptors have a role on the breathlessness experienced by those with heart failure and interstitial lung disease. The irritant receptors all share the ability to stimulate breathing and produce bronchoconstriction. The bronchoconstriction is produced when bronchiolar smooth muscle is activated from the nucleus ambiguus via the vagus nerve. Cough, for example, consists of a deep breath and explosive expiration with the bronchoconstriction increasing the velocity of airflow and therefore acting to expel foreign particles from the airway. However, o these reflexes can work against us. In asthma, bronchoconstriction is produced by the release of active agents, but these agents also stimulate irritant receptors and thereby exacerbate the bronchoconstriction via the reflex. There are a variety of irritants in the atmosphere including nitrogen oxides that cause inflammation of the V upper airway tract and eye irritation, sulfur oxides that cause pulmonary edema and bronchial inflammation, and carbon monoxide and hydrocarbons that exist in tobacco smoke. The size of the pollutant particle determines its fate. Large particles are trapped in the mucosa of the nose and pharynx. Medium-size particles typically deposit in the small airway and the smallest of particles reach the alveoli where some are deposited via diffusion through the wall. Many small particles are exhaled during subsequent breaths. There are a variety of clearance mechanisms to remove the particles, including being swept up by mucus propelled by cilia (medium size particles) and swallowed or expectorated. Particles deposited in the alveoli are engulfed by macrophages that leave via the blood or lymphatic system.