Reading Notes - Carlson & Birkett Chapter 2 PDF
Document Details
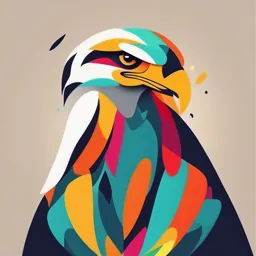
Uploaded by ReputableHorse
Tags
Summary
This document contains reading notes on chapter 2 of Carlson & Birkett's work, focusing on the central and peripheral nervous systems, neuron structures, supporting cells, and neural communication mechanisms. It explains concepts like membrane potential, resting potential, and action potentials.
Full Transcript
Reading Notes Carlson & Birkett Chapter 2 1. What are the features of the central and peripheral nervous systems, respectively? a. Central nervous system: brain and spinal cord i. Communicates with rest of body through nerves attached to brain and spinal...
Reading Notes Carlson & Birkett Chapter 2 1. What are the features of the central and peripheral nervous systems, respectively? a. Central nervous system: brain and spinal cord i. Communicates with rest of body through nerves attached to brain and spinal cord -> nerve fibres transmit messages through nerve from sense organ to the brain or from brain to muscle or gland ii. Between sensory and motor neurons -> interneurons, lie entirely within CNS 1. Local interneurons: form circuits with nearby neurons and analyse small pieces of information 2. Relay interneurons: connect circuits of local interneurons in one region of brain with those in other regions b. Peripheral nervous system: nerves and most sensory organs i. Sensory neurons: gathers information in form of light, sound, odors, taste, etc from environment ii. Motor neurons: control motor behaviour required for contracting muscles 2. Explain and distinguish the structures of a neuron. a. Basic information-processing and information- transmitting unit of nervous system (consist of cell body or soma, dendrites, axon, and terminal buttons) i. Soma: cell body; contains nucleus and much machinery involved in life processes of cell ii. Dendrites: receive messages from neuron communication, receive those across synapse -> happens in one direction: from terminal button (on presynaptic/sending cell) to membrane of other cell (postsynaptic cell/receiving cell) iii. Axon: long thin tube; outer surface of axon carries information from cell body to terminal buttons -> like electrical cord carrying electrical message from outlet to appliance 1. Carries action potential (= brief electrical and chemical event that starts at end of axon near cell body at point called axon hillock and travels to ends of terminal buttons) 2. In any axon, AP is always exactly same size and duration 3. Axons can be extremely long -> needs system that can transport items rapidly and efficiently inside axon => axoplasmic transport = moves substances along “tracks” that run inside the length of the axon (APs on surface of axon only) -> this is accomplished by molecules of a protein called kinesin a. Kinesin: resemble pair of legs and feet, attached to item being transported down the axon, walks down a microtubule, carrying cargo to its destination (powered by ATP molecules created by mitochondria) b. Dynein: carries substances from terminal buttons to soma => retrograde axoplasmic transport; about half as fast as anterograde transport iv. Myelin sheath: fatty substances providing insulation for electrical message carried along axon membrane 1. Bundle of myelinated axons appear as white matter in brain and nerves => tracts v. Terminal buttons: knobs at the end of axon branches 1. When AP travelling down reaches terminal buttons, they secrete neurotransmitters, either excitatory or inhibitory 2. Axon sends message to terminal buttons -> chemical message released to diffuse into synapse -> relay message from pre to postsynaptic cell vi. Other cell structures 1. Membrane: boundary of neuron, contains double layer of lipid molecules 2. Cytoskeleton: shape of neuron, made of three kinds or protein strands – thickest of these strands are microtubules 3. Microtubules: bundles of 13 protein filaments arranged around hollow core, form “tracks” for axoplasmic transport 4. Cytoplasm: semiliquid, jelly-like substance that fills the space surrounded by the membrane, including the soma 5. Nucleus: enclosed by nuclear membrane, contains chromosomes 6. Chromosomes: consist of long strands of DNA -> contain recipes for individual proteins, genes responsible for initiating process of protein synthesis in cell 7. MRNA: copies information stored by gene, leaves the nucleus with copied information and attaches to ribosomes in the soma, ribosomes use copied information from rMNA to synthesise proteins for cell 8. Enzymes: direct chemical processes of cell by controlling chemical reactions 9. Mitochondria: found in cytoplasm; use nutrients such as glucose to provide cell with energy to perform its functions a. Produces ATP b. Vital role in economy of cell 10. ATP: adenosine triphosphate, created by mitochondria, used as energy source throughout cell 3. What are the supporting cells in the central and peripheral nervous systems? Compare them. a. CNS: b. Glia (glial cells): most important supporting cells of CNS i. Surround neurons and hold them in place ii. Regulate their supply of nutrients and chemicals needed to exchange messages with other neurons iii. Insulate neurons from one another iv. Destroy and remove pathogens or dead neurons v. Involved in growth, repair, and development of nervous system vi. Can also be involved in synaptic communication c. Astrocyte: star cell, provide physical support to neurons and clean up debris within the brain i. Produce some of the chemicals that neurons need to do functions ii. Help control chemical composition of fluid surrounding neurons by taking up or releasing substances that need to be kept within specific levels iii. Surround somatic and dendritic membranes iv. Function as neuron glue v. Surround and isolate synapses, limiting dispersion of NTs released by TBs 1. Phagocytosis: astrocytes contact piece of debris from dead neuron, then engulf and digest it d. Oligodendrocytes: provide support to axons and produce myelin sheath, insulates axons from one another i. Produces myelin; 80% lipid and 20% protein in tube surrounding the axon -> tube consists of series of segments, each ca 1 mm long, with small portion of uncoated axon called node of Ranvier ii. Produces up to 50 segments of myelin e. Microglia: smallest glial cells i. Act as phagocytes ii. Serve as part of immune system in brain by protecting brain from invading microorganisms iii. Responsible for inflammatory reaction in response to brain damage f. PNS: g. Schwann cells: also support axons and produce myelin, but: i. In PNS, a Schwann cell provides myelin for only one axon and the entire cell (not just part of it) surrounds axon ii. Schwann cells in the PNS differ from oligodendrocytes in the CNS by aiding in nerve repair. After damage, Schwann cells digest the dead axons and form guiding cylinders for axon regrowth. Axon sprouts grow in all directions, but those that encounter a Schwann cell cylinder grow quickly, potentially reconnecting with their original targets if the nerve ends are close enough. 4. What is the function of the blood brain-barrier? a. Barrier between blood and fluid surrounding cells of the brain i. Some substances can cross BBB and enter CNS, but others can’t -> semi-permeable ii. In brain, capillaries are tightly packed together - > no gaps, so it’s very hard for substances to pass through, however, some substances can be transported through the capillary walls by special proteins 1. Example: glucose transporters bring brain its fuel and other transporters rid brain of toxic waste products iii. Function: transmitting messages from place to place in brain depends on delicate balance between substances within neurons and those in extracellular fluid surroundin gthem iv. Presence of BBB makes it easy to regulate the composition of extracellular fluid v. In some places, BBB is more permeable, like in area postrema (vomiting control centre) 1. BBB is weaker there, permitting neurons in region to detect presence of toxic substances in blood, prevents substances from diffusing from this region in brain to the rest of brain 2. Poison entering circulatory system from stomach can stimulate area postrema to initiate vomiting -> expels poison 5. What is the process of neural communication in a reflex? a. Flinching away from object: Neural communication in reflex begins when sensory neurons detect painful stimulus, such as heat, and send signals down axons to terminal buttons in spinal cord -> TBs release excitatory NTs, stimulating interneurons -> INs activate motor neurons, which send signals to muscles, causing them to contract and move body part away from stimulus b. When having to hold onto a cup: Inhibitory signals from brain counteract the reflex; axons from neurons activate inhibitory interneurons in spinal cord, releasing NTs that reduce motor neuron activity -> prevent reflexive action 6. Describe membrane potential, resting potential, hyperpolarization, depolarization, and the action potential. a. Membrane potential: Most neurons are approx. 70 units or –70mV more negatively charged inside axon compared to outside, any difference in charge (positive or negative) across membrane is called membrane potential i. Potential => stored-up source of energy ii. Neuron is like a battery; has a difference in charge between inside of cell (neg) and outside (pos) -> difference in charge is called membrane potential b. Resting potential: neuron at rest and not involved in communicating with any other neurons, the membrane potential remains at approximately –70mV c. Hyperpolarisation: inside of axon becomes more negative (from resting potential) relative to outside (less likely to send electrical message) d. Depolarisation: inside of axon becomes from positive relative to outside (more likely to send electrical message) e. Action potential: burst of rapid depolarisation followed by hyperpolarisation -> begins at point where soma meets axon (axon hillock) and travels all the way to end of TBs -> triggers TBs to release NTs into synapse 7. Summarize how diffusion, electrostatic pressure, and the sodium–potassium pump help establish membrane potential. a. Diffusion – the “crowded room” rule: if people are crammed into one room (high concentration) they will naturally spread out into nearby empty room (low concentration) to balance space i. In neurons: ions (Na+ and K+) move from areas of high concentration to low concentration -> Na+ wants to move into cell because there’s less of it inside b. Electrostatic pressure – the “magnet” rule: opposites attract (+ and – sticke together), but like charges repel (+ and +n push apart) i. In neurons: positive ions (cations, like Na+ and K+) are repelled by other positive regions and attracted to negative regions -> negative ions are pushed away from other regions c. Inside neuron – intracellular fluid: i. Potassium is the main positive ion ii. Organic anions (A-) are big, negative molecules that stay inside cell d. Outside the neuron – extracellular fluid: i. Sodium (Na+) and chloride (Cl-) are found mostly outside ii. Outside of neuron is like seawater (NaCl = salt water) e. Sodium-potassium pump – the “bouncer at the club”: i. Imagine cell membrane is a club, and sodium keeps trying to sneak inside ii. To maintain balance, there’s a bouncer called sodium-potassium pump: 1. Kicks 3 sodium ions (Na+) for every 2 potassium (K+) it lets in 2. This uses energy, like a bouncer that works hard to maintain order iii. Why? 1. Keeps Na+ outside and K+ inside neuron, maintaining battery-like charge iv. Key points: 1. Diffusion makes ions want to spread evenly (Na+ wants in, K+ wants out) 2. Electrostatic pressure keeps charges balanced (+ attacted to -) 3. Sodium-potassium pump constantly works to rest balance by pushing Na+ out and pulling K+ in 4. Balance keeps neuron ready to fire an AP (like charging up a battery) 8. Summarize the series of ion movements during the action potential. a. Resting state to threshold trigger i. At rest inside of neuron is negative (-70 mV) ii. When neuron gets excited enough (reaches threshold), sodium channels open b. Sodium rushes in -> depolarisation i. Sodium ions (Na+) rush into cell because of diffusion (spreading out) and electrostatic pressure (attracted to negative inside) ii. Makes inside of neuron rapidly positive (+40mV) c. Potassium channels open -> K+ rushes out i. After slight delay, potassium channels open, and K+ ions leave cell ii. Since inside is now positive, potassium (K+) is pushed out to rebalance things d. Sodium channels close -> refractory period i. Sodium channels close (become refractory, can’t open again until neuron resets) ii. K+ keeps leaving, makes inside start to become negative again) e. Return to resting state -> repolarisation i. Potassium channels begin to close as neuron’s charge returns to resting potential (-70 mV) ii. Neuron briefly overshoots and becomes a little too negative (= hyperpolarisation) before stabilising f. Cleanup by the sodium-potassium pump i. SPP works to push Na+ back out and bring K+ back in to reset neuron for next AP g. Big picture: i. NA+ rushes in -> neuron becomes more + ii. K+ rushes out -> neuron becomes – again iii. SPP then resets everything, like cleaning up after a party 9. Describe conduction of the action potential. a. How the electric signal gets transmitted b. Once action potential starts, it travels down axon without changing in size or strength, which is due to: i. All-or-none law: AP either fires completely or not at all, no in-between c. If APs always the same size, how do neurons communicate different strengths (like dim vs bright light)? -> rate law explains: i. Strong stimulus (like bright light) -> neurons fire more rapidly ii. Weak stimulus (dim light) -> neurons fire less frequently d. Myelinated vs unmyelinated axons -> myelinated axons conduct signals much faster e. Saltatory conduction (“jumping” signal) i. In myelinated axons: 1. Myelin sheath insulates axons, leaving only small gaps called nodes of Ranvier 2. At nodes, Na+ flow in, generating APs 3. Between nodes, electrical signal electrical travels passively and quickly like signal through cable 4. Signal “jumps” from one node to next 5. Benefits: energy efficient (sodium only enters at nodes of Ranvier so less energy is needed to pump it out) and faster signal transmission (myelinated axons send signals much faster than unmyelinated) 10. What are the presynaptic structures involved in synaptic communication? a. Presynaptic membrane: located at end of axon’s terminal button (sending message) i. Mitochondria: provide energy for TB to function, like releasing NTs ii. Synaptic vesicles: tiny membrane sacs filled with NTs iii. Two types: 1. Small synaptic vesicles: contain NTs, found in large numbers near release zone a. Made in soma and transported to TB b. Some recycled at TB after NTs are released 2. Large, dense-core vesicles: contain special molecules called peptides a. Only produced in the soma and then transported to TB iv. Microtubules: act like train tracks, transporting materials (like vesicles) from soma (cell body) to TB b. Postsynaptic membrane: on receiving neuron c. Synaptic cleft: between pre- and postsynaptic membrane; fluied-filled space where NTs travel d. Summary: synaptic vesicles hold NTs -> when AP reaches TB, vesicles move to releae zone -> NTs are released into synaptic cleft and travel to postsynaptic neuron to pass on signal e. Fun example!: think of terminal button as busy shipping centre: i. Mitochondria power the process ii. Vesicles are like tiny delivery trucks, packed with NTs iii. Microtubules are highways that transport vesicles from soma 11. What is the process of neurotransmitter release? a. Step 1: AP arrives i. AP travels down axon to TB at end of neuron ii. Depolarises the TB, like flipping a switch b. Step 2: calcium channels open i. Depolarisation causes voltage-dependent calcium (Ca2+) channels to open in presynapic membrane ii. Calcium ions from outside rush into TB because of diffusion and electrostatic pressure iii. Calcium is like “trigger” that starts release NTs c. Step 3: vesicles release NTs i. Inside TB are synaptic vesicles – tiny sacs filled with NTs ii. Docked vesicles near membrane fuse with presynaptic membrane, creating fusion pore (like a hole) iii. NTs spill out into synaptic cleft through process called exocytosis d. Step 4: vesicle recycling, 4 types: i. Kiss and run: vesicles briefly release their contents, close up, and get refilled ii. Merge-and-recycle: vesicles merge fully with the membrane, and new vesicles pinch off from the membrane iii. Bulk endocytosis: large parts of the membrane fold inward and break off, forming new vesicles e. Vesicle pools, 3 types: i. Ready-release pool (less than 1%): docked vesicles release NTs quickly (used during low rate/low activity) 1. Analogy: snack that is right next to you when you’re hungry, you can grab it instantly ii. Recycling pool (10-15%): used when firing increases (moderate activity) 1. Analogy: snacks in the kitchen pantry, they’re close, but you need to restock the table first iii. Reserve pool (85%-90%): backup vesicles for high firing rates 1. Analogy: snacks stored in garage or basement, only use them if everything else is gone 12. Contrast ionotropic and metabotropic receptors. a. Receptors = proteins in the postsynaptic membrane that NTs bind to b. When NTs attach to these receptors, they open ion channels that allow specific ions to flow into or out of the cell c. This changes the membrane potential and can either: i. Depolarise the cell (make it more positive = excitation) ii. Hyperpolarise the cell (make it more negative = inhibition) d. Ionotropic receptors (direct method) i. Have built-in ion channels with their own binding sites ii. When a NT binds, the ion channel opens immediately iii. Fast and simple- works quickly but doesn’t last long iv. Like a key turning a lock: NT (key) -> ion channel (lock) opens v. Analogy: ionotropic receptors are like automatic doors – the NT directly triggers the door to open e. Metabotropic receptors (indirect method) i. Do not directly open ion channels, instead, they start a chain reaction using a G protein ii. Steps: 1. A NT binds to receptor 2. Receptor activates G protein (next to it) 3. G protein activates enzyme that creates a second messenger (like cyclic AMP) 4. Second messenger travels through cell and opens ion channels or triggers other changes iii. Slower to act but has longer-lasting effects iv. Second messengers can also affect gene expression and trigger biochemical changes in cell v. Analogy: metabotropic receptors are like ringing a doorbell – you press the button (NT), and a chain reaction inside the house (G protein, second messenger) eventually opens the door 13. Compare EPSPs and IPSPs in postsynaptic cells. a. Excitatory: depolarises the cell -> brings it closed to firing (EPSP) i. Ion channel: Sodium (Na+) channels ii. Sodium Na+) rushes into the cell because there’s more Na+ outside iii. This makes the inside less negative (depolarisation) iv. Neuron gets closer to the threshold, increasing chance of firing v. Analogy: EPSPs are live revving up a car engine – it prepares the neuron to “go”! b. Inhibitory: hyperpolarises the cell -> makes it harder to fire (IPSP) i. Ion channels: Potassium (K+) or chloride (Cl-) channels ii. K+ leaves the cell -> makes inside more negative (hyperpolarisation) iii. Cl- enters the cell -> neutralises any positive depolarisation iv. Neuron gets farther from threshold, decreasing chance of firing v. Analogy: IPSPs are like hitting the brakes – slowing things down and preventing firing c. Calcium (Ca2+) channels -> act like sodium ions, they flow into the cell i. Produces an EPSP (depolarisation) ii. Bonus role: calcium can also activate enzymes inside the postsynapic neuron, causing changes like: 1. Structural changes in cell 2. Triggering other biochemical processes 14. Summarise neural integration of EPSPs and IPSPs. a. Neural integration = balancing act between excitatory and inhibitory inputs on a neuron i. EPSPs: push the neuron closer to firing an AP ii. IPSPs: push the neuron away from firing b. If excitatory synapses dominate: i. Many EPSPs are generated (depolarisation), making the neuron’s membrane potential rise above the threshold of excitation ii. This triggers an AP, and the neuron fires c. If inhibitory synapses dominate: i. Many IPSPs are generated (hyperpolarisation), which cancels out the EPSPs and keeps the membrane potential below threshold ii. The neuron does not fire an AP d. If both EPSPs and IPSPs are active: i. EPSPs and IPSPs interact, and their effects can cancel each other out ii. Whether neuron fires depends on the stronger input e. Example: i. If excitatory neurons want to make you perform a behaviour (like moving arm) and at the same time, inhibitory neurons try to block that behaviour -> behaviour that happens depends on whether excitatory or inhibitory inputs win out f. Behavioural inhibition can be confusing because inhibiting inhibitiory neurons can actually lead to excitation i. Example: if inhibitory neurons that prevent movement are turned off, the movement becomes more likely to occur ii. Fun interpretation: 1. EPSPs vote for neuron to fire 2. IPSPs vote for neuron to stay quiet 3. Outcome = AP or no AP depends on which inputs win 15. How are postsynaptic potentials terminated? a. EPSPs or IPSPs caused when NTs activate receptors on the postsynaptic membrane i. To avoid overstimulation, these effects must be terminated quickly b. Two mechanisms of postsynaptic potential termination: i. Reuptake: 1. NTs are removed from synaptic cleft and taken back into presynaptic TB 2. Special transporter molecules use cell’s energy to pull NT molecules into cytoplasm – quick process, giving postsynaptic receptors only brief exposure to NTs 3. Analogy: reuptake is like vacuuming up spilled confetti after a party, it quickly cleans up the mess ii. Enzymatic deactivation 1. An enzyme in the synaptic cleft breaks down NTs into inactive parts 2. Example: enzyme acetylcholinesterase (AChE) breaks down the NT acetylcholine (ACh) into choline and acetate, which cannot activate receptors 3. Ensures that signal is short-lived and doesn’t overstimulate muscle or neuron 4. Analogy: disassembling a puzzle – the pieces (choline and acetate) can no longer function together iii. Example of myasthenia gravis: 1. Immune system destroys ACh receptors on muscle cells, causing muscle weakness 2. Treatment: drugs that block AChE (e.g., acetylcholinesterase inhibitors) -> increases amount of ACh available in the synaptic cleft, helping the remaining receptors get enough ACh to send messages to the muscles -> muscle function improves, as mor ACh floods the receptors 16. Distinguish autoreceptors from postsynaptic receptors. a. Postsynaptic receptors: located on postsynaptic membrane; detects NTs in synaptic cleft and trigger EPSPs or IPSPs by opening ions channels -> membrane potential of the postsynaptic cell i. Analogy: postsynaptic receptors are like a doorbell – when NTs bind, they trigger a response (open the “door” for ion flow b. Autoreceptors: found on presynaptic membrane (same neuron that releases neurotransmitter), usually on TB; monitor and regulate amount of NT released into synaptic cleft -> usually inhibit production or release of NTs to prevent excess, do not change membrane potential (don’t open ion channels) i. Act as part of negative feedback system to maintain balance 1. If too much NT is released -> autoreceptors slow down synthesis/release 2. If too little is released -> increase synthesis/release 3. Analogy: autoreceptors are like a thermostat -> they monitor and adjust the “temperature” (NT levels) to keep things balanced c. Key takeaway: postsynaptic receptors receive messages and cause the postsynaptic neuron to fire or not fire, while autoreceptors act like internal monitors for the presynaptic neuron, ensuring NT levels stay balanced to avoid over- or under-communication 17. Analogy for AP steps: a. S - Sodium Channels Open → Sodium (Na⁺) rushes in (Depolarization begins). b. P - Potassium Channels Open → Potassium (K⁺) starts to leave (a bit slower). c. K - Kicking Sodium Out → Sodium channels close and go refractory (Na⁺ can't get back in). d. N - Now Potassium Leaves → Potassium fully exits, causing repolarization. e. P - Potassium Channels Close → Too much potassium leaves → Hyperpolarization (Oops, overshot!). f. R - Return to Resting Potential → Sodium-Potassium Pump restores balance (3 Na⁺ out, 2 K⁺ in). i. Story version: Imagine a group of penguins trying to kick naughty puppies out of their icy igloo. First, the sodium puppies rush in, causing chaos. Then, the potassium penguins start kicking them out one by one. The penguins get so overzealous that they kick too many puppies out (hyperpolarization!), but eventually, they calm down and restore balance to their igloo. 18. Identify synapses other than those involved in neural integration. a. Axoaxonic synapses: found on axons; adjust amount of NT released by presynaptic axon’s terminal buttons i. Presynaptic inhibition: decreases NT release ii. Presynaptic facilitation: increases NT release iii. Regulate communication strength between neurons without directly influencing whether the neuron fires iv. Analogy: like turning the volume up or down on a speaker b. Dendrodendritic synapses: found between dendrites of two neurons; have regulatory roles and help organise and coordinate neuron activity i. Chemical synapses: use NTs and vesicles (like typical synapses) ii. Electrical synapses (gap junctions): membranes of two neurons almost touch, allowing ions to pass directly between cells -> fast communication and synchronised activity iii. Found in areas like retina, hippocampus, and hypothalamus iv. Analogy: Chemical dendrodendritic synapses are like passing a note, electrical synapses are like two rooms connected by a door, where people can move freely back and forth 19. Describe examples of nonsynaptic communication. a. Neuromodulators: chemicals released by neurons that affect activity of many neurons over larger area i. Released in larger amounts and travel farther than NTs, do not bind to specific synapses and diffuse widely ii. Modulate general behaviour states like vigilance, fearfulness, sensitivity to pain iii. Example: peptides (chains of amino acids) b. Hormones: chemicals released by endocrine glands (like ovaries, testes, etc) or organs (stomach, brain, etc) i. Released into bloodstream and distributed throughout body ii. Only target cells with specialised hormone receptors respond 1. Peptide hormones a. Bind to metabotropic receptors on cell membrane b. Activate second messenger, which triggers changes in the cell’s processes (like sending signals to the nucleus) 2. Steroid hormones a. Small, fat-soluble molecules (sex hormones like testosterone) b. Can pass through cell membranes easily c. Travel to nucleus and attach to receptors, altering cell’s protein production iii. Nonsynaptic effectors on neurons 1. Some steroid hormone receptors are found in terminal buttons and postsynaptic membranes -> rapidly influence synaptic transmission, but exact mechanism is still unknown Cognitive Psychology Syllabus Chapter 1 What are the historical foundations of cognitive psychology? What is the (short) history of biological psychology? What can be said about the speed of information processing? What can be said about the field of cognitive neuroscience? Cognitive Psychology Syllabus Chapter 2 What are the reaction time methods? - Posner task: respond with key press to appearance of stimulus on screen o Examines effect of allocation of attention on response o Reaction times shorter when target is presented at cued location o Attention shifts from location to location to detect target - Visual search task: determine whether specified target object is presented on the display o Ps press one key when target is present and another key when target is absent -> crucial factors that are manipulated are the nature of other objects (non- targets) and number of objects presented o Two types of visual search: feature search and conjunction search ▪ Feature search: target distinguished from non- target on basis of single feature ▪ Conjunction search: all features of target shared by at least one of non-targets ▪ Examine: do reaction times increase as a function of the number of objects? -> reaction times increase as function of set size in conjunction search task, but NOT in feature search task -> attention not required to find the target ▪ Adding more non-targets makes no difference What are event-related potentials? - Cognitive activity in response to a given event -> average of many trials to average out noise - P1/P2: order in which events occur (P1 before P2) - N400/N600: time at which it occurs (400 ms or 600 ms after stimulus representation) - P1 -> detection of stimulus - N400 -> semantic information of stimulus - Example through Luck and Ford’s selective attention task: reaction times shortest when target is presented at attended location, but with their experiment the question is what effect attention has on ERP components o Attention has an effect on P1 and N1 components, but not on C1 component -> attentional modulation does not occur at earliest processing stage but later o => Attention improves processing o Relatively early effect on ERP components -> between 100 and 200 ms - McLaughlin and Bersick “surprise” word prediction: surprise word after for example “I need my umbrella, because it’s...” expected would be raining, but a surprise word appears instead o Semantic surprise -> N400 amplitude affected o Syntactic surprise -> P600 affected o -> It takes longer to process the syntactic anomaly than the semantic anomaly - Temporal resolution of ERPs very high, spatial low What are the various brain imaging methods? - PET and fMRI: poor temporal, good spatial; indirect measurements o Measure blood flow in brain o Brain region active? -> increased flow of oxygenated blood to brain region - PET: radioactive tracer injected in bloodstream - FMRI: measures changes in magnetic properties as result of presence of oxygen in blood - Petersen et al. PET study: subtracting conditions of brain activity -> to examine which brain region is involved in retrieving meaning of word from memory -> left inferior frontal gyrus, motor areas, occipital-temporal junction - Ganis et al. FMRI study: does mental imagery require perceptual processes? (imagery of tree vs shown a tree) o More activity in posterior regions of temporal and occipital in perception condition o Visual processing regions more involved in perception than in imagery o Visual processes might also be involved in imagery condition o Question: is activity in occipital lobe actually needed for performing mental imagery? Brain imaging cannot provide this information What is transcranial magnetic stimulation? - TMS: are certain brain regions causally involved in certain cognitive functions? o Disrupt functioning of brain region to see effect on task performance o Large coil on brain region, magnetic pulse o Kosslyn et al. TMS study: TMS pulses applied to region of occipital lobe during perception/imagery response time task -> if this part of occipital lobe is involved in perception and also imagery, then performance in imagery condition should be impaired in real TMS condition relative to sham TMS ▪ Imagery and perception impaired ▪ Showed that brain imaging studies show which regions activated in task, while TMS can show whether activity is causally related to task performance What is the evidence for and against the various brain imaging methods? - TMS: causal involvement of brain region in certain task - ERP: how cognitive processes are affected by certain factors -> good time course of cognitive processes - But Martinez MRI and ERP attention task showed C1 is unaffected by atention, while attention affected brain activity in V1 when measured by brain imaging Carlson & Birkett Chapter 4 1. Define and differentiate the following terms: drug, drug effect, and site of action. a. Drug = an exogenous (produced outside the body canceling out body NTs) chemical not necessary for normal cellular functioning that significantly alters functions of certain cells of the body when taken in relatively low doses b. Drug effects = changes observable in individual’s physiological processes and behaviour c. Sites of action = locations where drug molecules bind with molecules located on or in cells of the body to affect some biochemical processes of these cells 2. What are the steps of pharmacokinetics? a. Absorption i. Intravenous injection – injected into vein; immediately reaching brain within a few seconds ii. Intraperitoneal injection – through abdominal wall into peritoneal cavity (space surrounding stomach, intestines, etc) iii. Intramuscular injection – into large muscle; absorbed into bloodstream through muscle capillaries iv. Subcutaneous injection – beneath the skin v. Intracerebroventricular administration – deliver antibiotics straigjt to brain to treat certain types of infections; very rapid effects vi. Oral administration – absorbed into blood through digestive tract vii. Sublingual administration – drug under tongue; absorbed into bloodstream through capillaries supplying mucous membranes lining mouth viii. Inhalation ix. Topical administration b. Distribution i. Molecules that are soluble in lipids pass through cells lining capillaries in CNS, rapidly distributing themselves throughout the brain c. Metabolism & excretion i. Metabolised and deactivated by enzymes and excreted mainly by kidneys 3. Why do drugs vary in effectiveness and how can these differences be measured? a. Affinity = readiness with which two molecules join together for the sites to which drugs attach i. Most useful drugs have high affinity for sites of action that produce therapeutic effects and low affinity for sites of action producing toxic side effects ii. Dose-response curve: used to measure effectiveness of a drug; mg of drug/kg of person, effects of drug plotted b. Therapeutic index = margin of safety measure, obtained by administering varying doses of drug to group of lab animals or human volunteers, two numbers obtained: i. Dose producing desired effects in 50% of individuals ii. Dose producing toxic effects in 50% of individuals iii. Therapeutic index = ratio of the two numbers iv. The lower the therapeutic index, the more careful doctors have to be in prescribing drug 4. Differentiate between tolerance, sensitisation, and withdrawal effects following repeated use of a drug. a. Tolerance = after repeat administration, effects diminish i. Withdrawal symptoms may develop when stopping the drug ii. Both tolerance and withdrawal are the body trying to compensate for the drug’s effects b. Sensitisation = drug becomes more and more effective the more it’s administered 5. Describe a placebo and the placebo effect. a. Placebo = inactive substance; administering placebo may produce physiological or psychological effect if an expected effect is in mind 6. How can drugs affect neurotransmitter synthesis? a. 1) Drug serves as a precursor (agonist) -> drug acts as building block for NT synthesis, helping neuron make more NTs -> L-Dopa increases dopamine i. Analogy: supplying extra ingredients to bake more cookies (NTs) b. 2) Drug inhibits synthesis of NT (antagonist) -> drug blocks enzymes needed to make NTs so fewer NTs are produced -> PCPA inhibits serotonin production i. Analogy: breaking the oven so no cookies (NTs) can be baked c. 3) Drug prevents storage in vesicles (antagonist) -> NTs are not stored in vesicles so they can’t be released -> reserpine affects monoamines (like dopamine, norepinephrine) i. Analogy: stopping cookies from being packed into boxes (vesicles) for delivery d. 4) Drug stimulates release of NT (agonist) -> drug forces NTs to be released into synapse -> black widow spider venom causes release of ACh i. Analogy: shaking the cookie box until everything spills out e. 5) Drug inhibits release of NT (antagonist) -> drug blocks NT release from vesicles -> botulinum toxin stops release of ACh i. Like sealing the cookie box shut so nothing comes out f. 6) Drug stimulates postsynaptic receptors (agonist) -> drug mimics NT and activates postsynaptic receptors - > nicotine mimics ACh i. It’s like a fake key that opens the door (receptors) just like the real one g. 7) Drug blocks postsynaptic receptors (antagonist) -> drug blocks NTs from binding to receptors -> curare or atropine block ACh i. It’s like jamming the keyhole so the door (receptor) can’t open h. 8) Drug stimulates autoreceptors (antagonist) -> drug activates autoreceptors, which stop the release of NTs -> apomorphine reduces dopamine release i. It’s like pressing a “stop” button to shut down cookie deliveries i. 9) Drug blocks autoreceptors (agonist) -> drug blocks autoreceptors so neuron keeps releasing more NTs -> idazoxan increases norepinephrine release i. Analogy: it’s like disabling the “stop” button so cookies keep flowing j. 10) Drug blocks reuptake (agonist) -> drug prevents NTs from being reabsorbed, leaving more in synapse - > cocaine blocks dopamine reuptake i. It’s like stopping workers from vacuuming up spilled cookies so more remain outside k. 11) Drug inactivates enzymes (agonist) -> drug blocks enzymes that break down NTs, leaving more in synapse -> physostigmine stops AChE from breaking down ACh i. It’s like breaking the cookie-crushing machine so the cookies stick around longer 7. How do drugs affect neurotransmitter storage and release? a. Vesicle transporters = pump molecules of NT across vesicle membranes, filling the vesicles -> like workers packing the clean-up cookies into boxes (vesicles) so they’re ready to be delivered during the next action potential i. Blockers of vesicle transporters are antagonists, as they bind with a particular site on transporter and inactivate b. Terminal transporters: like vacuums, they clean up NTs and bring them back into the cell c. Some drugs act as antagonists by preventing release of NTs from terminal button -> deactivate proteins that usually cause docked synaptic vesicles to fuse with presynaptic membrane and expel contents into synaptic cleft (example Botox prevents release of ACh) 8. What are the effects of drugs at the receptor? a. Depends on location, what normal effects usually are, and whether drug activates receptor or blocks actions b. NT released -> must stimulate postsynaptic receptors - > some drugs bind with these, just as NT does -> once bound, serves as either agonist or antagonist i. Drug mimics effect -> direct agonist-> molecules of drug attach to binding site where NT normally attaches -> binding causes receptor to respond in same way when NT is present ii. Drug binds with postsynaptic receptor but blocks them from being activated -> prevent NT from activating receptor -> receptor blockers or direct antagonists 1. Some postsynaptic receptors have multiple binding sites, molecules of NT bind with one site and other substances bind with others -> when molecule binds with one of these alternative sites -> noncompetitive binding 2. If drug attaches to an alternative site and prevents ion channel from opening -> indirect antagonist (example PCP and ketamine 3. Effect of indirect antagonist and direct antagonist the same, but site of action is different iii. If drug attaches to alternative site and facilitates opening of ion channel -> indirect agonist (example Diazepam) c. Presynaptic membranes of some neurons have autoreceptors that modify the activity of presynaptic cell -> afgonist molecules at these receptors usually result in reduced synaptic communications & drugs selectively activating presynaptic receptors are antagonists, while drugs that block presynaptic autoreceptors have the opposite effect, they increase synapgtic communication -> agonist 9. What are the effects of drugs on NT reuptake and deactivation? a. Drugs that block reuptake or enzymatic of NTs are agonists 10. What are the features of the amino acid NT systems? a. Glutamate: excitatory effects i. Synthesised from precursor glutamine by enzyme glutaminase in one step -> after synthesis glutamate stored in vesicles -> glutamate is released from presynaptic neuron following action potential ii. Four major types of glutamate receptors, three ionotropic 1. NMDA/G-protein: opens sodium and calcium channels, but only under certain conditions a. Multiple binding sites -> glutamate binds to open receptor and glycine must also bind to help open channel b. At resting potential, magnesium blocks the channel c. For the channel to fully open, glutamate must bind and the membrane must be depolarised (excited) -> magnesium is repelled d. Alcohol blocks NMDA receptors, reducing activity e. PCP and ketamine bind to special site on NMDA and block calcium flow (indirect antagonists) f. Key for memory formation g. If glutamate isn’t removed properly -> overexcitation of neurons -> damage or death (conditions like strokes and ALS linked to this) h. Drug example: riluzole reduces glutamate release and helps in ALS treatment 2. AMPA: opens sodium channels -> create EPSPs 3. Kainate: similar to AMPA iii. One metabotropic receptor 1. Works indirectly by activating G-proteins, slower but longer-lasting effects 2. Some act as autoreceptors, regulating how much glutamate gets released b. GABA: inhibitory effects i. Prevents neurons from overfiring, stabilising brain activity ii. Without GABA, too much excitation would lead to seizures iii. Made from glutamic acid using enzyme GAD - > GABA packed into synaptic vesicles by vesicle GABA transporters -> when AP arrives, GABA released into synapse 1. GABAA receptor: ionotropic receptors and controls chloride channels -> when GABA binds, chloride ions flow into cell -> hyperpolarisation -> neuron is inhibited (less likely to fire) a. 5 binding sites c. Glycine: inhibitory, found in spinal cord and lower brain stem 11. What is the acetylcholine system? a. Used in CNS and PNS b. Controls muscle contractions and is involved in learning, memory, sleep c. Key pathways: i. Dorsolateral pons: 1. Role: regulates REM sleep ii. Basal forebrain 1. Activates cerebral cortex, supports learning and perception iii. Medial septum 1. Control hippocampus rhythms -> important for memory formation d. Botox blocks ACh release -> causes paralysis by stopping muscle contraction e. Black widow spider venom -> leads to overcontraction of muscles -> can be fatal in high doses f. Nicotinic receptors (ionotropic): i. Found in PNS, in muscles ii. Fast activation -> muscle contractions and quick responses g. Muscarinic receptors (metabotropic): i. CNS ii. Slower, longer-lasting effects through second messengers h. Myasthenia gravis i. Immune system attacks ACh receptors on skeletal muscles -> weak muscles ii. Treatment: Neostigmine (AChE inhibitor) increases ACh levels at receptors to improve muscle strength 12. What are the key features of the monoamine systems? a. Monoamines: group of classical NTs i. Dopamine, norepinephrine, serotonin ii. Have small cell bodies located mostly in brainstem, but their axons branch out widely to influence large areas of brain b. Dopamine: i. Dopamine receptors: D1, D2, D3, D4, D5 1. D1 & D5: stimulate production of cyclic AMP 2. D2, D3, D4: inhibit production of cyclic AMP 13. Contrast the features of peptide NTs with classical NTs. a. Whereas the classical neurotransmitters are synthesized from relatively small amino acid precursors, peptide neurotransmitters are synthesized from large polypeptides. Both peptides and classical neurotransmitters are stored in vesicles and can be co- released following an action potential. Peptide neurotransmitters can function as either neurotransmitters or neuromodulators to regulate the sensitivity of receptors to classical co-released neurotransmitters. 14. What are the features of the lipid NT systems? a. Lipid neurotransmitters are synthesized on demand and are not stored in vesicles. Endocannabinoids bind to CB1 and CB2 receptors. Anandamide is deactivated by the enzyme FAAH within the presynaptic neuron, and the anandamide transporter is responsible for the reuptake of anandamide to the presynaptic cell. Carlson and Birkett Chapter 8: Control of Movement 1. Describe the structures of a skeletal muscle. a. Flexion: contracction of flexor muscles-> moving limb towards body b. Extension: contraction of extensor muscles -> moving limb away from body -> antigravity muscles (muscles we use to stand up) c. Extrafusal muscle fibres: served by axons of alpha motor neurons -> contracting these fibres provides muscle’s motive force d. Intrafusal muscle fibres are specialised sensory organs that are served by two axons, one sensory and one motor, also called muscle spindles e. Intrafusal muscle fibres found within spindles, extrafusal found outside them i. IFs are proprioceptors -> tell us position of muscle, tendons, joints, ligaments ii. Pick up length/strength of muscle + speed of which it’s being stretched iii. Nuclear bag fibers: length and velocity iv. Nuclear chain fibres: only length v. Gamma motor neurons cause muscle to contract by releasing acetylcholine -> lengthens/stretches 2. What are the steps of neurotransmission at the neuromuscular junction? a. Synapse between terminal button of efferent neuron and motor endplate on muscle fiber -> like meeting point where neurons tell muscles to contract b. Steps of neurotranmission at the neuromuscular junction: i. AP arrives -> travels down motor neuron to its terminal button ii. ACh release -> terminal button releases ACh into synaptic cleft iii. Endplate potential -> ACh binds to receptors on motor endplate -> causes depolarisation (endplate potential) 1. Unlike normal EPSP, endplate potential is strong enough to always cause the muscle fibre to fire iv. AP in muscle fibre -> AP propagates along length of muscle fibre v. Calcium channels open -> AP opens voltage- dependent calcium channels -> calcium enters muscle cell’s cytoplasm vi. Calcium triggers contract -> calcium acts as a cofactor, it helps myofibrils (tiny muscle units) use energy from ATP 1. Causes myosin cross-bridges to attach to actin filaments 2. Myosin then rows along the actin like a paddle, pulling the filaments and shortening the muscle (causing contraction) c. What happens during contraction? i. Cross-bridge cycle: myosin cross-bridges attach, bend, detach, and reattach to actin -> rowing motion -> continuous rowing shortens muscle fibre which causes contraction d. Muscles never push, they always pull -> muscle that connects from bone to bone always has to pull because the muscle cannot push itself beyond its size/bounds e. Whatever one muscle does, another muscle can undo f. Motor unit = group of muscle fibres that all get their signals from the same, single motor neuron -> only listen to one neuron, so they work together for that one neuron g. What happens during a twitch? i. When calcium is being pumped back into the sarcoplasmic reticulum (during relaxation period) ii. If another AP travels down before that can happen, even more calcium gets released, expositing more actin for myosin to bind to -> more force in that fibre -> twitches add to reach other as they get closer together in time -> = temporal summation iii. At some point there’s a limit, regardless of how much more calcium or faster APs – when all little twitches blend together until they feel like one gigantic contraction, that’s called tetanus -> meet maximum of tension -> myosin and calcium pumps are burning up the muscle cells’ ATP -> the limited supply of ATP is what makes it impossible to maintain vigorous muscle activity forever -> if exerted it can lead to muscle fatigue (twitching happens in individual motor units, so frequency is only way to create grade of force bc APs always fire with same strength – each twitch happens at slightly different time, making muscle movement smooth, nuanced) h. Isotonic movement = changing length of muscles involved (like lifting a mug) i. Isometric: no change in length (large build-up of tension but no contraction; like trying to lift a building) 3. Analogy for muscle contraction: a. A signal (action potential) tells workers (myosin) to row boats (actin filaments) b. The workers use calcium and ATP to fuel the rowing c. More workers rowing faster → stronger contraction d. When the signal stops, calcium is cleared away, and the workers slow down 4. Contrast the roles of receptors for sensory feedback from muscles. 5. Explain the function of monosynaptic stretch reflexes. 6. Explain the function of the gamma motor system. 7. Contrast polysynaptic and monosynaptic reflexes. 8. Describe how motor control information is processed in the cortex. 9. Describe the functions of the motor association cortex in planning and initiating movement. 10. Describe the functions of subcortical regions involved in control of motor behaviour. 11. Describe the components of the descending pathways. 12. Describe the location, components, and functions of the mirror neuron system. 13. Summarise the contributions of the parietal cortex in reaching and grasping behaviour. 14. Describe how brain lesions can produce limb apraxia. 15. Describe how brain lesions can produce constructional apraxia. 16. Describe the biological basis of dyspraxia. From lecture: Muscle spindle 1: static muscle length - Arm lowers slowly in passive way - MS1 fires steadily because it detects change in overall muscle length - MS2 and GTO stay relatively quiet because there’s no sudden movement or significant tension change - How stretched muscle is MS2: responds to fast changes in muscle length - Arm is dropped abruptly - MS2 fires rapidly because it responds to the fast change in muscle length - MS1 keeps firing steadily to monitor muscle position - GTO stays mostly quiet since tension hasn’t dramatically increased yet - Reacts to quick changes Golgi: sensory receptors that detect muscle tension - Weight is added to the hand - Arm drops slightly, then comes back into position - GTO fires rapidly because it detects increase in muscle tension when weight is added - MS1 fires steadily as it monitors the new muscle length as the arm adjusts - MS2 fires briefly during fast adjustment - Monitors how much tension the muscle is experiencing Steps to the monosynaptic stretch-reflex: - Stretch detection: MS detect when muscle is being stretched o Stretch triggers sensory neurons to send signal to the spinal cord through the dorsal root - Signal transmission to spinal cord: o Sensory neuron synapses directly onto alpha motor neuron in spinal cord o Single connection (monosynaptic) makes reflex extremely fast because there’s no delay involving brain - Alpha motor neurons send reflexive command: o AMN send signal back to same muscle that was stretched o Causes muscle to contract, counteracting stretch and preventing overstretching or damage - Maintains posture and stability of body - Polysynaptic reflex: involves more than one synapse (sensory neuron -> interneuron -> motor neuron) - Example: weightlifting o During weightlifting, if GTOs sense excessive tension, they inhibit muscle contraction, making it harder to lift beyond safe threshold o To lift heavier weights, athletes try to “desensitize” GTOs through training, allowing muscles to handle greater tension - Reflex pathway o GTO detects excessive muscle tension o Afferent signal -> spinal cord -> inhibitory interneuron o Inhibition of alpha motor neurons o Reflexive muscle relaxation to prevent damage 1. Rubrospinal tract: rude red robot -> limbs only (arms and legs, NO fingers/toes) 2. Corticobulbar tract: bulb lights up the face -> neck, face, eyes, and tongue 3. Lateral corticospinal tract: later alligator moves it all -> arms, hands, fingers, legs, feet and toes 4. Ventral corticospinal tract: venturing to stay upright -> posture and balance, focusing on trunk and upper leg - Cerebellum: mini brain responsible for coordinating movement, posture balance – contains 80% of brain neurons o Fine tunes and adjusts movements o Keeps posture and balance steady o Ensures smooth, coordinated actions like walking, throwing, writing - Ventromedial system: body posture and orientation, controlling trunk - Lateral system: independent movement of limbs (moving arms or legs individually) (e.g. playing piano) - Damage? To lateral system: problems with timing (agonist- antagonist) muscles results in tremor particularly at end point of movements - SMA not just active for single movement, but specifically for sequences (monkeys did push, pull, and turn, but SMA most active during specific sequences like PUSH-PULL, as graphs on the right show) - Shows that SMA plays critical role in “planning ahead” to execute movements in correct order -> integrates sensory information and predicts movement outcomes -> essential for smooth, coordinated actions like typing, playing instruments or athletics - Damage -> impairs ability to remember or execute complex motor sequences - Premotor cortex o Plans and executes movements based on external signals like lights or sounds o Contains mirror neurons that help with learning movements by imitation o Helps create associations between arbitrary signals (like a green light) and correct movement o Essential for responding quickly and correctly to external cues in complex environments Apraxia: - Inability to carry out learned, complex actions without any paralysis or motor weakness -> caused by damage to frontal or parietal lobes Limb apraxia - Damage to left parietal lobe - Characteristics: o Misuse of body parts for actions o Correct body parts but movement wrong o Movements occur in wrong sequence o Difficulty imitating actions o Improves when tools or real objects are used because it bypasses need for symbolic understanding - Explanation: o Left parietal lobe controls understanding of one’s body o Damage -> disrupts organising movements internally based on instructions Constructional apraxia: - Damage to right parietal lobe - Characteristics: o Difficulty copying figures or drawing geometrical shapes o Trouble asembling objects like building blocks and puzzles o Not related to motor deficits (hand and arms work fine) o It’s a spatial perception problem, not a motor execution issue - Explanation: o Right parietal lobe handles extraperceptual space: recognising how things are positioned in space relative to one another o Damage disrupts spatial organisation -> difficulties assembling or perceiving whole objects o More of a “visual problem” because individuals fail to combine parts into coherent whole - Basal ganglia o Selects and initiates appropriate motor programs, inhibiting inappropriate ones o Key mechanism for smooth and controlled movement o “gatekeeper” for movement ▪ Possible responses enter basal ganglia ▪ Most active response (correct motor command) is facilitated and allowed through o Key parts of basal ganglia: ▪ Caudate nucleus & putamen (together they are the striatum) Receive excitatory input from motor cortex and somatosensory cortex ▪ Globus pallidus (internal and external) Acts as main output structure, sending inhibitory signals to the thalamus and back to the cortex ▪ Subthalamic nucleus: Excitatory input to regulate inhibition ▪ Substantia nigra Dopaminergic input modulates basal ganglia activity o Motor pathways in basal ganglia: ▪ Direct pathway (facilitates movement) Cortex -> striatum -> globus pallidus internal -> thalamus -> cortex Inhibits GPi, which removes inhibition from thalamus and allows motor commands to proceed ▪ Indirect pathway (inhibits unnecessary movement) Cortex -> striatum -> globus pallidus external (GPe) -> subthalamic nucleus -> GPi -> thalamus -> cortex Increases inhibition of the thalamus to suppress competing motor commands ▪ Hyperdirect pathway (emergency brake) Cortex -> subthalamic nucleus -> GPi-> thalamus -> cortex Bypasses striatum to quickly inhibit inappropriate movement o Motor gating ▪ Basal ganglia acts like filter: allows only most appropriate motor signal (response) to be sent to motor cortex ▪ Caudate nucleus and putamen receive possible responses from cortex and send them through direct and indirect pathways to facilitate or inhibit movement ▪ Globus pallidus sends final output to motor cortex via thalamus o Motor disorders ▪ Parkinson’s (loss of dopamine neurons in SN) Overactivation of indirect pathway -> struggle to initiate movement Tremors, rigidity, postural instability Treat with L-Dopa or electrical stimulation STN/Gpi (inhibit indirect pathway) ▪ Huntington’s (loss of GABAergic neurons in striatum) Loss of inhibition from indirect pathway -> excessive, uncontrollable movements (chorea) Involuntary movements, dystonia (cramped posture), athetosis (wiggling toes, ballismus (wild movements), eventual memory loss and mood problems Impaired function of indirect pathway -> increased motor activity (movements due to loss of caudate/putamen neurons that inhibit GPe) o Gating mechanism ▪ Direct path promotes motor activity ▪ Indirect path inhibits motor activity o 2 types of dopamine receptors in caudate nucleus and putamen ▪ D1: excites direct path ▪ D2: inhibits direct path Carlson and Birkett Chapter 9: Sleep and Biological Rhythms Sleep stages - Each cycle lasts approx 90 minutes, 3 stages o Awake state: alpha and beta waves ▪ Alpha activity: 8-12 Hz, moderate amplitude Occurs when awake, relaxed, and not actively engaged Example: quiet wakefulness with eyes closed ▪ Beta activity: 13-30 Hz, low amplitude Associated with alertness, thinking, active information processing Desynchronous waves indicate independent neural activity o Non-REM sleep (NREM, divided into stages 1-3) ▪ Stage 1 sleep: Theta activity (2.5-.7.5 Hz) Transition between wakefulness and sleep Light sleep; easy to wake someone May experience hypnic jerks (brief muscle contractions) and a sensation of falling If you get woken up here, you think you were not asleep yet ▪ Stage 2 sleep: theta activity with sleep spindles (12-14 Hz bursts) and K-complexes Sleep spindles: short bursts of brain activity that aid memory consolidation and protect against waking K complexes: sharp, high-amplitude waves related to neural inhibition Deeper than stage 1, still light enough for arousals ▪ Stage 3 sleep: delta activity ( stage 2 -> stage 3 -> REM sleep As night progresses, REM periods lengthen, deep slow-wave sleep decreases - REM sleep characteristics: o Rapid eye movements o EEG desynchronisation o Ponto geniculo occipital (PGO) waves (just before REM sleep) ▪ Activity from the pons to the lateral geniculate nucleus (LGN) of the thalamus and finally the primary visual cortex ▪ Visual system: eye movements/dreaming o Paresis of skeletal muscles o Increased genital activity (also in little children, not erotic) o Strong suppression of external stimuli (except for meaningful sounds) o Narrative, storylike dreaming o At waking you’re immediately alert o Chance of dream recall 75-95% o High probability of waking up spontaneously ▪ No logic continuity in space or time (caused by absent frontal lobe activity) - Slow-wave sleep characteristics o Strong suppression of external stimuli o Low probability of waking up spontaneously o At waking, drowsy and disoriented o Chance of dream recall is 20% o Fragmentary dreams (unrelated snapshots) with strong emotions o Nightmares - Sleep deprivation can lead to: o Loss of thermoregulation o Increased fat and sugar metabolism o Severe weight loss o Immune system disruption o Death - However, in some people, after voluntary deprivation of more than 10 days, it seems to be no problem for physical efforts/no physiological stress -> physical recovery not main function of sleep o Does lead to cognitive problems (concentration/perception/hallucinations) o Sleep required for recovery of brain o If sleep occurs after period of deprivation, there is no complete catch-up, but sleep is regained for stages 4 and REM o Stage 4 (slow-wave) sleep and REM most important - Function of slow-wave sleep o Primarily restorative o Build sugar supply (glycogen in astrocytes: fuel brain cells o Clearing of chemicals accumulated due to brain activity ▪ Adenosine inhibits neural activity when glycogen is low o Learning (especially consolidation of declarative memory) -> remember events you can talk about - Function of REM sleep o Alertness (vigilance) o Brain development o Learning, especially non-declarative -> example: hold a picture in front of a mirror and learn to draw it correctly o Reduction in the proportion of REM sleep with age - Physiological mechanisms of sleep o Sleep/waking controlled by accumulation of chemicals o Production during wakefulness of a chemical that makes you sleep o Adenosine accumulates in brain during waking -> inhibits cells when energy is low - Sleep regulation o Adenosine ▪ Key sleep-promoting substance ▪ During wakefulness, adenosine accumulates in extracellular space of brain -> inhibits neural activity, promoting drowsiness and slow-wave sleep o Astrocytes release adenosine when neurons are metabolically active ▪ High brain activity uses glycogen stored in astrocytes, leading to adenosine build-up ▪ During slow-wave sleep, adenosine levels decrease, and glycogen levels replenish ▪ Caffeine blocks adenosine receptors, preventing drowsiness o In dolphins, each brain hemisphere can sleep independently -> sleep regulation can be localised - Neural control of arousal o Acetylcholine (ACh): ▪ Promotes cortical arousal and desynchrony ▪ Found in basal forebrain, pons, and medial septum ▪ Active during waking and REM sleep (high cortical activation) o Norepinephrine (NE): ▪ Released from locus coeruleus in dorsal pons ▪ Promotes vigilance (alertness and attention) ▪ High during wakefulness, low during sleep (especially REM) o Serotonin (5-HT): ▪ Released from raphe nuclei (brainstem) ▪ Promotes ongoing activities (like walking or grooming) ▪ High during wakefulness, low during REM sleep o Histamine: ▪ Found in tuberomammillary nucleus (TMN) of the hypothalamus ▪ Activates cortex indirectly via acetylcholine ▪ Blocked by antihistamines (leading to drowsiness) o Orexin: ▪ Produced by neurons in the lateral hypothalamus ▪ Stabilises sleep/waking flip-flop (prevents abrupt switching between states) ▪ Activated by hunger signals and inhibited by satiety or adenosine build-up - Sleep/waking flip-flop model o Brain uses flip-flop mechanism to switch between: ▪ Wakefulness (arousal systems are active; vIPOA is inhibited) ▪ Sleep (vIPOA active; arousal systems are inhibited) VIPOA: ventrolateral preoptic area o Sleep neurons that release GABA to inhibit arousal systems Orexinergic neurons stabilise the flip-flop mechanism, preventing unwanted transitions If orexin not working, can cause unstable wakefulness (transitions between sleep and wakefulness), can result in sudden sleepiness (like narcolepsy), ▪ Summary: for sleep, arousal systems inhibited (GABA) by neurons in vIPOA – for waking, vIPOA is inhibited by arousal systems -> flip- flop ▪ Advantage flip-flop: fast transition between sleep and wakefulness ▪ Disadvantage: can be unstable, you suddenly fall asleep or wake up many times (narcolepsy) ▪ Orexin neurons stabilise flip-flop, can activate arousal systems and keep you awake ▪ Sleep stimulated by satiety signals (through inhibition of orexin neurons) ▪ Waking stimulated by biological clock and hunger signals (through activation of orexin neurons) ▪ REM sleep almost always follows period of slow-wave sleep (NREM stages) - REM sleep flip-flop o Like a flip-flop with two positions: ▪ On position (REM-ON): controlled by SLD (sublaterodorsal nucleus) ▪ OFF position (REM-OFF): controlled by vIPAG (ventrolateral periaqueductal gray) o How does this work? ▪ 1. slow-wave sleep happens first, and the REM- OFF system is active -> no REM sleep yet ▪ 2. As you transition from slow-wave, REM-ON system starts to fire -> REM sleep begins o So, there’s a mutual inhibition: ▪ When SLD (ON) is active -> vIPAG (OFF) is turned OFF ▪ When vIPAG (OFF) is active -> SLD (ON) is turned OFF ▪ Like two teams taking turns playing, but they can never play at the same time o Neural activity: ▪ SLD neurons fire more and more as you transition to REM ▪ REM sleep components controlled by SLD include: Atonia: paralysis of muscles to prevent acting out dreams Rapid eye movements Genital activivty and cortical arousal ▪ Interaction SWS x REM flip-flops 1. Sleep starts when vIPOA (ventrolateral preoptic area) is activated o VIPOA inhibits arousal system (norepinephrine, serotonin, etc) and REM-OFF system (vIPAG) o This turns off wakefulness and rem- off regions 2. REM sleep kicks in o When SWS deep enough, REM-ON region (SLD) activates o REM-OFF stays inhibited by vIPOA o REM sleep begins o Emotional stimuli and amygdala ▪ Amygdala can also activate REM-ON region ▪ Brain interprets emotion as start of a dream - Sleep disorders o Slow-wave sleep disorders ▪ Sleepwalking (somnambulism): unlike REM behaviour disorder, sleepwalkers do not “act out dreams” but perform routine behaviours -> this is because motor systems ae not fully inhibited during SWS ▪ Bed-wetting (nocturnal enuresis): happens due to immature or impaired bladder control during deep SWS ▪ Nightmares (pavor nocturnus): night terrors occur in SWS, particularly in children, and are not remembered as clearly as REM sleep dreams ▪ Cause: instability of sleep flip-flop system ▪ Activation of vLPOA suppresses arousal, but disruptions during transitions can lead to sleepwalking or bed wetting o REM sleep behaviour disorder (RBD) ▪ Impairment in inhibition of motor neurons -> physically acting vivid dreams, can be violent and intense ▪ Mechanism: REM flip-flop system fails Normally, SLD REM ON inhibits motor neurons through inhibitory interneurons, however, in RBD this is disrupted ▪ Cause: degeneration of brainstem regions involved in REM sleep regulation, often linked with neurodegenerative diseases like Parkinson’s - Narcolepsy: o Sleep attacks: irresistible urge to sleep during the day o Cataplexy: sudden muscle weakness (atonia) triggered by strong emotions (laughter, anger) - brain incorrectly activates REM flip-flop, causing atonia during wakefulness o Sleep paralysis: temporary atonia during waking transitions o Hypnagogic hallucinations o Cause: ▪ Loss of orexinergic neurons in lateral hypothalamus -> orexin normally stabilises sleep-wake flip-flop by maintaining arousal ▪ Genetic mutartions or autoimmune destruction can cause loss of these neurons o Strong emotional stimuli activate amygdala-> triggers REM flip flo system -> atonia - Sleep-wake rhythm o Follows 24 hour rhythm (circadian- circa one day) o Light synchronises the clock to 24 hours -> this is a zeitgeber o Other zeitgebers are sound, temperature, social interactions, eat/drink pattern o Internal biological clock (25 hour rhythm) -> localised in suprachiasmatic nucleus SCN of hypothalamus -> SCN cells have their own individual rhythms and “tick” - synchronisation happens through connections between cells and zeitgebers) ▪ Clock mechanism: protein production in SCN cell -> protein enters cell nucleus and inhibits gene causing its own production -> protein concentration decreases and gene becomes active again -> this modulation of concentration influences the firing rate of the cells and causes the “ticking” o Lesions of SCN lead to loss of sleep-wake cycle o Transplantation of SCN-tissue recovers the sleep- wake cycle - Seasonal rhythm o Increased testosterone secretion with longer exposure to light (summer): stimulates reproduction o The pineal gland produces melatonin during night - > light inhibits melatonin production o Longer nights -> more melatonin -> reduced testosterone/more rest and winter sleep Carlson and Birkett Chapter 11, 18: Emotion Nuclei of the amygdala - Amygdala is in temporal love, coordinates emotional responses, particularly fear, through its nuclei o 1. Lateral nucleus: ▪ Receives sensory input (from sensory cortex and thalamus) ▪ Sends information to the basal nucleus and central nucleus ▪ Lazy -> lateral nucleus: lazy because it sits and listens to the sensory input coming in o 2. Basal nucleus: ▪ Bunnies -> relays information to central nucleus or back (bunnies hop around = relay centre) ▪ Middle station in the flow of emotional processing o Central nucleus: ▪ Cry -> outputs emotional responses like fear (crying = big emotional reaction) ▪ Controls behavioural, autonomic, and hormonal responses Stress response: o Lateral hypothalamus -> sympathetic nervous system activation o Dorsal vagal nucleus -> parasympathetic inhibition o Paraventricular nucleus -> HPA axis - Components of emotional response o Behavioural: observable muscular movements (freezing, running away) o Autonomic: physiological responses (increased heart rate, blood pressure) o Hormonal: reinforces autonomic response via hormones (epinephrine, cortisol, etc) - Amygdala and PFC in fear o Lateral nucleus: initiates learning of conditioned emotional responses (pairing a shock with a cue) - remember learning/lateral o Central nucleus: produces fear-related responses (behavioural, autonomic, and hormonal) o VmPFC: extinction of fear responses and reduces conditioned emotional responses by inhibiting amygdala activity – plays a role in impulse control and decision-making - Conditioned fear and extinction o Conditioning o Extinction: learning that stimulus no longer predicts danger -> reduces but doesn’t erase conditioned response o Context-specific: extinction is environment-dependent - Aggression and serotonin o Types of aggression: ▪ Defensive behaviour: threat behaviours to deter an attack ▪ Predatory behaviour: attack without emotional arousal to secure food o Neural circuits: ▪ Amygdala: initiates aggression ▪ Hypothalamus: connects to brainstem regions for defensive and predatory responses o Role of serotonin: ▪ Low serotonin -> increased aggression and impulsivity ▪ High 5-HIAA levels (serotonin metabolite) indicate lower aggression - Emotional memory o Amygdala critical for emotional memories ▪ Damage -> reduced ability to encode or recall emotional memories ▪ Strong emotional responses increase memory retention of events - Aggression in women o Influenced by prenatal and early developmental factors, and hormonal/neural o Prenatal exposure: drugs, alcohol, testosterone o Hormones: high T linked to increased aggression, low cortisol, oxytocin and estradiol may also contribute o Brain activity: ▪ Greater left frontal asymmetry (EEG studies) ▪ FMRI shwos activation amygdala, vmPFC, DLPFC, and other brain areas - T and aggression: o Two effects: ▪ Organisational effect: early T exposure organises brain circuits during development -> impacts behaviour later in life ▪ Activational effect: T increases aggression in adults under certain conditions (competition for status for example) o T promotes dominance and status-seeking behaviours - Role of social context and T o T doesn’t directly cause aggression but it lowers inhibitions (disinhibition) o High T combined with psychological factors (like competition for example) leads to: ▪ Antisocial behaviour -> direct aggression ▪ Prosocial behaviour -> cooperative behaviours like leadership - Hypothalamic pituitary adrenocortical axis (HPA-axis) o Stress signal from amygdala/hippocampus activates paraventricular hypothalamic nucleus o Hypothalamus produces corticotropin releasing hormone (CRH) o CRH stimulates pituitary to secrete adrenocorticotropic hormone (ACTH) in the blood o ACTH stimulates the adrenal gland to produce cortisol o Cortisol breaks down proteins in the muscles to release glucose for physiological alertness and inhibits the hypothalamus and pituitary (suppressing additional cortisol production) - GABA in fear inhibition o Inhibitory NT that calms the amygdala o Prevents overactivation of amygala - Benzos enhance efficiency of GABA connections - SSRIs increase serotonin levels in brain o Serotonin excites GABA-ergic neurons, increasing GABA release - VmPFC lesions -> patients doing social dilemma tasks will choose the “rational” choice, when others are hindered by emotions - VmPFC also regulates the amygdala o Inhibits amygdala activity via neural pathways o Real-life example: Gage had damage to vmPFC and that led to emotional dysregulation, without the proper control the amygdala became overactive, resulting in impulsive or inappropriate emotional behaviour - Also, the influence of memories are important for the context (hippocampus) - Blind children experience facial expressions of emotion the same as sighted children do - Chronic stress leads to overactivation of HPA axis and increased blood cortisol levels - Effects of cortisol: o Cortisol released, goes through feedback loop to eventually then suppress the activity of the HPA-axis, preventing further cortisol production and brings body to homeostasis o In addition to hypothalamus and pituitary, there is feedback to frontal lobe and hippocampus o Inhibition of hippocampus -> reduction of the influence of negative memories to an emotional response - In humans, untreated depression can cause hippocampal volume loss - Effects stress on immune system o Immune system uses lymphocytes: type white blood cells from bone marrow o Lymphocytes communicate using cytokines (interleukins, TNFalpha and interferon) o Cortisol suppresses immune system by interfering with cytokine signals o Saves energy for fighting/fleeing in case of stress o One is then more prone to sickness after stress - Subjective feelings of emotions o James & Lange suggested a theory about feelings paired with emotions -> James-Lange theory ▪ Something happens -> physiological response (like dry mouth, sweating etc) -> emotional feelings arise as a consequence of awareness of these physiological responses ▪ This is counterintuitive, as physiological responses are the cause and not the consequence of emotional feelings - Expression of emotions: face o Spontaneous (automatic) ▪ Initiated by both hemispheres by subcortical regions ▪ Damage to white matter lesion causes emotional paresis o Voluntarily (on command) but difficult to make a realistic expression because we cannot control our orbicularis oculi ▪ Initiated in left motor cortex ▪ Lesion in right motor cortex causes volunary paresis - Feedback of simulated face expressions and physiological rsponse o Subjects asked to make an anxious face with specific bodily instructions, they didn’t know they were making an anxious face, yet it caused changes in the autonomic nervous system (heart rate increase, body temperature) o Humans imitate facial expressions of others – mirror neuron -> may be a mechanism that supports empathy Carlson and Birkett Chapter 6: Vision - Visual system takes up around 25% of brain capacity - Breaking down perception of stimulus: o Colour: hue o Intensity: brightness o Colour mixing: saturation ▪ Exactly 1 wavelength: fully saturated - Eye components: o Conjunctiva: ▪ Thin membrane lining inside of eyelids, merges with sclera (white outer layer of eye) ▪ Function: protects eye and prevents particles from going in o Cornea: ▪ Transparent front layer of eye ▪ Function: allows eye to enter and focuses light onto retina, accounts for most of eye’s focusing power o Iris: ▪ Coloured ring of muscles located behind cornea ▪ Function: controls size of pupil, regulating how much light enters eye o Pupil (opening in iris): ▪ Black circular opening in iris ▪ Function: adjusts size to let in more or less light (dilates in dim light, constricts in bright light) o Lens: ▪ Flexible, transparent structure located behind iris ▪ Function: changes shape through process called accommodation to focus images of near or far objects onto retina o Layers of retina: ▪ Contains photoreceptor cells (rods and cones), bipolar cells, and ganglion cells ▪ Function: detects light and converts it into electrical signals = transduction, which begins visual perception o Fovea: ▪ Small central region of retina containing only CONES ▪ Function: responsible for sharp, detailed, and colour vision (high acuity) - critical for activities requiring precision, like reading o Blind spot: ▪ Located where optic nerve exits retina ▪ Function: area lacks photoreceptors, so no visual information is detected here o Optic nerve (2 cranial nerve) nd ▪ Made up of axons of retinal ganglion cells ▪ Function: carries visual information from retina to LGN in thalamus and then to striate cortex (V1) for processing o Blood vessels: ▪ Supply oxygen and nutrients to retina o Sclera: ▪ Opaque, white outer layer of eye ▪ Function: provides structure and protection for eye o Light enters through cornea -> passes through pupil and lens -> focused onto fovea or peripheral retina o Rods (peripheral vision) and cones (foveal vision) transduce light into signals -> signals travel through optic nerve -> LGN -> primary visual cortex (V1) for processing - Photoreceptors: o Specialised neurons located in retina of eye o Detect light and convert it into electrical signals in process called transduction, which is the first step in visual perception o 2 main types of photoreceptors: ▪ Rods – specialised for vision in low light Found in periphery of retina, absent in fovea Highly sensitive to low levels of light and are responsible for night vision/scotopic vision Cannot detect colour, only monochromatic information Approx 120 million rods in retina When rods active: in dim lighting conditions ▪ Cones – colour vision and sharp acuity Mostly in fovea (central retina), densely packed Sharp, detailed and colour vision -> photopic vision High visual acuity and colour discrimination Approx 6 million cones in retina Types of cones o Blue cones -> sensitive to short wavelengths (ca 419 nm) o Green cones -> medium wavelengths (531 nm) o Red cones -> long wavelengths (559 nm) - How do photoreceptors work? o Transduction process: photoreceptors contain photopigments (specialised chemicals in outer segment) o In rods, photopigment is rhodopsin (made of rod opsin and retinal) o When light hits photoreceptor: ▪ Splits photopigment into its components ▪ Triggers cascade of events that hyperpolarises PR membrane ▪ Change reduces release of NT glutamate to bipolar cells o Signaling: PR connect to bipolar cells -> connect to retinal ganglion cells o Ganglion cells send processed signal through optic nerve to brain - Frequency determines hue - Amplitude relates to its brightness - Receptive fields: o Region of space where a stimulus induces change in firing frequency (the region a ganglion cell/neuron sees) o Area of the visual field that a specific ganglion cell or neuron in the visual pathway “sees” and responds to o Light within receptive field -> neuron fires o Light outside -> little or no response o Many receptive fields have centre-surround structure (ON-centre or OFF-centre) -> allows neurons to detect contrast (light spots on dark backgrounds) - Hierarchical processing: small receptive fields in retina -> combine to form larger, more complex receptive fields in the striate cortex (V1) - Transduction: o The lamellae contain photo pigment (molecules consisting of an opsin (protein) and a retinal (lipid from vitamin A) o Photopigments in photoreceptors split when exposed to light -> triggering change in membrane potential o When light hits photopigment, retinal changes its shape -> triggers casc