Psychology Chapter 4.1-4.2 PDF
Document Details
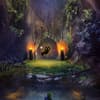
Uploaded by iiScholar
Arizona State University
Tags
Summary
This document is a chapter from a psychology textbook, covering the nervous system. It details the central and peripheral nervous systems, as well as the somatic and autonomic branches. The chapter also explains the functions of neurons and how they communicate.
Full Transcript
**The Nervous System** 4.1.01 The Central Nervous System The **nervous system** is responsible for the regulation and integration of all body systems. Information from inside and outside the body is received and processed by the nervous system, which then coordinates purposeful reactions to this i...
**The Nervous System** 4.1.01 The Central Nervous System The **nervous system** is responsible for the regulation and integration of all body systems. Information from inside and outside the body is received and processed by the nervous system, which then coordinates purposeful reactions to this information. The nervous system can be broadly divided into two major branches, the central and the peripheral nervous systems (Figure 4.1). The **peripheral nervous system** (PNS) comprises the neurons and glia located outside the brain and spinal cord. The **central nervous system** (CNS) consists of the brain and spinal cord and is responsible for the integration of information from the PNS. The PNS relays both sensory information to the CNS and motor commands from the CNS. **Figure 4.1** Divisions of the nervous system. A diagram of a human body Description automatically generated Chapter 4: The Nervous and Endocrine Systems 16 The CNS is composed of white matter and gray matter. **Gray matter** comprises unmyelinated axons, neuronal cell bodies, and dendrites. **White matter** consists primarily of myelinated axons that allow for long-distance communication between neurons. White matter can be described as afferent (meaning toward) or efferent (meaning away from). In the context of the sensory and motor systems, **afferent** neurons send signals toward the brain, and **efferent** neurons carry messages away from the brain. Specifically, afferent sensory neurons of the PNS relay sensory information from the body (eg, sensation from the skin) to afferent (ascending) bundles of axons of the CNS, which carry the information to the brain in the spinal cord. Efferent (descending) bundles of axons in the spinal cord carry motor commands from the brain (eg, impulses to skeletal muscles) to the body. Efferent motor neurons relay these commands to the skeletal muscles. This process is illustrated in Figure 4.2. **Figure 4.2** Sensory and motor neurons. 4.1.02 The Peripheral Nervous System As introduced in Concept 4.1.01, the **peripheral nervous system** (PNS) consists of all neurons located outside the central nervous system (CNS) (ie, the brain and spinal cord). The PNS has two branches: the somatic nervous system and the autonomic nervous system (Figure 4.3).  Chapter 4: The Nervous and Endocrine Systems 17 **Figure 4.3** Divisions of the peripheral nervous system. The **somatic nervous system** includes the sensory neurons that relay information from the body to the CNS and the motor neurons that relay commands from the CNS to the body. Somatic sensory neurons transmit impulses from receptors in the skin, muscles, and joints to the spinal cord. Somatic motor neurons transmit impulses from the spinal cord to the skeletal muscles, allowing individuals to consciously perform specific movements (Figure 4.4). As a result, all movements carried out on a voluntary basis (eg, walking, talking) involve the somatic nervous system. **Figure 4.4** Somatic sensory and motor neurons. In contrast, the **autonomic nervous system** (ANS) controls subconscious, automatic functions that are not subject to voluntary control. Accordingly, the activity of glands, smooth muscles, and cardiac muscles is regulated by the autonomic nervous system\'s visceral motor nerves. For example, the smooth muscles located within the walls of blood vessels can contract or relax to constrict or dilate the vessel. The ANS is further divided into the **sympathetic nervous system**, which helps the body prepare for stressors, and the **parasympathetic nervous system**, which enables the body's return to homeostasis (see Figure 4.5). A diagram of several functions Description automatically generated with medium confidence  The sympathetic (\"fight or flight\") division mobilizes the body for action during stress. For example, oxygen delivery to the skeletal muscles is maximized (eg, increasing heart rate; dilating airways; constricting blood vessels that supply the visceral organs, glands, and skin). The sympathetic nervous system also interacts with the endocrine system. For example, the adrenal glands are innervated by sympathetic neurons. As part of the stress response, these sympathetic neurons stimulate adrenal cells to secrete norepinephrine and epinephrine, which increase sympathetic responses (eg, blood pressure increases). In contrast, the parasympathetic (\"rest and digest\") division promotes energy conservation and storage when stress is low. Parasympathetic neurons are responsible for lowering the heart rate, decreasing airflow through the lungs, increasing blood flow to the visceral organs, and promoting digestion. These functions are suppressed during times of stress. **Neurons and Neural Communication** 4.2.01 Structure and Function of the Neuron Cells of the nervous system include both neurons and glial cells (also called glia or neuroglia). **Neurons** are responsible for sending chemical or electrical signals to other cells, and **glial cells** provide support functions to neurons and the nervous system. A neuron\'s **soma** (cell body) houses the nucleus. The nucleus contains the cell\'s DNA, its genetic material. Everything contained within the cell is enclosed within the neuron\'s membrane. See Figure 4.6 for an illustration of a prototypical neuron. **Figure 4.6** Anatomy of a prototypical neuron. **Dendrites** are branches that extend from the cell body. Dendrites receive input from other neurons when neurotransmitters (chemical messengers) bind to postsynaptic receptors on the dendrites, causing the neuron to respond. In the prototypical neuron, one **axon** then relays the neuron\'s output. The end of the axon at the synapse is the axon terminal (also called the terminal button). Neurons communicate when an **action potential** (AP) (electrical signal) is generated, travels down the axon, and causes the release of neurotransmitters from the axon terminal (depicted in Figure 4.7). A diagram of a nerve cell Description automatically generated Chapter 4: The Nervous and Endocrine Systems 20 **Figure 4.7** A chemical synapse. Most neurons are myelinated, meaning that they have a layer of **myelin** (also called myelin sheath) wrapped around their axons. Myelin is a lipid, multilayered, segmented covering that surrounds and insulates axons. Produced by glia, myelin is nearly continuous along the length of an axon, interrupted only at small, regularly spaced sites called the **nodes of Ranvier**. Myelin greatly [increases the speed](javascript:void(0)) with which the AP travels down the axon. When the AP reaches the axon terminals (also called terminal branches), neurotransmitters are released into the **synapse** (also called the synaptic cleft), a junction formed between the axon terminal of a presynaptic neuron and the dendrites (in most cases) of a postsynaptic neuron. The neurotransmitters can then bind postsynaptic receptors, effecting change in the postsynaptic neuron. Neural communication is aided by glia. Glial cells serve a wide range of functions; some provide structural support and chemical buffering for neurons, produce cerebrospinal fluid, or serve as immune cells in the brain. In addition, some glial cells form myelin sheaths around neurons\' axons. 4.2.02 Neural Communication Communication between neurons involves an electrical signal called an **action potential** (AP) traveling down a neuron\'s axon. The properties of the neuronal membrane and the ion concentrations inside and outside of the cell enable the AP.  Chapter 4: The Nervous and Endocrine Systems 21 The unequal concentration of charged ions between the inside and the outside of all living cells determines the **membrane potential** (voltage difference). The resting membrane potential (RMP) of neurons is due primarily to the high concentration of potassium ions (K+) and the low concentration of sodium ions (Na+) inside neurons compared to outside. When neurotransmitters bind receptors on the dendrites of the postsynaptic neuron, it alters the membrane potential of the cell. Often, when a specific neurotransmitter binds to its receptor, ions can enter or exit the cell. See Figure 4.8 for an illustration of neurotransmitter binding. **Figure 4.8** Neurotransmitter binding postsynaptic receptor. Depending on the type of channel and the environmental conditions (eg, membrane potential) in which the channel is found, ionic movement across the membrane results in either postsynaptic depolarization (excitation) or hyperpolarization (inhibition). When neurotransmitters bind postsynaptic receptors, there is often a change in the electrical potential of the postsynaptic cell. If the change has an excitatory effect, bringing the membrane potential closer to threshold and increasing the likelihood of an AP, it is called an excitatory postsynaptic potential (EPSP). Conversely, an inhibitory postsynaptic potential (IPSP) brings the membrane potential further from threshold and decreases the likelihood of an AP. The postsynaptic neuron receives multiple inputs; the voltage changes in electrical potential from IPSPs and EPSPs are summed. If the summed EPSPs and IPSPs from presynaptic neurons cause depolarization of the postsynaptic neuron that exceeds a certain threshold, an AP is fired (see Figure 4.9). A diagram of a cell membrane Description automatically generated Chapter 4: The Nervous and Endocrine Systems 22 **Figure 4.9** Summation of postsynaptic potentials. The AP is a change in the membrane potential of a neuron and results in the transmission of an electrical signal down its axon. During an AP, the membrane potential of the neuron changes due to the opening and closing of Na+ and K+ ion channels, as follows: At rest, postsynaptic Na+ channels are closed, but if a stimulus causes them to open, Na+ ions enter the cell, causing the membrane to depolarize (ie, the membrane potential becomes more positive). If this depolarization is enough to reach a certain threshold, an AP is initiated in the neuron. If the threshold is reached, additional Na+ channels open, causing further, rapid depolarization of the membrane. Repolarization occurs when Na+ channels inactivate and K+ channels open, allowing K+ to rush out of the cell, and the membrane potential becomes negative again. The RMP is fully restored when K+ and Na+ channels are closed. These steps are shown in Figure 4.10.  Chapter 4: The Nervous and Endocrine Systems 23 **Figure 4.10** Phases of the action potential. Another AP cannot occur during repolarization, the phase during which K+ channels open and Na+ channels inactivate. The **absolute refractory period** refers to this time, during which no new APs can be generated, regardless of the strength of the stimulus received. APs travel down an axon toward the axon terminal as successive regions are depolarized. The voltage-gated Na+ and K+ channels open and close in a sequential manner to propagate the electrical signal down the axon (Figure 4.11). A diagram of a normal phase Description automatically generated Chapter 4: The Nervous and Endocrine Systems 24 **Figure 4.11** Action potential propagation. The rate at which an AP travels down the axon is influenced by axon size, as well as by myelination; larger-diameter axons and axons that are myelinated transmit neural impulses faster than smaller and unmyelinated axons, respectively. At chemical synapses, when an AP reaches the axon terminal of the presynaptic neuron, neurotransmitters are released from the synaptic vesicles (where they are stored) into the synaptic cleft. The neurotransmitters travel across the synaptic cleft, and some bind to receptors on the postsynaptic cell membrane.  Chapter 4: The Nervous and Endocrine Systems 25 In most cases when a neurotransmitter binds to its receptor on the postsynaptic neuron (Figure 4.12), ions can move through the open channel into or out of the postsynaptic neuron, which alters the neuron\'s RMP. The type of receptor and the postsynaptic neuron\'s membrane potential determine whether ionic movement across the membrane results in either postsynaptic excitation (ie, depolarization) or inhibition. **Figure 4.12** Neurotransmitter release at the synapse. Depending on the net effect on the postsynaptic cell, synapses can be classified as excitatory (promoting AP initiation) or inhibitory (inhibiting AP initiation). At excitatory synapses, the membrane potential of the postsynaptic neuron becomes more positive (ie, depolarizes), and if it exceeds threshold, an AP is initiated. In contrast, at inhibitory synapses, neurotransmitters affect the postsynaptic neuron by causing the cell\'s membrane potential to become more negative, inhibiting AP initiation. An excitatory and an inhibitory synapse are contrasted in Figure 4.13. A diagram of a human brain Description automatically generated Chapter 4: The Nervous and Endocrine Systems 26 **Figure 4.13** Example of an excitatory versus an inhibitory synapse. Neurotransmitters themselves can also be classified as excitatory (ie, they make an AP more likely to occur in the postsynaptic neuron), or inhibitory (ie, they make an AP less likely to occur in the postsynaptic neuron). 4.2.03 Neurotransmitters The amino acid neurotransmitters are gamma-aminobutyric acid (GABA), glutamate, and glycine. **GABA** is the principal inhibitory neurotransmitter of the CNS, and **glycine** is also a primarily inhibitory neurotransmitter found in the CNS. **Glutamate** is the primary excitatory neurotransmitter of the CNS. Figure 4.14 shows examples of synapses using glutamate and GABA. **Figure 4.14** Glutamate and GABA at the synapse.  A diagram of a plate with text and images Description automatically generated with medium confidence Chapter 4: The Nervous and Endocrine Systems 27 **Acetylcholine** is the neurotransmitter released by somatic motor neurons at the neuromuscular junction. In addition, acetylcholine plays an important role in the sympathetic and parasympathetic nervous systems. In the brain, acetylcholine is involved in learning and memory, arousal, and sleep-wake cycles. The catecholamines are a category of neurotransmitter that includes dopamine, epinephrine (also called adrenaline), and norepinephrine (also called noradrenaline). **Dopamine** is involved in movement, reward, motivation, and cognition. The dopaminergic system is also implicated in schizophrenia and Parkinson\'s disease and is discussed in Concept 30.1.01 and Lesson 30.2, respectively. **Epinephrine** and **norepinephrine** are involved in autonomic nervous system responses and contribute to wakefulness, alertness, attention, memory, and mood. The monoaminergic neurotransmitters include the catecholamines and serotonin. **Serotonin** is a neurotransmitter involved in sleep/wake regulation, mood, and appetite, among others. **Endorphins** are peptides that act as neurotransmitters. Endorphins are opioids produced by the body that, similar to morphine, modulate pain, as well as contribute to elevated mood following exercise. The neurotransmitter classifications are shown in Table 4.1. **Table 4.1** Primary neurotransmitters. **Neurotransmitter** **Functions** Glutamate (Glu) Primary excitatory neurotransmitter of the central nervous system Involved in learning and memory Gamma-aminobutyric acid (GABA) Primary inhibitory neurotransmitter of the brain Amino acids Glycine (Gly) Primary inhibitory neurotransmitter of the spinal cord Dopamine (DA) Involved in cognition, attention, movement, reward Serotonin (5-HT) Involved in sleep, appetite, mood Epinephrine Involved in sympathetic signaling in the autonomic nervous system Norepinephrine (NE) Involved in sympathetic signaling in the autonomic nervous system Amines Acetylcholine (ACh) Involved in parasympathetic signaling in the autonomic nervous system Released by motor neurons at NMJs of the somatic nervous system to excite skeletal muscle Peptides Endorphins Opioids produced by the body that modulate pain, as well as contribute to elevated mood following exercise ***NMJ** = neuromuscular junction.* Chapter 4: The Nervous and Endocrine Systems 28 Excess neurotransmitters can diffuse out of the cleft, get broken down, or get reabsorbed back into the presynaptic neuron through [reuptake](javascript:void(0)). Some drugs affect these processes, altering neural communication. **Agonists** mimic or enhance the effects of a neurotransmitter (eg, by inhibiting reuptake). **Antagonists** block or reduce the effects of a neurotransmitter (eg, by blocking postsynaptic receptors). Figure 4.15 depicts agonists and antagonists