Field Work (ENV 604) - A Field Report on Hazard Prevalence - PDF
Document Details
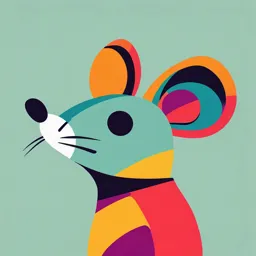
Uploaded by PreEminentJadeite1817
GoldenGate International College
2024
Anima Singh
Tags
Summary
A field report on hazard prevalence and risk appraisal in the Burtibang to Dhorpatan Hunting Reserve region of Nepal, submitted as partial fulfillment for a postgraduate degree in Environmental Science.
Full Transcript
Course Title: Field Work Course No: ENV 604 A FIELD REPORT ON Hazard prevalence and risk appraisal from Burtibang to Dhorpatan Hunting Reserve, Baglung, Nepal. A Field Report Submitted to Department of Envir...
Course Title: Field Work Course No: ENV 604 A FIELD REPORT ON Hazard prevalence and risk appraisal from Burtibang to Dhorpatan Hunting Reserve, Baglung, Nepal. A Field Report Submitted to Department of Environmental Science GoldenGate International College Institute of Science and Technology Tribhuvan University Battisputali, Kathmandu, Nepal In Partial Fulfillment of the Requirements for the Award of Degree of M.Sc. in Environmental Science SUBMITTED BY Anima Singh T.U. Registration No.: 5-3-453-188-2022 T.U. Exam Roll No.: 7919061 November, 2024 TRIBHUVAN UNIVERSITY Institute of Science and Technology GOLDENGATE INTERNATIONAL COLLEGE Battisputali, Kathmandu Date: 2081/07 /29 LETTER OF RECOMMENDATION This is to certify that Ms. Anima Singh has completed the field report titled “A Field Report of Dhorpatan Hunting Reserve, Nepal” as a partial fulfillment of the requirement of M.Sc. Third Semester in Environmental Science. To my knowledge, this report has not been submitted for any other degree anywhere else. I, therefore, recommend the report for acceptance and approval. ………..……………………. Mr. Amrit Sharma (PhD) Field Supervisor Department of Environment Science i TRIBHUVAN UNIVERSITY Institute of Science and Technology GOLDENGATE INTERNATIONAL COLLEGE Battisputali, Kathmandu Date: 2081/07 /29 LETTER OF APPROVAL On the recommendation of the supervisor, Mr. Amrit Sharma (PhD) the field report titled “ A Field Report of Dhorpatan Hunting Reserve, Nepal” submitted by Ms. Anima Singh has been accepted for the examination and submitted to Tribhuvan University in partial fulfillment of the requirements of M.Sc. in Environmental Science. ………..……………………. Mr. Prakash Chandra Aryal Program Coordinator Department of Environment Science Goldengate International College Battisputali, Kathmandu ii ACKNOWLEDGEMENT We want to extend our heartfelt gratitude to our esteemed teachers, Mr. Amrit Sharma (PhDd) and Mr. Sijar Bhatta for their ongoing support, advice, and encouragement during the entire process of developing a report, conducting fieldwork, and finishing the report. We were motivated to complete this assignment effectively by their encouraging feedback, tireless assistance, advice, and helpful suggestions. We appreciate the kind assistance of Mr. Prakash Chandra Aryal, the Program Coordinator for the M.Sc. in Environmental Science. We would especially like to thank every member of our team for their steadfast assistance during the field survey. We thank Dhorpatan Hunting Reserve for permitting us to conduct the research. Lastly, we want to sincerely thank our families for their encouragement and support during our journey. We would like to express our sincere appreciation to the entire M.Sc. Environmental Science Department at Goldengate International College for organizing such a fantastic field trip to the Dhorpatan Hunting Reserve. We are grateful to all of our well-wishers and supporters as well as everyone else who helped with the project and who we may have forgotten to thank. Anima Singh M.Sc. Environmental Science Third Semester iii ABSTRACT This study describes the numerous facets of DRR: field investigations, discharge measurements, boulder size classification, an analysis of structural mitigation practices, landslide study, and the effect of disaster and risk management practices in Dhorpatan and the local community of Burtibang. The data were gathered between October 2024 utilizing a mix of semi-structured questionnaire surveys and field visit observation. Floods and landslides were the two main disasters in the region. Significant variations in discharge rates were observed between upstream and downstream sites, with Bhuju Khola exhibiting the highest flow. These differences highlight the influence of catchment characteristics, rainfall, and terrain on river flow dynamics. The boulder size analysis revealed diverse boulder sizes, ranging from cobbles to boulders, indicating varying geological processes and erosive forces at different sites. Similarly, both Burtibang and Dhorpatan employ structural measures to manage flood risks, including gabion walls, embankments, floodwalls, channelization, and diversion channels. These practices demonstrate a strategic approach to flood mitigation, considering both upstream and downstream impacts. The studied region's was found to be geology, characterized by young sedimentary rocks, particularly mudstone and sandstone, contributing to its susceptibility to landslides. Here, human activities, such as road construction and deforestation, might have significantly altered the region's LULC, increasing the risk of landslides. Thus, to address landslide hazards, a combination of structural and non- structural measures is recommended, including retaining walls, drainage systems, bioengineering techniques, reforestation, and controlled land-use practices. Likewise, through household surveys, the majority of respondents were aware of disasters. Although the areas were thought to be susceptible to flooding, there was no early warning system for sudden floods. The majority of them have used various methods, such as the building of gabion walls when it comes to catastrophe mitigation measures. Other important factors that should be taken into account to minimize the disaster include a proper mitigation plan, urban planning, the employment of bio-engineering technology with structural measures, and public participation. Keywords: DRR, Landslide, vulnerability, flood, survey, susceptibility, mitigation iv Table of Contents LETTER OF RECOMMENDATION i LETTER OF APPROVAL ii ACKNOWLEDGEMENT i ABSTRACT i LIST OF TABLES ii LIST OF FIGURES ii LANDSLIDE HAZARD ASSESSMENT 1 INTRODUCTION 1 1.1 BACKGROUND 1 1.2 OBJECTIVES 2 General Objective: 2 Specific Objectives: 2 1.3 LIMITATION 2 MATERIALS AND METHODOLOGY 3 2.1 STUDY AREA 3 2.2 MATERIALS 3 2.3 METHODS 4 2.4 DATA ANALYSIS 4 RESULTS AND DISCUSSION 5 4.1 Results 5 4.1.1 Landslide Susceptibility Mapping 5 4.1.2 Landslide Distribution and Dimension 5 4.1.3 Geomorphological Characteristics 8 4.1.4 Land Use Land Cover 9 4.1.5 Structural/Bio-engineering measures 9 4.2 DISCUSSION 10 CONCLUSION 11 FLOOD HAZARD ASSESSMENT 12 INTRODUCTION 12 1.1. BACKGROUND 12 1.1.1. Flow velocity and discharge rate 12 v 1.1.2. Boulder size 13 1.1.3. Structural mitigation 13 1.1.4. Flood Hazard Mapping 13 1.2. OBJECTIVES OF THE STUDY 14 General Objective 14 Specific Objectives 14 1.3. LIMITATIONS OF THE STUDY 14 MATERIALS AND METHODOLOGY 15 2.1. STUDY AREA 15 2.2. MATERIALS 16 2.3. METHODS 16 2.3.1 Flow Velocity and Discharge Rate Measurement 16 2.3.2 Boulder size 16 2.3.4 Flood Hazard Mapping 18 2.4 DATA ANALYSIS 18 2.4.1 Flow Velocity and Discharge Rate 18 RESULTS AND DISCUSSION 19 3.1 Result and Discussion 19 3.1.1 Flow Velocity and Discharge Rate Measurement 19 3.1.2 Boulder size classification 19 3.1.3 Structural Mitigation Practices 20 CONCLUSION AND RECOMMENDATIONS 21 4.1 CONCLUSION 21 4.2 RECOMMENDATIONS 21 ANALYSIS OF COMMUNITY'S RISK PERCEPTION AND COPING MEASURES ON DRR THROUGH SURVEY METHOD 22 INTRODUCTION 22 1.1. BACKGROUND 22 1.2. OBJECTIVES OF THE STUDY 23 General Objective 23 Specific Objectives 23 1.3. LIMITATIONS OF THE STUDY 23 2.1. STUDY AREA 24 2.2. MATERIALS 25 vi 2.3 METHODS 25 RESULTS AND DISCUSSION 27 3.1. RESULTS 27 3.1.1 Descriptive Analysis by Demographics 27 3.1.2 Hazard Prevalence and Disaster Trends 29 3.1.3. Disaster Impact and Loss Assessment 30 3.1.4. Community Preparedness, Response, and Mitigation Measures for different types of disasters. 33 3.2 DISCUSSION 33 CONCLUSION AND RECOMMENDATIONS 35 4.1 CONCLUSION 35 REFERENCES 36 ANNEXES 39 PHOTOGRAPHS 41 vi LIST OF TABLES Table 1: Wentworth's (1922) grain size classification 17 Table 2: Records of damage caused by different disasters in different locations 32 Table 3: Community Response and Mitigation measures for different types of disasters 33 Table 4: Upstream and Downstream Location coordinates 39 Table 5: Flow velocity and discharge rate calculation 39 Table 6: Calculation of Boulder size and volume 40 Table 7: Sites along with the average length of boulders measured 40 LIST OF FIGURES Figure 1: Map of the study area: Burtibang, Baglung, Nepal. 3 Figure 2: Landslide susceptibility mapping 5 Figure 3: Landslide 1 6 Figure 4: Landslide 2 7 Figure 5: Landslide 3 8 Figure 6: Study sites for flood measurement 15 Figure 7: Map showing the area of household survey sites 25 Figure 8: Methodological framework of the study 26 Figure 9: Total number of respondents in different locations 27 Figure 10: Gender distribution of total number of respondents 28 Figure 11: Age group of total number of respondents in different sites 28 Figure 12: Number of respondents according to their social status 29 Figure 13: Disaster ranking by respondents across different sites 30 Figure 14: Human Loss due to different types of disasters. 31 Figure 15: Group photo downstream at Burtibang 41 Figure 16: Group photo upstream at Dhorpatan 41 Figure 17: Measurement of Boulder volume 41 Figure 18: Landslide viewing 41 Figure 19: Survey with local community members 42 Figure 20: Flow measurement using Current meter 42 ACRONYMS DRM Disaster Risk Management DRR Disaster Risk Reduction LULC Land Use Land Cover GIS Geographic Information Systems viii LANDSLIDE HAZARD ASSESSMENT INTRODUCTION 1.1 BACKGROUND One significant geological hazard that harms the social and natural environments is landslides. Many authors handle the idea of a landslide in different ways. Varnes (1984) "Almost all varieties of mass movements on a slope, including some like rock, falls, topples, and debris flow that involves little or no true sliding," is how landslides are classified. According to Brusden (1984), landslides are a special kind of mass transit that doesn't require a conveyance medium like water, air, or ice. Although landslides are a natural occurrence, they can become dangerous and result in fatalities as well as the destruction of both natural and man-made structures. There are numerous definitions of landslide hazard, but some of the more significant ones are provided by Burton et al. (1978). An essential phase in landslide risk management and inquiry is landslide hazard zonation. (Holec et al., 2013) assert that if the issue is identified before the landslide event, at least 90% of landslide losses can be prevented. The process of dividing the land surface into areas and rating these areas based on the level of existing or anticipated hazards from landslides or other mass movements is characterized as "zonation." (Varnes, 1984). To mitigate the impacts of landslides, it is essential to understand the factors that influence people’s ability to respond to future events effectively. A review of the literature indicates that the following four issues are crucial to this study. Hazard assessment Hazard knowledge Risk perception Disaster preparedness Understanding how residents of high-risk areas view risks and hazards, as well as their awareness and readiness for specific risks, is crucial to reducing the effects of natural catastrophes (Burton, 1993). Risk perception and hazard understanding are crucial elements of risk reduction strategies. The rationale is that with an understanding of the risk and hazard, a person is likely to be encouraged to participate in mitigation efforts for natural calamities. A thorough grasp of the institutional, cultural, economic, social, and physical aspects of landslide vulnerability must be carefully evaluated to implement successful risk reduction initiatives (Alexander, 2018). 1 1.2 OBJECTIVES General Objective: To assess the landslide hazards in the Burtibang region of Baglung, Nepal, and propose mitigation strategies. Specific Objectives: Identify and map landslides in the study area. Analyze the characteristics of each landslide (type, size, location). Investigate the relationship between landslides and various factors (geology, slope, rainfall, land use). Evaluate the effectiveness of existing mitigation measures (gabion walls, vegetation planting). 1.3 LIMITATION Data Availability for Landslide Susceptibility Mapping Geographical and Access Constraints Since the field survey was conducted at a specific time, the study did not capture seasonal variations or events, such as monsoon-triggered landslides, which could affect the analysis of landslide frequency and hazard assessment. 2 MATERIALS AND METHODOLOGY 2.1 STUDY AREA Burtibang is a study area for landslide hazard assessments. Burtibang lies in between 28.3319° N, 83.1602° E. It is a village development committee in Baglung district in the Dhaulagiri zone of central Nepal. Burtibang is the center of Dhorpatan municipality in the western part of Baglung District in Gandaki province. Dhorpatan municipality with 9 wards has been formed by merging Burtibang, Khunga, Adhikarichaur, and Bowang VDCs. Along with the confluence of Nisi, Bhuji, and Taman Rivers, the Badigad River is also a source of Burtibang. Figure 1: Map of the study area: Burtibang, Baglung, Nepal. 2.2 MATERIALS i) Brunton compass ii) Global Positioning System (GPS). 3 2.3 METHODS A detailed field survey was conducted to identify the active and old landslide sites. Then, the location, type, and size of each landslide were recorded by using GPS. Also, the visible signs of cracks, displaced trees, vegetation types, infrastructure, or soil movement were documented. Similarly, the slope angle of each landslide was measured by using a Brunton compass. The slope, curvature, elevation, length, and breadth were also evaluated and noted. Then, for creating the distribution map, the data of distance from roads, distance from streams, topography, geology, land use, and so on were gathered into GIS. Here, the historic landslide data were obtained from Google Earth images along with the observed landslide sites for mapping. 2.4 DATA ANALYSIS The field data were systematically organized, analyzed, and interpreted to achieve the study's objectives, incorporating a range of tools and methodologies. Microsoft Word and Excel were employed for documentation and data management, while ArcGIS was utilized for spatial analysis. Data collection involved detailed documentation of topographic maps, geological surveys, soil samples, and rock samples. Using Geographic Information System (GIS) techniques, spatial analysis was conducted to identify high-risk areas by overlaying various data layers, including slope, land use, and precipitation patterns. Statistical analysis quantified relationships between landslide occurrences and potential triggering factors, such as rainfall intensity and soil moisture content. These findings were then integrated to create hazard maps that categorized regions by risk levels, informed by slope stability and historical data. 4 RESULTS AND DISCUSSION 4.1 Results 4.1.1 Landslide Susceptibility Mapping The map of Figure 2 classifies different zones within the study area based on their susceptibility to landslides: very low, low, moderate, high, and very high. Notably, a significant portion of the study area is classified as high to very high susceptibility. These zones might be characterized by steep slopes, loose soil, and proximity to drainage channels, which are known predisposing factors for landslides. Figure 2: Landslide susceptibility mapping 4.1.2 Landslide Distribution and Dimension Three distinct landslides were identified in the Burtibang region, each exhibiting unique characteristics and posing varying levels of risk. These landslides are primarily located near hilltops, a position inherently prone to slope instability due to gravity and the geological makeup of the area. Landslide number 1: Location: The study site was located about 200 meters downstream of MisiKhola and opposite a powerhouse, considered a natural landslide. Description: It is prone to reactivation or further movement under certain conditions, such as heavy rainfall or seismic activity. Debris flow, with loose earth and smaller rocks, carried downward by gravity, and possibly water flow was seen. 5 Characteristics: It is a deep-seated, concave-shaped formation with loose soil. Its concave structure was coupled with the loose soil, making it particularly susceptible to gravitational pull and water infiltration, increasing its likelihood of reactivation during intense rainfall. Dimension: Covering an area of 26,000 square meters, and lying on a slope angle of 30 degrees, this landslide has significant dimensions and poses a considerable risk to the surrounding environment and infrastructure. Causes: Likely triggered by natural slope instability and soil composition in the area. Figure 3: Landslide 1 Landslide number 2: Description: The second landslide, however, is classified as a complex, quasi-natural landslide. It is prone to reactivation or further movement under certain conditions, such as heavy rainfall or seismic activity. Characteristics: Debris flow, with loose earth and smaller rocks, carried downward by gravity, and possibly water flow was seen. The shallower nature of this landslide suggests that it may be more influenced by surface runoff and erosion, making it susceptible to gradual downslope movement, especially during monsoon periods. Dimension: It is a complex formation that features a concave, shallow-sheeted profile. A gabion wall, measuring approximately 2.3 meters in height, has been constructed as a stabilization effort, though its effectiveness may be limited given the extensive length and breadth of the landslide—180 meters and 151 meters, respectively. This landslide spans an area of approximately 27,180 square meters, indicating a broad impact zone. 6 Causes: Its formation may involve both natural processes and human influence, indicating that while environmental conditions contribute, human activity—such as road construction—might have played a role in destabilizing the slope. Figure 4: Landslide 2 Landslide number 3: Location: The third landslide, situated across Turture Khola is the largest in the area and is classified as an active landslide. Description: The constant instability, posing a more persistent threat to nearby infrastructure was observed at the site. Large boulders were observed that were indicative of a strong and forceful movement that dislodged significant portions of the rock. Characteristics: The presence of large boulders suggests that the slope’s underlying geology includes resistant rock layers that fractured and detached during movement, adding to the destructive potential of the landslide. The observed convex configuration may also contribute to further slope instability by creating high-stress zones along the hillside. Dimension: It is also deep-seated but exhibits a convex shape, indicating a bulging slope surface. It is marked by enormous boulders, some larger than the size of a house, indicating a significant force behind the slide and substantial risk to structures and lives in its path. Causes: Implying ongoing slope movement that continues to affect the region. Together, these landslides underscore the region's susceptibility to varying types of slope failures. The differences in their shapes, soil compositions, and stabilization requirements 7 illustrate the complex interplay of geological and environmental factors affecting slope stability in this mountainous area. Further assessment and stabilization measures are crucial to managing the ongoing and potential hazards these landslides pose to local communities and infrastructure. Figure 5: Landslide 3 4.1.3 Geomorphological Characteristics The Burtibang region is composed of relatively young and unstable sedimentary rocks, including mudstone, siltstone, sandstone, and shale, which significantly increase its susceptibility to landslides. Mudstone, with its banded structure, easily absorbs water and becomes unstable during heavy rainfall, often creating overhanging sandstone beds above it. These jointed and fractured sandstone beds, when undermined by softened mudstone, quickly break into blocks, leading to sudden landslides. Shale and siltstone add to this instability due to their brittleness, which generates loose debris that flows down with water. As noted by (Dahal R. K., 2012), the region’s jointed sandstone and layered mudstone create an environment prone to frequent and severe landslides, especially during monsoon rains. This geological composition, combined with steep slopes and intense rainfall, poses significant risks to the local communities, highlighting the need for effective slope management and mitigation. 8 This region is composed of geologically very young sedimentary rocks such as mudstone, siltstone, sandstone, and shale. Mudstones with alternating bands can flow when saturated with water, creating overhanging sandstone beds. Such overhang, jointed sandstone beds quickly break up into blocks (Dahal R. K., 2012) which results in being highly susceptible to landslide processes during rainfall. 4.1.4 Land Use Land Cover The Land Use Land Cover (LULC) analysis of the Burtibang region, classified according to the Nepal LULC 2021 system, reveals a complex interaction between land cover types and landslide occurrences. While the landslides identified in the study area were situated away from existing built-up areas, the influence of road construction and unmanaged planning on LULC emerged as a critical factor in understanding the region's landslide hazard. Road construction, particularly in the hilly terrain of Burtibang, has inevitably altered the LULC. This shift in land cover has significant implications for slope stability. The removal of trees and vegetation during road construction diminishes the soil's natural reinforcement, leading to increased erosion and susceptibility to landslides. Furthermore, the disruption of natural drainage patterns due to road cuts and embankments can exacerbate slope instability. 4.1.5 Structural/Bio-engineering measures In the second landslide area, bio-engineering practices were implemented to help stabilize the slope, which had been compromised due to road construction activities. A gabion wall was constructed as an initial step to control erosion by acting as a physical barrier. This wall effectively reduces surface erosion by shielding the slope from the impact of flowing water, particularly during intense rainfall, which often exacerbates landslide risk in such unstable regions. Alongside the gabion wall, bioengineering techniques were employed, including the planting of grass, shrubs, and trees. These vegetation types play a critical role in binding soil particles, enhancing slope stability, and reducing surface runoff. Tree roots, for instance, penetrate deeper into the soil, providing additional stability, while grasses and shrubs offer a dense cover that mitigates erosion. These measures, combined with small-scale civil engineering structures, are tailored to address shallow-seated instability by reinforcing the slope naturally and allowing it to withstand seasonal rainfall more effectively. According to Howell and Deoja et al. (1991), the success of bio-engineering techniques in managing landslide-prone areas largely depends on understanding the specific failure characteristics of the slope, and here, the combination of vegetation and structural barriers was chosen for its suitability to the region's instability challenges. 9 4.2 DISCUSSION Debris flow which was seen on the first and second landslide is a form of rapid mass movement in which the combination of loose soil, rock, organic matter, air, and water mobilize as a slurry that flows downslope. It is caused by the heavy precipitation that erodes and mobilizes loose soil and rock on steep slopes. Landslides and debris flows are most frequent within the region of active tectonism with distinctive geological structures and differential lithostratigraphy. The lesser Himalayan region in the Himalayan arc has suffered from multiple events of mass movements because of fragile geology and high-intensity rainfall(Dahal & Dahal, 2017).The region's geological characteristics, with young sedimentary rocks like mudstone and sandstone, significantly influence landslide behavior, making the area highly susceptible to slope failures. These weak, weather-prone rocks are easily destabilized by heavy rainfall and seismic activity. Mudstone, which readily absorbs water, loses cohesion and contributes to the debris flows seen in the first and second landslides, where loose soil, pebbles, and cobbles slide downhill. Sandstone, although more resistant, can still fracture under stress, as evidenced by the large boulders in the third landslide, where deeper-seated, more forceful movements occur. The concave profiles of the first and second landslides indicate zones vulnerable to water infiltration and gravitational pull, while the convex shape of the third landslide suggests high-stress zones that lead to rock failure. The third landslide, in particular, poses a greater risk as it occupies a large area and contains massive boulders, some as large as houses, that threaten road sections and require vehicles to navigate carefully. In contrast, the first and second landslides have smaller impacts and involve primarily loose soil and smaller rocks. Furthermore, the landslide- prone nature of the region is exacerbated by anthropogenic factors, including unplanned construction and deforestation, which disrupt natural drainage and increase slope instability. Altogether, the interplay of geological fragility and environmental pressures creates a high-risk environment for landslides, posing serious threats to communities and infrastructure. According to(Poudel & Regmi, 2016) The Slope factor is the most significant in the occurrence of landslides as it affects the soil water content (surface and subsurface), formation of soil, erosion potential, and so on Land use and land cover (LULC) changes, especially through road construction and deforestation, have a direct impact on slope instability and landslide susceptibility. Road construction on steep or fragile slopes often involves cutting into hillsides, which disturbs the natural soil and rock layers, reduces slope support, and alters drainage patterns. These changes increase the likelihood of slope failure, particularly in areas with loose soil or weak geological formations. The lack of vegetation along roadsides further contributes to instability, as 10 vegetation roots play a crucial role in binding the soil and absorbing water. Without these roots, slopes become more prone to erosion and rapid water infiltration, which can trigger landslides, especially during intense rainfall. Similarly, deforestation removes essential tree cover that stabilizes slopes and controls soil moisture levels. When forests are cleared, the soil becomes exposed, and its ability to resist gravitational pull is weakened, creating conditions ripe for landslides. Together, unregulated road building and deforestation disrupt the delicate balance of mountainous terrain, accelerating erosion and undermining slope stability, making landslides more frequent and severe in these altered landscapes. To mitigate the landslide risks identified in the region, a range of structural and non-structural measures can be implemented. Key slope stabilization techniques include constructing retaining walls, such as gabion and reinforced concrete walls, to support unstable slopes and reduce erosion, particularly near infrastructure and road sections threatened by large landslides. Installing drainage systems to divert surface runoff away from landslide-prone areas can help reduce water infiltration into the soil, which is especially effective for areas with high rainfall. Bioengineering solutions, such as terracing and the use of erosion-resistant vegetation, can further stabilize slopes by enhancing soil cohesion and reducing surface erosion. Reforestation is another essential measure, as it helps restore natural vegetation that stabilizes the soil with deep-rooted plants, reduces surface runoff, and minimizes soil loss. Implementing controlled land-use practices, including regulated construction and restrictions on deforestation, would also help maintain the stability of natural slopes. Moreover, early warning systems, particularly in regions with high rainfall or tectonic activity, can alert local communities to potential landslide events. Together, these mitigation efforts can significantly reduce the vulnerability of communities and infrastructure to landslides, while contributing to long-term slope stability in the fragile Himalayan region. CONCLUSION The study on landslide hazards in the Burtibang region of Baglung, Nepal, highlights the significant geological and human factors contributing to slope instability. The area’s young sedimentary rocks, particularly mudstone and sandstone, increase vulnerability during monsoon rains. Three types of landslides were identified, each posing risks to infrastructure and safety. The research emphasizes the need for effective hazard zonation and community awareness to improve disaster preparedness. Additionally, bioengineering techniques like gabion walls and vegetation planting have shown potential in stabilizing slopes. Overall, ongoing monitoring and proactive management strategies are essential for mitigating landslide risks in this vulnerable region. 11 FLOOD HAZARD ASSESSMENT INTRODUCTION 1.1. BACKGROUND A disaster is a serious problem occurring over a short or long period that causes widespread human, material, economic, or environmental loss that exceeds the ability of the affected community or society to cope using its resources. Nepal is at risk of disasters triggered by natural hazards including earthquakes, floods, landslides, glacial lake outburst floods, and droughts. The country geologically lies across the boundary between India and southern Tibet which are still moving towards each other by 2 meters per century. Natural disasters occur and affect people’s lives and livelihoods in almost all parts of the world. Some populations are more vulnerable than others and disparity exists between nations and communities within a country. Furthermore, within communities’ different households may be affected differently, and even within households, the vulnerability of individual household members may vary. Disasters are the product of a combination of hazards and vulnerability (Yan Du et al., 2015). Flood hazards are natural events that occur when water levels in rivers, lakes, or oceans exceed their normal capacity, inundating surrounding areas. Nepal is vulnerable to flood threats and calamities due to a combination of high terrain, steep mountain topography, deep and narrow river basins with frequent mass-wasting occurrences, and highly concentrated monsoon precipitation (Khanal et al., 2007) that can have devastating consequences, including loss of life, property damage, and disruption of infrastructure. Floods are complex natural phenomena influenced by various factors. Thus, understanding flow velocity and discharge rate, boulder size on the bank of the river, applied structural mitigation-like factors and their interactions is crucial for effective flood risk management. 1.1.1. Flow velocity and discharge rate Flow velocity and discharge rate are two critical parameters in understanding and managing flood hazards that provide valuable insights into the magnitude, timing, and potential impacts of flood events. Flow velocity refers to the speed at which water moves through a river channel which is measured in meters per second (m/s). Increased flow rates can make floodwaters more erosive, causing more harm to property, infrastructure, and the environment. High velocity can also hasten the transport of silt, which can choke river channels and raise the danger of flooding (Othman et al., 2023). 12 Similarly, discharge rate represents the volume of water flowing through a river channel per unit time that is measured in cubic meters per second (m³/s). A greater amount of water passing through the river is indicated by a higher discharge rate, which might raise the probability and intensity of floods. To predict future flood events, it is crucial to keep a record of discharge rates during times of intense precipitation or snowmelt. 1.1.2. Boulder size The classification of boulder sizes along the riverbank is essential for understanding their role in erosion and sediment transport during flood events. This will contribute to developing effective erosion control measures and assessing potential impacts on river morphology. Some of the importance of boulder size classification are listed below: Boulders act as natural barriers, reducing the erosive power of floodwaters and protecting riverbanks from collapse. The presence of boulders can influence sediment transport patterns, affecting the formation of sandbars and other river features that can impact flood risk. Its data is essential for designing and implementing effective erosion control measures, such as boulder placement, gabion walls, or channel armoring. 1.1.3. Structural mitigation Structural mitigation refers to the physical or engineering measures taken to reduce the impact of natural disasters and other hazards. These measures safeguard lives, properties, and infrastructure by enhancing their resilience against threats like earthquakes, floods, landslides, and hurricanes. Structural mitigation includes constructing dams and levees to control floods, building earthquake-resistant structures, retrofitting old buildings to meet modern safety standards, and establishing sea walls to protect coastal areas from storm surges. These interventions often require significant financial investment and technical expertise, but they play a crucial role in minimizing disaster damage and ensuring long-term safety. By focusing on the strength and resilience of physical structures, structural mitigation helps communities withstand and recover more quickly from adverse events, reducing the need for extensive emergency responses and recovery efforts. 1.1.4. Flood Hazard Mapping Flood Hazard Mapping is the process of identifying and delineating areas that are prone to flooding based on historical data, hydrological and hydraulic modeling, and geographical factors. The goal is to assess the spatial extent of potential floods, their frequency, and severity to aid in flood risk management, land-use planning, and disaster preparedness. 13 Among various non-structural measures needed for disaster mitigation, hazard mapping is one of the important nonstructural measures (Mahato, et. al., 1996). Land flood hazard areas can be delineated based on hydrologic studies for selected flood peak magnitudes and topographic information (Joshi, 1987). Some of the applications of Flood Hazard Mapping are listed below: Urban Planning and Development: Guides urban expansion, land-use zoning, and infrastructure planning to avoid flood-prone areas. Disaster Risk Reduction and Mitigation: This helps in planning structural and non- structural flood mitigation measures, such as levees, flood walls, retention basins, or green infrastructure. Emergency Response and Evacuation Planning: Provides crucial information for developing flood warning systems, evacuation routes, and relief centers. Insurance and Risk Assessment: Assists insurance companies in assessing risks and setting appropriate premiums for flood-prone areas. 1.2. OBJECTIVES OF THE STUDY General Objective To assess the flood hazard from Burtibang to Dhorpatan Hunting Reserve, Baglung, Nepal. Specific Objectives To measure flow velocity and calculate the flood discharge rate of rivers upstream and downstream using the float method and current meter To classify the boulder size along the riverbank To study the structural mitigation practices to reduce flood risk To prepare flood hazard mapping 1.3. LIMITATIONS OF THE STUDY The study was carried out during high rainfall and Elevated River flows thus rainfall and high water levels can make it difficult to accurately measure the flow velocity and discharge rate. 14 MATERIALS AND METHODOLOGY 2.1. STUDY AREA The study was conducted in Baglung district located in the hilly region of Nepal. Baglung district lies in the Gandaki Province, 272 km from Kathmandu, and has four municipalities and six rural municipalities with a total area of 1784 square kilometers (Panta,2021). It has a latitude of 28°20′47.04″ North and a longitude of 83°14′43.80″ East. The study was conducted downstream at Burtibang, midstream at Bobang, and upstream at Dhorpatan, each representing distinct hydrological and geomorphological characteristics as shown in figure 1. Burtibang is a rural municipality in Baglung District and the center of Dhorpatan municipality in the western part of Baglung District. Bobang, a transitional zone between the downstream and upstream areas, is characterized by a mix of agricultural land, forests, and settlements. Dhorpatan, a municipality in Nepal's Baglung District is a remote region at 3,900 meters elevation. Figure 6: Study sites for flood measurement 15 2.2. MATERIALS I. Measuring tape II. GPS device III. Bottle IV. Current meter V. Thread VI. Stopwatch 2.3. METHODS 2.3.1 Flow Velocity and Discharge Rate Measurement The data was collected by employing two methods which include; i. Floating method To measure the flow velocity of the river, the floating method was used with the use of bottles. A straight section of the river approximately 15 meters in length was selected, with a starting and ending point marking over a distance. An empty plastic bottle was used as a float, and it transmitted time between two marked points was recorded using a stopwatch. The flow velocity was then calculated by dividing the distance by the recorded time. This process was repeated multiple times at different locations along the river to obtain an average flow velocity. ii. Current meter To measure the flow velocity of the river using a current meter, a straight section of the river was selected, ideally where the depth and flow were uniform. The current meter was set up by lowering it into the water at a specified depth, positioning it perpendicular to the flow direction. The device was activated to start recording the number of revolutions or pulses, which correspond to the water's velocity. The data were recorded over a set period, typically 40 seconds to a minute. This reading was converted into flow velocity using the meter’s calibration formula. For accuracy, measurements were repeated at different points across the river's cross- section, and an average was calculated to estimate the overall flow velocity. 2.3.2 Boulder size A boulder-shaped grain and sediments were collected using a 1x1 feet quadrant to represent the sediment characteristics of the study site. Boulders were visually classified into size categories based on their approximate dimensions. For more precise measurements, the length, width, and height of boulders were measured using a measuring tape. The collected data on boulder size and distribution were analyzed to classify boulders into different size categories. This classification was based on visual observation and, in some cases, direct measurements which were then classified based on Wentworth's (1922) grain size 16 classification. The data was used to assess the potential impact of boulders on river morphology and flood dynamics. Table 1: Wentworth's (1922) grain size classification Size Class Diameter Range Boulder > 256 mm Cobble 64 mm – 256 mm Pebble 4 mm – 64 mm Granule 2 mm – 4 mm Sand 0.0625 mm – 2 mm Silt 0.0039 mm – 0.0625 mm Clay < 0.0039 mm 2.3.3 Structural Measurements To assess the structural integrity of the gabion wall, the following parameters were measured: i) Geometric properties: Shape: The overall configuration of the gabion structure (such as straight, curved, or stepped). Size: The dimensions of the gabion units, including length, height, and width. Depth: The depth of the gabion wall, considering both deep-seated and shallow-seated sections. i) Sediment load assessment (Bed load and suspended load) To understand the sediment load and its impact on the gabion wall, the bed load and suspended load observations were made: The assessment of bed load involved observing the transport of larger sediment particles along the riverbed during flood events. And, the assessment of suspended load involved evaluating the transport of finer sediment particles carried within the water column. This involved: Using standardized dimension measurement to assess the dimensions of the gabion units. Recording the characteristics of the sediment samples obtained from the quadrat, emphasizing grain size distribution. 17 Then, the measurements of gathered data were plotted to visualize the relationship between the flood wall dimensions and the associated sediment characteristics. This graphical representation facilitated the evaluation of gabion wall effectiveness under different flood scenarios. 2.3.4 Flood Hazard Mapping The collected data, including topographic maps, hydrological data, and field observations, was used to create flood hazard maps. Geographic Information Systems (GIS) software was used to integrate and analyze the spatial data. 2.4 DATA ANALYSIS 2.4.1 Flow Velocity and Discharge Rate The collected data on flow velocity and cross-sectional area were used to calculate the discharge rate of the river using the following formula: The data was analyzed by using the formula: Discharge (Q) = Area (A) × Velocity (V) Velocity= Distance/ Time (m/s) Cross-section area = length x breath Where: Q is the discharge rate in cubic meters per second (m³/s) A is the cross-sectional area of the river in square meters (m²) V is the flow velocity in meters per second (m/s) The flow velocity was calculated using the float method and current meter measurements. The cross-sectional area was determined by measuring the width and depth of the river at various points along the study reach. 18 RESULTS AND DISCUSSION 3.1 Result and Discussion 3.1.1 Flow Velocity and Discharge Rate Measurement The downstream sites exhibited varying discharge rates: Bhuju Khola 24.22 m³/s, Taman Khola 21.29 m³/s, and Bobang River 8.04 m³/s. Among the three downstream sites, Bhuju Khola was found with the highest discharge rate. According to Shrestha & Bajracharaya(2015), Bhuju Khola had the most substantial flow, likely influenced by factors such as a larger catchment area, rainfall, or terrain, contributing to higher runoff and flow velocity. Similarly, the significantly lower discharge at Bobang River suggests a smaller contributing watershed or reduced flow velocity, impacting its overall discharge capacity (Poudel et al., 2018), which may be attributed to factors like channel morphology or vegetation cover. Likewise, the upstream site, Uttarganga River in Dhorpatan, recorded a discharge rate of 9.31 m³/s. This value, while significant, is lower than the peak discharge observed at Bhuju Khola. This difference highlights the influence of downstream tributaries and catchment characteristics, precipitation, and seasonal variations on overall river flow. Flow velocity is crucial for understanding river discharge which directly affects water resource management, sediment transport, and ecosystem health (Shrestha et al., 2014). This measurement provides insights into the river's hydrological regime, contributing to flood forecasting and irrigation planning in the region (Ghimire & Shakya, 2019). Accurate discharge data are essential for sustainable river basin management, particularly in Nepal’s mountainous regions, where rivers are prone to seasonal fluctuations. Therefore, the discharge measurements in the Dhorpatan region show a clear difference between the downstream and upstream flow dynamics. Downstream, Bhuju Khola recorded the highest discharge at 24.22 m³/s, surpassing the 9.31 m³/s discharge measured upstream at the Uttarganga River. 3.1.2 Boulder size classification Based on measurements from three different sites, the boulder sizes were recorded as follows: Site 1 with 180 mm, Site 2 with 260 mm, and Site 3 with 180 mm. According to Wentworth (1922), grain size classification consists of sediment sizes generally divided into gravel, sand, silt, and mud categories, with additional classifications based on size. Using this classification, the particles at Site 1 and Site 3 were identified as "Cobble," as their measured size of 180 mm fits within the 64-256 mm range. In contrast, the particle size at Site 2 was measured at 260 mm, which falls into the "Boulder" category as it exceeds the 256 mm threshold. Overall, the analysis highlights the sediment distribution and particle size variations, showing that Sites 1 19 and 3 contain Cobble-sized particles, while Site 2 contains Boulder-sized particles. The observed variation in boulder sizes across the three sites suggests differences in the geological processes that have shaped these areas. Site 2, with its larger boulders, may have experienced more intense erosive forces or the presence of larger source rocks. Sites 1 and 3, with their smaller cobble-sized particles, might have been influenced by less intense processes. Potential geological factors contributing to these variations include fluvial processes (river and stream erosion and deposition), mass wasting events (landslides and rockfalls), the inherent properties of rock types and their susceptibility to weathering, and anthropogenic influences such as mining, quarrying, and construction. 3.1.3 Structural Mitigation Practices In Burtibang and Dhorpatan, a combination of structural mitigation practices has been implemented to manage the flood risks posed by their river systems, with tailored approaches suited to the specific needs of each area’s geography and flood exposure. In the downstream area of Burtibang, a more defensive strategy is in place, featuring gabion walls, embankments, and floodwalls. These structures protect Burtibang's settlements and agricultural land from overflow and erosion by reinforcing riverbanks and creating physical barriers. Gabion walls offer a flexible and permeable solution to erosion, allowing water to flow through while keeping soil in place. Embankments and floodwalls heighten the river's edges, effectively reducing the likelihood of water spilling over into vulnerable areas during peak flows. Additionally, reservoirs and dams upstream contribute to water regulation, storing excess rainfall and gradually releasing it, which helps prevent sudden surges in downstream water levels. This layered strategy in Burtibang significantly reduces direct flood impacts on its communities and infrastructure. In contrast, the upstream region of Dhorpatan employs structural measures that focus on managing and redirecting water flow to mitigate potential flooding impacts on downstream areas like Burtibang. Here, channelization and diversion channels have been constructed to modify and direct the river’s natural course away from populated or critical zones. These measures help manage the river’s speed and volume, particularly during the monsoon season, lowering the risk of flash floods that could affect downstream communities. Dhorpatan's use of gabion wall channels serves a dual purpose: reinforcing the banks along modified channels and preventing erosion while maintaining the channels’ integrity for controlled water flow. The comparative approach in both regions highlights a strategic integration of upstream and downstream flood mitigation efforts. Dhorpatan’s interventions focus on preemptive measures to direct and control river flow, creating a more manageable volume of water downstream. 20 Burtibang, on the other hand, relies on barriers and reservoirs to directly protect against and mitigate flood impacts. Together, these structural practices create a resilient system, with upstream flow management easing the pressure on downstream defenses, effectively protecting Burtibang’s communities and infrastructure from seasonal flooding threats. CONCLUSION AND RECOMMENDATIONS 4.1 CONCLUSION This study in Burtibang, Baglung district, assessed flood hazards by examining flow velocity, discharge rates, boulder sizes, and structural mitigation practices. The findings revealed significant variations in flow velocities, discharge rates, and boulder sizes across different sites within the study area. These variations are attributed to factors such as catchment area, topography, rainfall patterns, and human interventions. The implementation of structural mitigation measures, including gabion walls, embankments, and floodwalls, has been effective in reducing flood risks in Burtibang. However, continuous monitoring and maintenance of these structures are crucial to ensure their long-term effectiveness. 4.2 RECOMMENDATIONS A comprehensive flood risk assessment should be conducted considering climate change, land use, and population growth to identify vulnerable areas Early warning systems should be placed to alert communities in advance of potential floods Sustainable land use practices, such as reforestation, to reduce soil erosion and mitigate flood risks along with traditional knowledge and practices should be promoted and incorporated Regular monitoring program for structural mitigation measures and conduct necessary repairs should be established Further research should be done to improve predictions and mitigation measures. 21 ANALYSIS OF COMMUNITY'S RISK PERCEPTION AND COPING MEASURES ON DRR THROUGH SURVEY METHOD INTRODUCTION 1.1. BACKGROUND Disasters, both natural and anthropogenic, pose significant threats to communities worldwide, leading to loss of life, property damage, and disruption of social and economic systems (Feng & Cui, 2021). Disaster and risk management is a critical field focused on minimizing the impact of disasters on communities and individuals (Kayona et al., 2023). It has become an essential field, particularly in regions vulnerable to natural and human-made hazards. Effective DRM involves several critical components, including risk assessment, early warning systems, public awareness and education, infrastructure planning, and preparedness (Asih et al., 2023). The goal is to enhance resilience by developing systems that can effectively manage risks before, during, and after a disaster event (Donahue et al., 2023). Understanding the vulnerability and capacity of communities is fundamental to effective disaster risk management (DRM) (Jamshed et al., 2019). Vulnerability refers to the susceptibility of a community to the impacts of hazards, influenced by various factors such as socioeconomic status, infrastructure quality, and environmental conditions (Cutter et al., 2003). Conversely, community capacity encompasses the strengths and resources available to mitigate risks and respond effectively to disasters. Communities with high vulnerability often face greater challenges during disasters, leading to increased loss of life and property. Factors contributing to vulnerability include poverty, lack of access to information, inadequate infrastructure, and social inequalities. For instance, research indicates that marginalized groups are disproportionately affected by disasters due to their limited resources and access to services (Flanagan et al., 2011). The perception of risk within a community is crucial for understanding how residents respond to potential hazards (Dhar et al., 2023). Analyzing community risk perception and coping measures provides insights into their vulnerabilities and the strategies they employ to mitigate risks (Khadka et al., 2024). This understanding is essential for developing targeted interventions that enhance resilience against future disasters. Loss and damage refer to the adverse impacts resulting from disasters, encompassing both tangible and intangible consequences experienced by affected communities. These impacts can manifest in various forms, including loss of life, injury, property destruction, and disruption of essential services, leading to significant economic and social repercussions. Effective assessment of loss and damage is crucial for understanding the full impact of a disaster. This involves collecting data 22 on fatalities, injuries, property damage, economic losses, and environmental degradation. The analysis of the community's risk perception and coping measures on DRR will provide insights into how residents understand their vulnerabilities and the strategies they employ to mitigate risks. Understanding community perceptions is essential for developing targeted interventions that enhance resilience (Zhai & Lee, 2024). Household surveys are a critical tool in disaster risk management. They gather firsthand information about a community's experiences, vulnerabilities, and capacities, providing essential insights into the local context of disaster risk. These surveys help assess how people perceive different hazards and the strategies they use to cope, allowing for targeted interventions. Additionally, by collecting data on past disasters and current risk management practices, surveys enable evaluation of their effectiveness and pinpoint areas for improvement. This information is invaluable for informing policy and decision-making processes, ensuring that resources are allocated effectively and interventions are appropriate. Furthermore, the involvement of community members in data collection raises awareness about disaster risk, encourages participation in decision-making, and builds local capacity for resilience. In this study, we aim to explore the specific disaster risks present in the Dhorpatan municipality, an area characterized by its unique geographical and socio-economic context. By conducting household surveys, we will gather primary data that will inform our understanding of local vulnerabilities and capacities. This data is crucial for assessing risk perceptions among community members and evaluating existing disaster risk management practices. Ultimately, the study seeks to contribute to the development of evidence-based policies that enhance disaster resilience at the household level. 1.2. OBJECTIVES OF THE STUDY General Objective To study the effect of disaster and risk management practices through household survey Specific Objectives To determine the hazard prevalence in the study area To assess the disaster loss and damage assessment To analyze the community's risk perception and coping measures on DRR 1.3. LIMITATIONS OF THE STUDY Geographical challenges and limited accessibility within the study area may impact the inclusivity of the study. Social and cultural factors might have influenced participant responses and willingness to engage in the survey. 23 The sole reliance on household surveys potentially limited the inclusion of diverse stakeholder perspectives. MATERIALS AND METHODOLOGY 2.1. STUDY AREA The study was conducted within Baglung District, a hilly area in Gandaki Province 275 km west of Kathmandu, Nepal. It has four municipalities and six rural municipalities and a total area of 1784 square kilometers (Panta, 2021). To conduct a comprehensive study on disaster and risk management practices, the following sites within the Baglung district were selected: i) Upstream: Dhorpatan, a municipality in Nepal's Baglung District is a remote region at 3,900 meters elevation in an east-west valley south of the Dhaulagiri mountain range in the Himalayas. ii) Midstream: Bobang, a transitional zone between the downstream and upstream areas is characterized by a mix of agricultural land, forests, and settlements. iii) Downstream: Burtibang is the central hub of Dhorpatan Municipality which is the second- largest commercial center in the district after Baglung. It is a commercial center situated at the lower end of the valley, characterized by a dense population, mixed land use, and a complex network of infrastructure. 24 Figure 7: Map showing the area of household survey sites 2.2. MATERIALS Questionnaire Diary Pen/pencil GPS device Clipboard Ethical Approval Documents 2.3. METHODS 85 random questionnaire surveys were employed to collect primary data from the local population. The selection of three downstream sites (Bazar Area, Shera Dovan, and Churelabas Dovan), one midstream site (Bobang), and one upstream site (Dhorpatan) was done for the study. The questionnaire was designed to gather information on the following aspects: A. Demographic information: Age, gender, occupation, education level, and income level. 25 B. Perception of hazards: Awareness of different hazards, perceived severity, and frequency of occurrence. C. Experience of past disasters: Personal experiences with past disasters, including flood and landslide events. D. Impact of disasters: Assessment of economic, social, and psychological impacts. E. Community preparedness and response: Knowledge of early warning systems, evacuation plans, and emergency response measures. F. Coping mechanisms and resilience: Strategies employed by communities to cope with disasters and build resilience. G. Government and NGO interventions: Perception of government and NGO initiatives in disaster risk reduction and response. Figure 8: Methodological framework of the study 26 RESULTS AND DISCUSSION 3.1. RESULTS 3.1.1 Descriptive Analysis by Demographics 3.1.1.1 Total Respondents The survey included a total of 85 respondents from various locations within the Dhorpatan municipality, with a balanced representation from upstream, midstream, and downstream areas. Figure 9: Total number of respondents in different locations 3.1.1.2 Gender Distribution The gender distribution is nearly balanced, with a slight majority of female respondents (50.6%) compared to male respondents (49.4%).This equal representation indicates that both genders are actively engaged in community discussions and perceptions regarding disaster risk management. 27 Figure 10: Gender distribution of total number of respondents 3.1.1.3 Age group of the respondents The predominant age range among respondents in most locations (Shera Dovan, Bazar Area, Churela Bas Confluence, Gadi Doban, Bobang) is 20-59 years, indicating a strong representation of younger to middle-aged individuals actively participating in the survey. Notably, Bhandari Gau has a significant portion of respondents aged 60 and above, which may reflect the community's demographic composition and the experiences of older individuals with disaster risks. Some areas, such as Nabi Gaau and Selpakhey-Kalimati, include respondents aged up to 60 years, suggesting a slight extension of the typical age range in these locations. Figure 11: Age group of total number of respondents in different sites 28 3.1.1.4 Community Representation by Social Status Across Survey Locations The highest representation of socially advantaged individuals was observed in Shera Dovan (10 respondents) and Nauthanga (10 respondents), indicating these areas have a significant presence in this group. The Nabi Gau area had the highest number of socially marginalized respondents (9), highlighting the challenges faced by this community. Areas like Bazar Area and Bhandari Gau show a mix of both socially advantaged and marginalized communities, with notable numbers in each category, suggesting a diverse social fabric. The Gadi Doban location had the lowest representation of socially advantaged individuals (3) and only one socially marginalized respondent, indicating potential socio-economic challenges. Figure 12: Number of respondents according to their social status 3.1.2 Hazard Prevalence and Disaster Trends The survey data reveals that flooding is the most prevalent hazard in the study area, with 12 respondents ranking it as the most significant threat. Landslides follow closely, with 5 respondents identifying it as the primary concern. Pests and hailstorms were ranked as significant by 3 respondents each. Fire, thunderstorms, and drought were identified as significant by 2 respondents each. There is a noticeable variation in hazard perception across different locations. In upstream areas, flooding and landslides were ranked as the most significant hazards, while in downstream areas, fire and drought were perceived as greater threats. 29 Figure 13: Disaster ranking by respondents across different sites 3.1.3. Disaster Impact and Loss Assessment 3.1.3.1 Record of loss caused by different disasters in different locations The survey results regarding the losses caused by various disasters across different locations in Dhorpatan municipality provide critical insights into the impact of these events on the community. The most significant impact was observed from floods, particularly in Bobang, where there were 60 deaths reported. Other areas, such as Bazar Area and Churela Bas Confluence, also experienced fatalities due to floods, with 3 and 2 deaths, respectively. Notably, several areas reported no losses from floods. Landslides caused minimal impact overall, with only 1 death reported in the Bazar Area. All other locations indicated no losses from landslides. Similar to landslides, thunderstorms resulted in few fatalities, with 2 deaths recorded in the Bazar Area and no other losses reported across the remaining areas. 30 Figure 14: Human Loss due to different types of disasters. 3.1.3.2 Records of damage caused by different disasters in different locations Among the 5 listed disasters, significant property damage was reported in the Bazar Area, while Bhandari Gau experienced property damage along with livestock accidents and reduced production due to flood. Whereas, other areas reported no losses from floods. Damage from landslides was noted in Shera Dovan (property damage) and the Bazar Area (livestock accident). Due to pests, crop damage was reported in Churela Bas Confluence, while other locations indicated no losses. Crop damage and reduced production were reported in Shera Dovan, while other areas like Bhandari Gau and Gadi Doban also experienced crop damage. Agricultural damage was noted in Nauthanga. There were no reported damages from thunderstorms across all surveyed areas. The impact of drought included reduced production and soil quality in Bhandari Gau, with other areas reporting no losses. Issues related to animal attacks and drinking water shortages were noted in specific locations post-disaster events, particularly in Bhandari Gau, which also faced earthquake-related damages. 31 Table 2: Records of damage caused by different disasters in different locations Downstream Upstream Types of Shreea Bazar Churela Bas Bhandari Gau Gadi Doban Nabi Bobang Selpakhey- Nauthang Disaster Dovan Area Confluence Gau Kalimati Flood No Property No Property damage, No No No No loss No damage livestock accident, reduced production Landslide Property Livestock Crop damage No No No No No loss No damage accident Pest Crop damage, No No Crop damage Crop damage No No No loss Agricultural reduced damage production Thunderstorm No No No No No No No No Drought No No No Reduced No No Reduced soil production, quality reduced soil quality Other No No No Animal attack, Water issues No Drinking Damage Problems drinking water for irrigation water caused by and drinking problem earthquake purposes 32 3.1.4. Community Preparedness, Response, and Mitigation Measures for different types of disasters. Table 3: Community Response and Mitigation measures for different types of disasters Type of disaster Response Mitigation Immediate Post-disaster Flood Move uphill or evacuate Food & Temporary shelter Gabion Wall Landslide Escape the risk area Food & Temporary shelter Gabion Wall, plantation In terms of preparedness, a significant number of respondents (65%) reported having evacuation plans in place, demonstrating a proactive approach to disaster risk reduction. However, only a minority of respondents had access to the early warning system through messages on cell phones by government authorities, highlighting a need for improvement in this area. Regarding response measures, 70% of respondents indicated that they would seek help from local authorities in case of a disaster. This suggests a reliance on government agencies for emergency response. However, only 30% of respondents reported having first-aid training, indicating a potential gap in community-level response capabilities. Only 20% of respondents had access to insurance coverage, indicating a potential vulnerability to economic losses from disasters. 3.2 DISCUSSION The survey revealed that floods were the most devastating disaster type in Dhorpatan, particularly affecting Bobang with 60 reported deaths. This aligns with findings from other studies in Nepal, where floods frequently cause significant fatalities and property damage due to the country's topography and monsoon climate. For instance, a study by Jamshedet al. (2019) emphasizes that communities in hilly regions of Nepal are particularly vulnerable to flooding due to inadequate infrastructure and poor land management practices. Similarly, research in Bangladesh indicates that flood-related fatalities often arise from a lack of preparedness and early warning systems (Dhar et al., 2023). In contrast, landslides caused minimal impact overall in Dhorpatan, with only one death reported in the Bazar Area. This contrasts with findings from regions like Sindhupalchowk, where landslides have been linked to significant loss of life and property due to steep terrain and heavy rainfall (United Nations University, 2019). The differences in impact may be attributed to varying geological conditions and community preparedness levels across different regions. The survey indicated that while 65% of respondents had evacuation plans, only 30% reported having first-aid training.This highlights 33 a gap in community response capabilities, which is consistent with findings from a study conducted in rural India where similar deficiencies were noted (Asih et al., 2023). Effective DRM requires not only planning but also training community members to respond effectively during disasters. As noted by Asih et al. (2023), effective DRM requires active participation from community members in data collection and decision-making processes. Our research emphasizes this by involving residents in surveys, thereby raising awareness about disaster risks and fostering a sense of ownership over DRM strategies. Moreover, the reliance on local authorities for emergency response was evident, with 70% of respondents indicating they would seek help from government agencies. This dependence underscores the need for strengthening local governance structures and enhancing community engagement in DRM processes. Research by Feng and Cui(2021) suggests that empowering local communities through training and resources can significantly improve disaster resilience. The survey results also highlighted disparities in social status among respondents, with socially marginalized communities facing greater challenges during disasters. This finding is echoed in broader research indicating that marginalized groups often experience higher vulnerability due to limited resources and access to information (Flanagan et al., 2011). For example, a study conducted in rural Nepal found that marginalized communities were disproportionately affected by disasters due to their socio-economic conditions, which hinder their ability to recover (Cutter et al., 2003). Moreover, research conducted by Dhar et al. (2023) illustrates that marginalized communities often experience heightened vulnerability due to limited resources and access to information. This is reflected in our survey results, which indicate that socially marginalized groups in Dhorpatan face greater challenges during disasters. Such findings underscore the necessity for targeted interventions that address the specific needs of these communities. The findings from the Dhorpatanmunicipality survey reflect broader trends observed in disaster risk management across Nepal and South Asia. While there are similarities in the types of disasters faced and the impacts experienced, variations exist based on geographical conditions, community preparedness, and social structures. To enhance resilience against future disasters, policymakers must focus on improving infrastructure, increasing community training programs, and ensuring equitable access to resources for all social groups. By addressing these issues through targeted interventions, communities can better prepare for and respond to disasters, ultimately reducing loss of life and property. 34 CONCLUSION AND RECOMMENDATIONS 4.1 CONCLUSION This study underscores the critical importance of understanding local contexts, community perceptions, and existing capacities in developing effective disaster risk management (DRM) strategies. The findings highlight the need for context-specific interventions that address the unique challenges and vulnerabilities faced by different communities within the Dhorpatan municipality. While floods emerged as the most significant hazard, the impact of other disasters like landslides and droughts varied based on location and socio-economic factors. The study emphasizes the importance of strengthening community-level preparedness and response capacities, including enhancing early warning systems, first aid training, and access to insurance coverage. Additionally, it underscores the need to address the specific needs of marginalized communities, who often face greater challenges during disasters due to limited resources and social inequities. 4.2 RECOMMENDATIONS There should be an investment in community-based disaster preparedness, first aid, and emergency response There should be improvement in the reach and effectiveness of early warning systems, particularly in remote and vulnerable areas Targeted interventions that address the specific needs and vulnerabilities of marginalized communities should be developed There should be investment in Risk-reduction infrastructures to mitigate the impacts of disasters By implementing these recommendations, the Dhorpatan municipality can enhance its resilience to disasters and minimize the loss of life and property. 35 REFERENCES Alexander, D. (2018). New perspectives on natural disasters. Oxford University Press. Asih, S. W., Pandin, M. G. R., Yusuf, A., & Supriyadi. (2023). Literature Review: Disaster Risk Reduction Programs To Increase Public Awareness Of Natural Disasters. https://doi.org/10.1101/2023.12.15.23300051 Burton, I. (1993). The environment is hazard. The Guilford Press. Burton, I., Kates, R., & White, G. (1978). The environment is hazard. Oxford University Press. Cutter, S. L., Boruff, B. J., & Shirley, W. L. (2003). Social Vulnerability to Environmental Hazards *. Social Science Quarterly, 84(2), 242–261. https://doi.org/10.1111/1540- 6237.8402002 Dahal, B. K., & Dahal, R. K. (2017). Landslide hazard map: Tool for optimization of low-cost mitigation. Geoenvironmental Disasters, 4(1), 8. https://doi.org/10.1186/s40677-017-0071- 3 Deoja, B., Dhital, M., Thapa, B., & Wagner, A. (1991). Mountain risk engineering-Part I (pp. 188–192). International Center for Integrated Mountain Development. Dhar, T., Bornstein, L., Lizarralde, G., & Nazimuddin, S. M. (2023). Risk perception—A lens for understanding adaptive behaviour in the age of climate change? Narratives from the Global South. International Journal of Disaster Risk Reduction, 95, 103886. https://doi.org/10.1016/j.ijdrr.2023.103886 Donahue, D., Barach, P., Swienton, R. E., Hansen, J.-C., & Harris, C. (2023). Creating a Health Emergency and Disaster Risk Management (EDRM) Learning Community. Prehospital and Disaster Medicine, 38, s159–s159. Du, Y., Ding, Y., Li, Z., & Cao, G. (2015). The role of hazard vulnerability assessments in disaster preparedness and prevention in China. Military Medical Research, 2, 1-7. Feng, Y., & Cui, S. (2021). A review of emergency response in disasters: Present and future perspectives. Natural Hazards, 105(1), 1109–1138. https://doi.org/10.1007/s11069-020- 04297-x Flanagan, B. E., Gregory, E. W., Hallisey, E. J., Heitgerd, J. L., & Lewis, B. (2011). A Social Vulnerability Index for Disaster Management. Journal of Homeland Security and Emergency Management, 8(1), 0000102202154773551792. https://doi.org/doi:10.2202/1547-7355.1792 Ghimire, B., & Shakya, S. R. (2019). Water Resource Management in Nepal: Challenges and Opportunities. Nepal Water Conservation Journal. 36 Glastonbury, J., & Fell, R. (2008). Geotechnical characteristics of large slow, very slow, and extremely slow landslides. Canadian Geotechnical Journal, 45(7), 984–1005. Holec, J., Bednarik, M., Šabo, M., Minár, J., Yilmaz, I., & Marschalko, M. (2013). A small- scale landslide susceptibility assessment for the territory of Western Carpathians. Natural Hazards, 69, 1081–1107. Howell, J. (1999). Roadside bioengineering reference manual (pp. 81–102). Department of Roads, Government of Nepal. Jamshed, A., Rana, I. A., Mirza, U. M., & Birkmann, J. (2019). Assessing the relationship between vulnerability and capacity: An empirical study on rural flooding in Pakistan. International Journal of Disaster Risk Reduction, 36, 101109. https://doi.org/10.1016/j.ijdrr.2019.101109 Joshi, K. N., 1987. Satellite Remote Sensing and Its Application in Flood Hazard- A Case Study in Transitional Zone (Luni Basin) of Great India. Kayona, R., Clarke, M., Murray, V., Chan, E. Y. Y., Abrahams, J., & O’Sullivan, T. L. (2023). WHO Guidance on Research Methods for Health Emergency and Disaster Risk Management. Prehospital and Disaster Medicine, 38, s25–s25. Khadka, N., Chen, X., Shrestha, M., & Liu, W. (2024). Risk perception and vulnerability of communities in Nepal to transboundary glacial lake outburst floods from Tibet, China. International Journal of Disaster Risk Reduction, 107, 104476. https://doi.org/10.1016/j.ijdrr.2024.104476 Khanal, N. R., Shrestha, M., & Ghimire, M. (2007). Preparing for flood disaster: mapping and assessing hazard in the Ratu Watershed, Nepal. International Centre for Integrated Mountain Development (ICIMOD). Mahato, R. C., D. Higaki, T. B. Thapa and N. P. Paudyal, 1996. Hazard Mapping Based on the Few Case Studies in the Central Region of Nepal. Proceedings of An International Seminar on Water Induced Disaster, Kathmandu, Nepal 26-29 November, pg 260. Othman, A., El-Saoud, W. A., Habeebullah, T., Shaaban, F., & Abotalib, A. Z. (2023). Risk assessment of flash flood and soil erosion impacts on electrical infrastructures in overcrowded mountainous urban areas under climate change. Reliability Engineering & System Safety, 236. Panta, S. R. (2021). Gandharva and their livelihood in Baglung District, Nepal. Dhaulagiri Journal of Sociology and Anthropology, 15(01), 95-100. 37 Poudel, K., & Regmi, A.D. (2016). Landslide susceptibility mapping along Tulsipur-Kapurkot road section and its surrounding region using a bivariate statistical model. Journal of Nepal Geological Society, 50(1), 83-93. https://doi.org/10.3126/jngs.v Poudel, K., Gurung, H., & Lama, P. (2018). Flow Dynamics and River Discharge in the Himalayas. Himalayan Journal of Water Sciences, 7(2), 89-102. Shrestha, B., Gurung, P., & Dhakal, S. (2014). Hydrological Analysis of Nepal's River Basins. Journal of Hydrology Shrestha, N., & Bajracharya, S. R. (2015). Hydrology and Discharge Patterns in Nepalese Rivers. Kathmandu University Press United Nations University. (2019). Disaster risk reduction in Nepal: A comprehensive assessment of disaster risk management practices. Retrieved from https://collections.unu.edu/eserv/unu:5854/CSR_Nepal_Final.pdf Varnes, D. J. (1984). Landslide hazard zonation: A review of principles and practice. UNESCO. Wentworth's, C. K. (n.d.). A scale of grade and class terms for clastic sediments. The Journal of Geology, 377-392. Zhai, L., & Lee, J. E. (2024). Exploring and Enhancing Community Disaster Resilience: Perspectives from Different Types of Communities. Water, 16(6), 881. https://doi.org/10.3390/w16060881 38 ANNEXES 1. Annex 1: GPS of study sites Table 4: Upstream and Downstream Location coordinates Site Location Latitude Longitude Downstream I Jaljala 28.26658333 83.30416667 II Bhakunde 28.22877778 83.61808333 III Burtibang 28.334539 83.159898 IV Burtibang 28.337195 83.161515 V Burtibang 28.337048 83.162388 Upstream VI Bobang 28.490551 83.05464 VII Bobang 28.488205 83.056684 VIII Dhorpatan 28.67667222 83.07959 2. Annex 2: Data Calculation Table 5: Flow velocity and discharge rate calculation Distance Length Breadth Area A Time Velocity V Discharge( S.n. Site Travelled (m) (m) (m²) taken (s) (m/s) Q) (m³/s) (m) 1 Bhuji Khola 14 1.67 23.38 10.36 10 1.036 24.22 2 Tamag Khola 13 1.83 23.79 8.95 10 0.895 21.29 3 Bobang river 6 1 6 13.4 10 1.34 8.04 4 Uttarganga river 15 0.98 14.7 6.33 10 0.633 9.31 39 Table 6: Calculation of Boulder size and volume length breadth Volume Average max Length SITE SN height (cm) (cm) (cm) (cm³) Volume (cm³) length (mm) 1 10 7 4 280 2 10 8.2 0.5 41 I 3 18 11 6.2 1227.6 368.92 18 180 4 6 4 3 72 5 8 7 4 224 1 11 4 5 220 2 18 11 3 594 3 26 6 6 936 II 4 13 10 9 1170 1022.86 26 260 5 21 17 10 3570 6 11 4 4 176 7 19 13 2 494 1 9 4 2 72 2 17 13 6 1326 3 10 7 6 420 III 578.77 18 180 4 18 10 5 900 5 14 10 5 700 6 9.1 6 1 54.6 Table 7: Sites along with the average length of boulders measured SITE LENGTH (mm) I 180 II 260 III 180 40 PHOTOGRAPHS Figure 15: Group photo downstream at Figure 16: Group photo upstream at Burtibang Dhorpatan Figure 17: Measurement of Boulder volume Figure 18: Landslide viewing 41 Figure 19: Survey with local community Figure 20: Flow measurement using Current members meter 42