Principles Of Development Summary PDF
Document Details
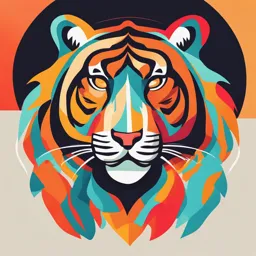
Uploaded by DynamicFresno7722
University of Ibadan
Tags
Summary
This document summarizes the principles of development, covering developmental anatomy, life cycles, and developmental genetics. It discusses the function of organs during development, how eggs become adults, and the role of genetics in development, including topics such as differentiation, morphogenesis, growth, reproduction, and evolution. It also includes principles from various researchers like Karl von Baer and Charles Darwin.
Full Transcript
Principles of Development: Developmental Anatomy 1. Organisms must function as they form their organs. They have to use one set of structures while constructing others. 2. The main question of development is, How does the egg becomes an adult? This question can be broken down into the component pr...
Principles of Development: Developmental Anatomy 1. Organisms must function as they form their organs. They have to use one set of structures while constructing others. 2. The main question of development is, How does the egg becomes an adult? This question can be broken down into the component problems of differentiation (How do cells become different from one another and from their precursors?), morphogenesis (How is ordered form is generated?), growth (How is size regulated?), reproduction (How does one generation create another generation?), and evolution (How do changes in developmental processes create new anatomical structures?). 3. Epigenesis happens. New organisms are created de novo each generation from the relatively disordered cytoplasm of the egg. 4. Preformation is not in the anatomical structures, but in the instructions to form them. The inheritance of the fertilized egg includes the genetic potentials of the organism. 5. The preformed nuclear instructions include the ability to respond to environmental stimuli in specific ways. 6. The ectoderm gives rise to the epidermis, nervous system, and pigment cells. 7. The mesoderm generates the kidneys, gonads, bones, heart, and blood cells. 8. The endoderm forms the lining of the digestive tube and the respiratory system. 9. Karl von Baer's principles state that the general features of a large group of animals appear earlier in the embryo than do the specialized features of a smaller group. As each embryo of a given species develops, it diverges from the adult forms of other species. The early embryo of a "higher" animal species is not like the adult of a "lower" animal. 10. Labeling cells with dyes shows that some cells differentiate where they form, while others migrate from their original sites and differentiate in their new locations. Migratory cells include neural crest cells and the precursors of germ cells and blood cells. 11. "Community of embryonic structure reveals community of descent" (Charles Darwin). 12. Homologous structures in different species are those organs whose similarity is due to their sharing a common ancestral structure. Analogous structures are those organs whose similarity comes from their serving a similar function (but which are not derived from a common ancestral structure). 13. Congenital anomalies can be caused by genetic factors (mutations, aneuploidies, translocations) or by environmental agents (certain chemicals, certain viruses, radiation). 14. Syndromes consists of sets of developmental abnormalities that "run together." 15. Organs that are linked in developmental syndromes share either a common origin or a common mechanism of formation. 16. If growth is isometric, a twofold change in weight will cause a 1.26-fold expansion in length. 17. Allometric growth can create dramatic changes in the structure of organisms. 18. Complex patterns may be self-generated by reaction-diffusion events, wherein the activator of a local phenomenon stimulates the production of more of itself as well as the production of a more diffusible inhibitor. Principles of Development: Life Cycles and Developmental Patterns 1. The life cycle can be considered a central unit in biology. The adult form need not be paramount. In a sense, the life cycle is the organism. 2. The basic life cycle consists of fertilization, cleavage, gastrulation, germ layer formation, organogenesis, metamorphosis, adulthood, and senescence. 3. Reproduction need not be sexual. Some organisms, such as Volvox and Dictyostelium, exhibit both asexual reproduction and sexual reproduction. 4. Cleavage divides the zygote into numerous cells called blastomeres. 5. In animal development, gastrulation rearranges the blastomeres and forms the three germ layers. 6. Organogenesis often involves interactions between germ layers to produce distinct organs. 7. Germ cells are the precursors of the gametes. Gametogenesis forms the sperm and the eggs. 8. There are three main ways to provide nutrition to the developing embryo: (1) supply the embryo with yolk; (2) form a larval feeding stage between the embryo and the adult; or (3) create a placenta between the mother and the embryo. 9. Life cycles must be adapted to the nonliving environment and interwoven with other life cycle 10. Don't regress your tail until you've formed your hindlimbs. 11. There are several types of evidence. Correlation between phenomenon A and phenomenon B does not imply that A causes B or that B causes A. Loss-of-function data (if A is experimentally removed, B does not occur) suggests that A causes B, but other explanations are possible. Gain- of-function data (if A happens where or when it does not usually occur, then B also happens in this new time or place) is most convincing. 12. Protostomes and deuterostomes represent two different sets of variations on development. Protostomes form the mouth first, while deuterostomes form the anus first. There are five major questions for embryologists who study morphogenesis: 1. How are tissues formed from populations of cells? For example, how do neural retina cells stick to other neural retina cells and not become integrated into the pigmented retina or iris cells next to them? How are the various cell types within the retina (the three distinct layers of photoreceptors, bipolar neurons, and ganglion cells) arranged so that the retina is functional? 2. How are organs constructed from tissues? The retina of the eye forms at a precise distance behind the cornea and the lens. The retina would be useless if it developed behind a bone or in the middle of the kidney. Moreover, neurons from the retina must enter the brain to innervate the regions of the brain cortex that analyze visual information. All these connections must be precisely ordered. 3. How do organs form in particular locations, and how do migrating cells reach their destinations? Eyes develop only in the head and nowhere else. What stops an eye from forming in some other area of the body? Some cells for instance, the precursors of our pigment cells, germ cells, and blood cells must travel long distances to reach their final destinations. How are cells instructed to travel along certain routes in our embryonic bodies, and how are they told to stop once they have reached their appropriate destinations? 4. How do organs and their cells grow, and how is their growth coordinated throughout development? The cells of all the tissues in the eye must grow in a coordinated fashion if one is to see. Some cells, including most neurons, do not divide after birth. In contrast, the intestine is constantly shedding cells, and new intestinal cells are regenerated each day. The mitotic rate of this tissue must be carefully regulated. If the intestine generated more cells than it sloughed off, it could produce tumorous outgrowths. If it produced fewer cells than it sloughed off, it would soon become nonfunctional. What controls the rate of mitosis in the intestine? 5. How do organs achieve polarity? If one were to look at a cross section of the fingers, one would see a certain organized collection of tissues bone, cartilage, muscle, fat, dermis, epidermis, blood, and neurons. Looking at a cross section of the forearm, one would find the same collection of tissues. But they are arranged very differently in different parts of the arm. How is it that the same cell types can be arranged in different ways in different parts of the same structure? All these questions concern aspects of cell behavior. There are two major types of cell arrangements in the embryo: epithelial cells, which are tightly connected to one another in sheets or tubes, and mesenchymal cells, which are unconnected to one another and which operate as independent units. Morphogenesis is brought about through a limited repertoire of variations in cellular processes within these two types of arrangements: (1) the direction and number of cell divisions; (2) cell shape changes; (3) cell movement; (4) cell growth; (5) cell death; and (6) changes in the composition of the cell membrane or secreted products. We will discuss the last of these considerations here. Principles of Development: Experimental Embryology 1. There are norms of reaction that describe an embryo's inherited ability to develop a range of phenotypes. The environment can play a role in selecting which phenotype is expressed. (Examples include temperature-dependent sex determination and seasonal phenotypic changes in caterpillars and butterflies.) 2. Developing organisms are adapted to the ecological niches in which they develop. (Examples include the ability of frog eggs exposed to sunlight to repair DNA damage.) 3. Before cells overtly differentiate into the many cell types of the body, they undergo a "covert" commitment to a certain fate. This commitment is first labile (the specification step) but later becomes irreversible (the determination step). 4. In autonomous specification, removal of a blastomere from an embryo causes the absence in the embryo of those tissues formed by that blastomere. This mechanism of specification produces a mosaic pattern of development. (Examples include early snail and tunicate embryos.) 5. In autonomous specification, morphogenetic determinants are apportioned to different blastomeres during cell cleavage. (An example is the yellow crescent cytoplasm that is found in the muscle- forming cells of tunicate embryos.) 6. In conditional specification, the removal of a blastomere from the embryo can be compensated for by the other cells' changing their fates. Each cell can potentially give rise to more cell types than it normally does. This produces a regulative pattern of development wherein cell fates are determined relatively late. (Examples include frog and mammalian embryos.) 7. In conditional specification, the fate of a cell often depends upon its neighbors ("whom it meets"). 8. In conditional specification, groups of cells can have their fates determined according to a concentration gradient of morphogen. The cells specified by such a morphogen can constitute a field. 9. In syncytial specification, the fates of cells can be determined by gradients of morphogens within the egg cytoplasm. 10. Different cell types can sort themselves into regions by means of cell surface molecules such as cadherins. These molecules can be critical in patterning cells into tissues and organs. Principles of Development: Genes and Development 1. Development connects genotype and phenotype. 2. Nuclear genes are not lost or mutated during development. The genome of every cell is equivalent. 3. The exceptions to the rule of genomic equivalence are the lymphocytes. During differentiation, these cells rearrange their DNA to create new immunoglobulin and antigen receptor genes. 4. Genomic equivalence is implied by metaplasia, in which one differentiated cell type becomes another differentiated cell type. An example is the transdifferentiation of the salamander dorsal iris into a lens when the lens is removed. 5. The ability of nuclei from differentiated cells to direct the development of complete adult organisms has recently confirmed the principle of genomic equivalence. 6. The cloning of human beings, as well as regenerating damaged organs or enhancing physical abilities, may soon be possible through cloning technology and the use of embryonic stem cells. 7. Only a small percentage of the genome is expressed in any particular cell. 8. Polytene chromosomes, in which the DNA has replicated without separating (as in larval Drosophila salivary glands), show regions where DNA is being transcribed. Different cell types show different regions of DNA being transcribed. 9. Northern blots, in situ hybridization, and the polymerase chain reaction can show which cells are transcribing particular genes. 10. The functions of a gene often can be ascertained by antisense mRNA, transgenic expression, or (in the case of mammals) gene knockouts. 11. Knowledge of gene activity in humans can be obtained by candidate gene mapping or positional cloning Principles of Development: Developmental Genetics 1. Differential gene expression from genetically identical nuclei creates different cell types. Differential gene expression can occur at the levels of gene transcription, nuclear RNA processing, mRNA translation, and protein modification. 2. Genes are usually repressed. Activation of a gene often means inhibiting its repressor. This leads to thinking in double and triple negatives: Activation is often the inhibition of the inhibitor; repression is the inhibition of the inhibitor of the inhibitor. 3. Eukaryotic genes contain promoter sequences to which RNA polymerase can bind to initiate transcription. The eukaryotic RNA polymerases are bound by a series of proteins called basal transcription factors. 4. Eukaryotic genes expressed in specific cell types contain enhancer sequences that regulate their transcription in time and space. 5. Specific transcription factors can recognize specific sequences of DNA in the promoter and enhancer regions. They activate or repress transcription from the genes to which they have bound. 6. Enhancers work in a combinatorial fashion. The binding of several transcription factors can act to promote or inhibit transcription from a certain promoter. In some cases transcription is activated only if both factor A and factor B are present, while in other cases, transcription is activated if either factor A or factor B is present. 7. A gene encoding a transcription factor can keep itself activated if the transcription factor it encodes also activates its own promoter. Thus, a transcription factor gene can have one set of enhancer sites to initiate its activation and a second set of enhancer sites (that bind the encoded transcription factor) to maintain its activation. 8. Often, the same transcription factors that are used during the differentiation of a particular cell type are also used to activate the genes for that cell type's specific products. For instance, Pax6 is needed both for the differentiation of the lens and for the transcription of the lens crystallin genes, and Mitf is needed for pigment cell differentiation and for the transcription of the genes whose products catalyze the synthesis of melanin. 9. Enhancers can act as silencers to suppress the transcription of a gene in inappropriate cell types. 10. Locus control regions may function by making relatively large portions of a chromosome accessible to transcription factors. 11. Transcription factors act in different ways to regulate RNA synthesis. Some transcription factors stabilize RNA polymerase binding to the DNA, some disrupt nucleosomes, and some increase the efficiency of transcription. 12. Transcription correlates with a lack of methylation on the promoter and enhancer regions of genes. Methylation differences can account for examples of genomic imprinting, wherein a gene transmitted through the sperm is expressed differently from the same gene transmitted through the egg. 13. Dosage compensation enables the X chromosome-derived products of males (which have one X chromosome per cell in fruit flies and mammals) to equal the X chromosome-derived products of females (which have two X chromosomes per cell). This compensation is accomplished at the level of transcription, either by accelerating transcription from the lone X chromosome in males (Drosophila) or by inactivating a large portion of one of the two X chromosomes in females (mammals). 14. X chromosome inactivation in placental mammals is generally random and involves the activation of the Xist gene on the chromosome that will be inactivated. 15. Differential RNA selection can allow certain transcripts to enter the cytoplasm while preventing other transcripts from leaving the nucleus. 16. Differential RNA splicing can create a family of related proteins by causing different regions of the nRNA to be read as exons and introns. What is an exon in one set of circumstances may be an intron in another. 17. Some messages are translated only at certain times. The oocyte, in particular, uses translational regulation to set aside certain messages that it transcribes during egg development but uses only after the egg is fertilized. This activation is often accomplished either by the removal of inhibitory proteins or by the polyadenylation of the message. 18. Many messenger RNAs are localized to particular regions of the oocyte or other cells. This localization appears to be regulated by the 3 ́ untranslated region of the mRNA.